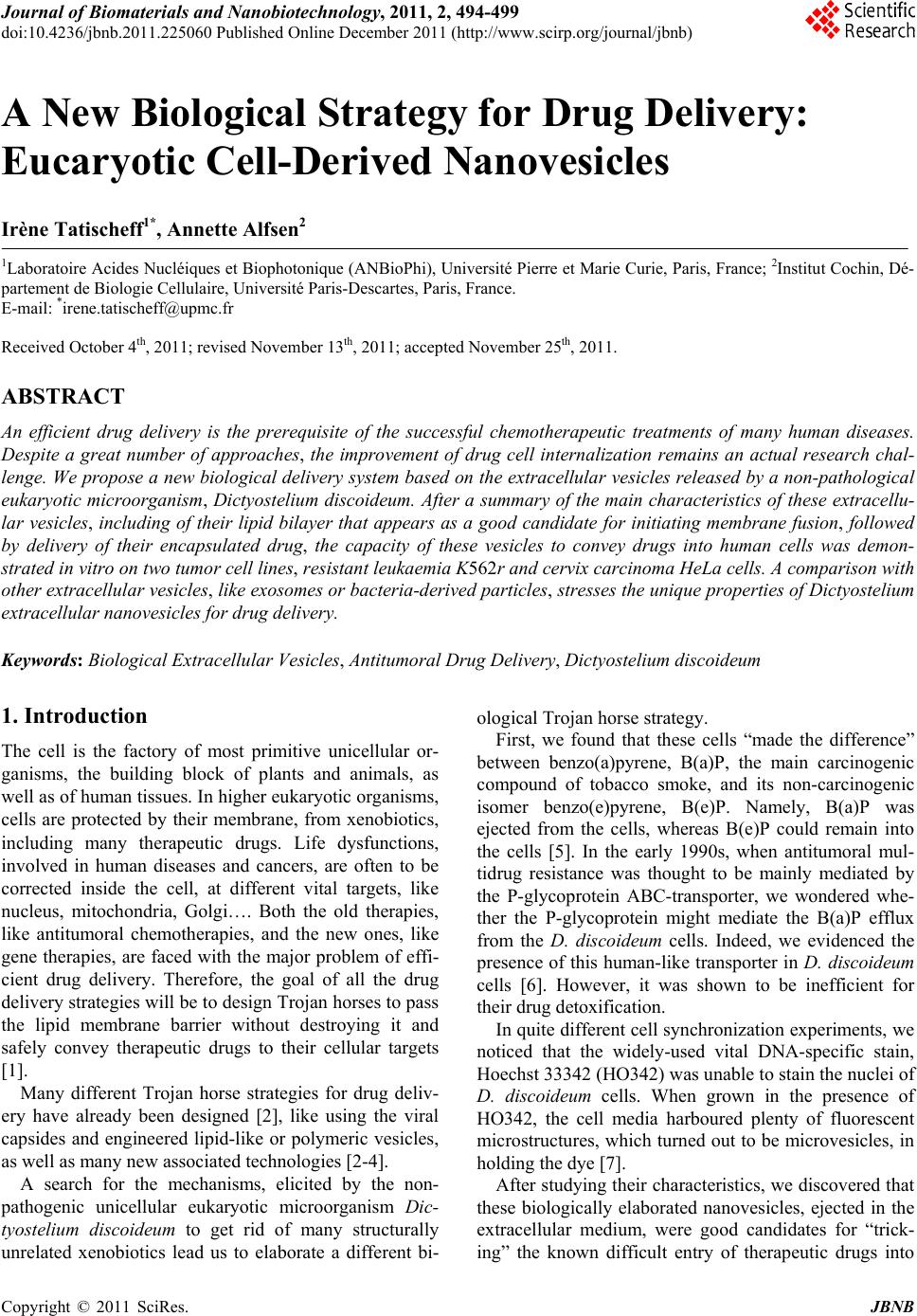 Journal of Biomaterials and Nanobiotechnology, 2011, 2, 494-499 doi:10.4236/jbnb.2011.225060 Published Online December 2011 (http://www.scirp.org/journal/jbnb) Copyright © 2011 SciRes. JBNB A New Biological Strategy for Drug Delivery: Eucaryotic Cell-Derived Nanovesicles Irène Tatischeff1*, Annette Alfsen2 1Laboratoire Acides Nucléiques et Biophotonique (ANBioPhi), Université Pierre et Marie Curie, Paris, France; 2Institut Cochin, Dé- partement de Biologie Cellulaire, Université Paris-Descartes, Paris, France. E-mail: *irene.tatischeff@upmc.fr Received October 4th, 2011; revised November 13th, 2011; accepted November 25th, 2011. ABSTRACT An efficient drug delivery is the prerequisite of the successful chemotherapeutic treatments of many human diseases. Despite a great number of approaches, the improvement of drug cell internalization remains an actual research chal- lenge. We propose a new biological delivery system based on the extracellular vesicles released by a non-pathological eukaryotic microorganism, Dictyostelium discoideum. After a summary of the main characteristics of these extracellu- lar vesicles, including of their lipid bilayer that appears as a good candidate for initiating membrane fusion, followed by delivery of their encapsulated drug, the capacity of these vesicles to convey drugs into human cells was demon- strated in vitro on two tumor cell lines, resistant leukaemia K562r and cervix carcinoma HeLa cells. A comparison with other extracellular vesicles, like exosomes or bacteria-derived particles, stresses the unique properties of Dictyostelium extracellular nanovesicles for drug delivery. Keywords: Biological Extracellular Vesicles, Antitumoral Drug Delivery, Dict yo st elium discoid eu m 1. Introduction The cell is the factory of most primitive unicellular or- ganisms, the building block of plants and animals, as well as of human tissues. In higher eukaryotic organisms, cells are protected by their membrane, from xenobiotics, including many therapeutic drugs. Life dysfunctions, involved in human diseases and cancers, are often to be corrected inside the cell, at different vital targets, like nucleus, mitochondria, Golgi…. Both the old therapies, like antitumoral chemotherapies, and the new ones, like gene therapies, are faced with the major problem of effi- cient drug delivery. Therefore, the goal of all the drug delivery strategies will be to design Trojan horses to pass the lipid membrane barrier without destroying it and safely convey therapeutic drugs to their cellular targets [1]. Many different Trojan horse strategies for drug deliv- ery have already been designed [2], like using the viral capsides and engineered lipid-like or polymeric vesicles, as well as many new associated technologies [2-4]. A search for the mechanisms, elicited by the non- pathogenic unicellular eukaryotic microorganism Dic- tyostelium discoideum to get rid of many structurally unrelated xenobiotics lead us to elaborate a different bi- ological Trojan horse strategy. First, we found that these cells “made the difference” between benzo(a)pyrene, B(a)P, the main carcinogenic compound of tobacco smoke, and its non-carcinogenic isomer benzo(e)pyrene, B(e)P. Namely, B(a)P was ejected from the cells, whereas B(e)P could remain into the cells [5]. In the early 1990s, when antitumoral mul- tidrug resistance was thought to be mainly mediated by the P-glycoprotein ABC-transporter, we wondered whe- ther the P-glycoprotein might mediate the B(a)P efflux from the D. discoideum cells. Indeed, we evidenced the presence of this human-like transporter in D. discoideum cells [6]. However, it was shown to be inefficient for their drug detoxification. In quite different cell synchronization experiments, we noticed that the widely-used vital DNA-specific stain, Hoechst 33342 (HO342) was unable to stain the nuclei of D. discoideum cells. When grown in the presence of HO342, the cell media harboured plenty of fluorescent microstructures, which turned out to be microvesicles, in holding the dye [7]. After studying their characteristics, we discovered that these biologically elaborated nanovesicles, ejected in the extracellular medium, were good candidates for “trick- ing” the known difficult entry of therapeutic drugs into
 A New Biological Strategy for Drug Delivery: Eucaryotic Cell-Derived Nanovesicle495 human cells. Using HO342, we have shown that the iso- lated dye-containing vesicles were able to deliver it into the nuclei of naive Dictyostelium cells, thus overcoming their constitutive resistance to the free dye. Moreover, with living human leukemia multidrug resistant cells, K562r, known to be resistant to HO342, a Dictyostelium vesicle-mediated dye-transfer into the nuclei was also evidenced [8]. Control experiments showed that Dic- tyostelium vesicle-release was not a mere detoxification mechanism, but was both constitutive (Tatischeff, et al., 1998) and important for intercellular communication. With the goal of using these biological extracellular nanovesicles for drug delivery, an in vitro investigation was conducted on HeLa cells, with hypericin, a hydro- phobic fluorescent photosensitizer, aimed for antitumoral photodynamic therapy. Thus, we found that Dictyos- telium nanovesicles, biologically loaded with this thera- peutic molecule, are a promising nanodevice for cellular drug delivery [9]. In the present paper, we intend to further propose the use of D. discoideum extracellular nanovesicles for drug delivery1. We summarize their main characteristics, and stress their efficiency for drug delivery into tumoral hu- man cells, as shown by our in vitro experiments. The advantages upon the use of these nanovesicles, when compared with other cell-derived microvesicles are dis- cussed. 2. Materials and Methods All the materials and methods used in our work on Dic- tyostelium nanovesicles have been detailed in our previ- ous papers [7-9]. Only the methods dealing with Dic- tyostelium cell cultures and preparation of vesicles will be recalled here for clarity. 2.1. Cell Culture Dictyostelium cells, Ax-2 strain, were grown in suspen- sion in the dark, on a gyratory shaker (150 rpm) at +22˚C, in HL5 semi-defined medium [10], containing penicillin (50 U/mL) and streptomycin (50 U/mL) (Biomedia, Bou- ssens, France). In contrast with mammalian cells, Dic- tyostelium cells are grown without fetal calf serum. For proper oxygenation, each suspension was grown in an Erlenmeyer containing five times the suspension volume. 2.2. Preparation of Dictyostelium Nanovesicles Dictyostelium cell cultures, were initiated at 5 × 105 - 106 cells·ml−1 and grown in suspension in HL5 medium in the absence (control) or in the presence of the drug to be vectorized. For preparation of nanovesicles, cells were generally used in the late exponential or early stationary phase of growth, at a cell density about 107 cells·ml−1. One has to check that the drug does not affect cell growth. After 48 h of growth, both cell suspensions were centri- fuged at 700 x g for 5 min (+20˚C). The cell-free media were centrifuged at 2000 x g for 10 min (+20˚C). The 2000 x g supernatants were centrifuged at 12,000 x g for 30 min (+4˚C). Nanovesicles present in the pellets were concentrated (×100) in phosphate buffered saline (PBS), pH 7.2 without calcium and magnesium (GIBCO). These nanovesicles were quite stable in PBS, as they did withstand repeated liquid nitrogen freeze-thaw cycles without breaking. As observed with nanovesicles in holding HO342, they could be kept at +4˚C at least 2 months without releasing the dye. 3. Main Results In the Eukarya branch of the evolutionary tree, Dictyos- telium discoideum (http://www.dictybase.org), an ances- tral non-pathogenic amoeba, placed at the border be- tween the plant and animal kingdoms, is equipped with a lipid membrane that plays a critical role in many aspects of cell development. As in other eukaryotic cells, Dic- tyostelium plasma membrane contains proteins, lipids and carbohydrates. The phospholipid content of the plasma membrane of axenically grown vegetative Ax-2 cells is not very different from the one of mammalian cell membranes, except for an extremely high amount of unsaturated fatty acids, making up 75% - 90% of the fatty acids of the organism and of the membrane, respec- tively. The membrane sterol is stigmasterol, instead of the mammalian cholesterol. 3.1. Characteristics of Dictyostelium Nanovesicles Morphological analysis of control vesicles prepared from the cell growth medium was carried out by cryoelectron microscopy imaging. The vesicles appeared mostly smooth and rounded, delineated by a lipid membrane bilayer (Figure 1). The histogram of their heterogeneous size distribution indicated that almost 80% of them have an average diameter within a range of 50 - 150 nm [9]. CdSe/CdZnS quantum dots phospholipid micelles were used, to check whether Dictyostelium cells might be de- toxified from these quantum dots by following the vesi- cle-mediated pathway. As shown on Figure 1, contrary to many xenobiotics, these quantum dots were excreted from Dictyostelium cells, as aggregates outside the vesi- cles. This observation indicates that all the foreign com- pounds, incubated with Dictyostelium cells, are not deto- 1Tatischeff, I., Alfsen, A., Lavialle, F. “Extracellular vesicles from non-pathogenic amoebae useful as vehicle for transferring a molecule of interest to an eukaryotic cell” Patent european priority N 03 291 752 07/15/2003 (DRITT-UPMC) (European Patents in Danemark, Deutschland, France, Great Britain, Italy, Netherland and Spain), US Patent and Pending Canadian Patent). Copyright © 2011 SciRes. JBNB
 A New Biological Strategy for Drug Delivery: Eucaryotic Cell-Derived Nanovesicle 496 Figure 1. Morphological characterization of Dictyostelium nanovesicles by cryoelectron microscopy. For methodologi- cal details, cf. [9]. As shown on the figure, CdSe/CdZnS quantum dots phospholipid micelles, incubated with grow- ing Dictyostelium cells, were excreted as (black) aggregates outside the nanovesicles. xified by means of the extravesicular pathway. Further on- going studies should help understanding the structural cha- racteristics required for a drug vesicle-mediated transport. A proteomic analysis of the control vesicles revealed a predominance of actin and actin-related proteins. The detection of a lysosomal membrane protein (LIMP II) [9] indicated that these vesicles are likely generated in the late endosomal compartment, as the known exosomes of many mammalian cells. Lipid analysis of the bilayer surrounding the vesicles [7] showed, beside the phospholipids and lipids common in the plasma membrane: PC, PI, PS and PE, sphingo- myelin, PG and DPG, the presence of lyso bis-phos- phatidic acid (LBPA), a lipid inducing membrane fusion [11]. Noteworthy, this unusual phospholipid is a major membrane constituent of the internal vesicles of multive- sicular bodies [12-14], like the known exosomes of many human cells. These observations strengthen the hypothe- sis of a multivesicular origin for the nanovesicles re- leased by Dictyostelium cells and emphasize the role of the nature of the lipids in the formation and function of Dictyostelium vesicles. 3.2. Vesicle-Mediated Transfer of Hoechst 33342 to the Nuclei of Human Leukemia Resistant Cells, K562r HO342-loaded nanovesicles allowed to label the nuclei of naive Dictyostelium cells, thus overcoming their con- stitutive resistance to the free dye [8]. The ability of these nanovesicles, to transfer HO342 to the nuclei of human leukemia living cells, was investigated by adding the nanovesicles (×50) to multidrug resistant K562r cells (Figure 2). Four cells were observed by phase contrast microscopy after about 4 h incubation (Figures 2(a), (c), (e) and (g)). The efficient transfer of HO342 to the cell nuclei was observed under UV excitation. The simulta- neous observation of the same cells with white light and UV excitation (Figures 2(b), (d), (f) and (h)) clearly showed that the HO342-stained nuclei were inside the K562r cells. Thus, the Dictyostelium nanovesicle-medi- ated HO342 delivery had overcome the induced resis- tance of K562r cells to the vital staining of their nuclei. 3.3. Vesicle-Mediated Transfer of Hypericin to the Golgi of Human Cervix Carcinoma HeLa Cells Hypericin transfer to target cells was first visualized us- ing human fibroblasts (HS68) as control cells and with HeLa cells used as a model of tumoral cells. After a 1-h incubation in the presence of hypericin packaged into nanovesicles, a fluorescent signal was detected almost exclusively in the perinuclear area of HeLa cells (Figure 3). Under these experimental conditions, neither the cell plasma membrane, nor the nucleus, was labeled by the hydrophobic cargo molecule. Similar experiments were conducted using free hy- pericin, i.e. not encapsulated into vesicles, for compari- son. After only a 30-min incubation, HeLa cells became round-shaped, the typical morphology of dying cells that have lost their adhesion properties (Figure 3). This result demonstrates that the nanovesicles vectorize significant amounts of drug, but also prevent the uncontrolled cell Figure 2. Transfer of HO342 mediated by the Dictyostelium nanovesicles to the nuclei of living human leukaemia cells, K562r. Cells were incubated (v/v) with these nanovesicles (×50). Four different K562r cells are shown, in the range of incubation time 3 h 40 - 4 h 10, as observed either with phase contrast (×40 CP objective) (a, c, e, g), or with both white light and UV fluorescence microscopy (×40 UV objec- tive) (b, d, f, h). Figure reproduced with permission from [8]. Copyright © 2011 SciRes. JBNB
 A New Biological Strategy for Drug Delivery: Eucaryotic Cell-Derived Nanovesicle497 Figure 3. Light-transmission and fluorescence microscopy of hypericin internalization within human target cells. Hu- man fibroblasts (HS68) (a) and HeLa cells (b and c) were incubated at 37˚C in the dark for the indicated times, in the presence of 7 µM hypericin packaged within Dictyostelium nanovesicles (a and b) or free in DMEM (c). Figure repro- duced with permission from [9]. death triggered by free hypericin under the blue light used for observation. To check the intracellular localization of hypericin de- livered by the nanovesicles into HeLa cells, co-localiza- tion studies were performed with fluorescent markers of the Golgi apparatus (BODIPY ceramide) and of lysosomes (LysoTracker). Within 1 h, hypericin was shown to co- accumulate with BODIPY into the Golgi apparatus, and the hypericin-loaded nanovesicles were efficient for photodynamic killing of HeLa cells [9]. The previous studies were initiated to investigate the potential use of Dictyostelium cell-released nanovesicles as in vitro drug carriers for cancer therapy. Dictyostelium cells were able to release into the culture medium nano- vesicles loaded either with HO342 or with hypericin, a photosensitizer differing in charge and hydrophobicity from the DNA marker. Additionally, these nanovesicles were shown to respectively label human leukaemic re- sistant cells K562r, and human cervix carcinoma, HeLa, cells. It is worth stressing that the two cargo molecules, HO342 and hypericin, were transferred to two distinct intracellular locations, namely, the nucleus for HO342 and the Golgi apparatus for hypericin. Vesicular-mediated delivery of hypericin depicted good photo efficiency for killing HeLa cells. Using ve- sicular hypericin, instead of free hypericin, ensures a better control of drug cell loading, by an easy manipula- tion of the amount of vesicles incubated with the cells. Most importantly, the vesicular delivery prevents the det- rimental aggregation of free hypericin in aqueous media. 4. Discussion Cell membranes are a structured self-assembly of lipids interacting with proteins and in contact with both exter- nal medium and the internal aqueous solvent and solutes. They constantly form lipid vesicles that either move in- ternally from the cell membrane to sites within the cell or are externalized. These vesicles are formed of a selection of membrane lipids and are characterized by specific properties, either of fusion and/or of targeting, that give them their dynamic properties, allowing the exchange of materials and informations. From prokaryotic unicellular organisms to primitive eukaryotes, at the border between the vegetal and animal kingdoms, like the amoebae Dictyosteli um di sc oideum up to mammalian cells [15] the formation of extracellular vesicles of different sizes, origins and functions, was shown to be a property of almost all cell types. Thus, extracellular vesicles have been described as originating from almost all mammalian cell types: human blood cells (platelets [16], B lymphocytes [15], dendritic cells [17], but also from intestinal epithelial cells [18] or from dif- ferent pathological cells [15]. All these extracellular vesicles are examples of “vesi- cles shipping extracellular messages” [19] and “trigger- ing intercellular communication” from one cell to an- other, either by fusion with the target cell [20] and/or by allowing internalization of signaling molecules [15,21]. These extracellular vesicles are, therefore, also potential Trojan Horses for drug delivery to target cells. The exosomes, originating from the endosomal traffic [22], are mostly considered as candidates to develop ge- netic vaccines for immunotherapy [23], but have recently been also considered for drug delivery [24]. However, as mentioned by these authors, many “critical obstacles to the clinical translation of exosome-based therapy” re- main. The growth of Dictyostelium cells in a semi-de- fined medium, without any serum, as compared to more complex human cell media, might favor this simple eu- karyotic cell model for solving some of the remaining problems. Moreover, it is to be stressed, that our drug delivery device, based on a detoxification process, in- cludes the Dictyostelium cell conditioning of the thera- peutic drug into the vesicles that will be released. There- fore, no drug internalization agents into the vesicles are needed, as the ones in exosome-based therapy. Bacteri- ally derived particles have also to be further loaded with therapeutic agents, in order to be efficient carriers [25]. Therefore, among all the cell-derived extracellular vesi- cles, Dictyostelium nanovesicles present the unique ad- vantage of being cell-engineered for drug delivery. With regard to a potential strong immunogenicity of these nanovesicles, due to their microbial origin, which might be a serious drawback for their use in vivo, it has already been documented for bacterial derived particles. By contrast, a first in vivo study upon the immunogenic- ity of Dictyostelium nanovesicles, intravenously injected Copyright © 2011 SciRes. JBNB
 A New Biological Strategy for Drug Delivery: Eucaryotic Cell-Derived Nanovesicle 498 twice in the tail of Balb/C mice, has shown a specific antibody response, but no pyrogenic response nor any inflammation, as measured by five pertinent cytokines (study performed by Genosafe, Evry, France). This should minimize the risk of undesirable immune responses upon in vivo administration of these biological nanovesicles, such as those triggered by tumor-released exosomes [26]. 5. Conclusions Our work indicates that D. discoideum is a bioengineer- ing designer able to formulate vesicular drug carriers. To our knowledge, this is the first study describing cell-en- gineered vesicles able to both load a therapeutic mole- cule and vectorize it into human cells, as we have shown with the important antitumoral photosensitizer, hypericin [9]. Other characteristics of Dictyostelium vesicles appear interesting for targeting purpose; namely, the presence of discoidins I and II [9], which bind to the cell membrane with a specificity for galactose-related residues, might be of interest for lectin-mediated drug delivery [27]. The targeting strategy, tested with bacterially derived vesicles [25], by using bi-specific antibodies, with one arm rec- ognizing a component of the vesicle surface, the other a cell surface receptor of the target cell, might also be ap- plied to Dictyostelium nanovesicles. Another vectoriza- tion strategy could be developed by using Dictyostelium cells cultured in the presence of magnetic nanoparticles to engineer magnetic nanovesicles [28]. With regard to the possibility of using Dictyostelium nanovesicles in therapy, many problems remain to be solved. A study to elucidate the membrane events in- volved in cell entry of the drug vectorized by the nano- vesicles should be performed. For further in vivo ap- proaches, toxicity and allergenic properties of more puri- fied nanovesicle preparations are to be characterized. Taking into account the described culture of Dictyos- telium cells in bioreactors [29], a drug vectorization strat- egy could, indeed, be developed at a large pharmacol- ogical scale. 6. Acknowledgements Eric Larquet (CNRS, UMR7590, UPMC, IMPMC, Paris, France) is kindly acknowledged for the cryoelectron mi- croscopy measurements and Benoit Dubertret (CNRS UPR A0005, UPMC, ESPCI, Paris, France) for the gift of the CdSe/CdZnS quantum dots. REFERENCES [1] W. M. Pardridge, “Molecular Trojan Horses for Blood- Brain Barrier Drug Delivery,” Current Opinion in Phar- macology, Vol. 6, No. 5, 2006, pp. 494-500. doi:10.1016/j.coph.2006.06.001 [2] Y. Seow and M. J. Wood, “Biological Gene Delivery Vehicles: Beyond Viral Vectors,” The American Society of Gene Therapy, Vol. 17, No. 5, 2009, pp. 767-777. [3] K. M. Kroeger, A. K. M. G. Muhammad, G. J. Baker, H. Assi, M. K. Wibowo, W. Xiong, K. Yagiz, M. Candolfi, P. R. Lowenstein and M. G. Castro, “Gene Therapy and Virotherapy: Novel Therapeutic Approaches for Brain Tumors,” Discovery Medicine, Vol. 10, No. 53, 2010, pp. 293-304. [4] A. Villaverde, “Nanotechnology, Bionanotechnology and Microbial Cell Factories,” Microbial Cell Factories, Vol. 9, 2010, pp. 53-56. doi:10.1186/1475-2859-9-53 [5] I. Tatischeff, F. Lavialle, C. de Paillerets, H. Weintraub, G. Tham and A. Alfsen, “Carcinogenic Benzo(a)pyrene and Non-Carcinogenic Benzo(e)pyrene Discriminated by Dictyostelium discoideum Cells through Internalization,” In: P. Garrigues and M. Lamotte, Eds., Polycyclic Aro- matic Compounds, PAH XIII, Gordon and Breach Science Publishers, Switzerland, 1993, pp. 695-702. [6] I. Tatischeff and F. Lavialle, “Immunological Evidence of a P-Glycoprotein in the Microorganism Dictyostelium,” Comptes Rendus de l’Académie des Sciences III (Paris), Vol. 316, No. 6, 1993, pp. 560-563. [7] I. Tatischeff, M. Bomsel, C. de Paillerets, H. Durand, B. Geny, D. Segretain, E. Turpin and A. Alfsen, “Dictyos- telium discoideum Cells Shed Vesicles with Associated DNA and Vital Stain Hoechst 33342,” Cellular and Mo- lecular Life Sciences, Vol. 54, No. 5, 1998, pp. 476-487. doi:10.1007/s000180050176 [8] I. Tatischeff, F. Lavialle, S. Pigaglio-Deshayes, C. Pé- choux-Longin, L. Chinsky and A. Alfsen, “Dictyostelium Extracellular Vesicles Containing Hoechst 33342 Trans- fer the Dye into the Nuclei of Living Cells: A Fluores- cence Study,” Journal of Fluorescence, Vol. 18, No. 2, 2008, pp. 319-328. doi:10.1007/s10895-007-0271-4 [9] F. Lavialle, S. Deshayes, F. Gonnet, E. Larquet, S. G. Kruglik, N. Boisset, R. Daniel, A. Alfsen and I. Ta- tischeff, “Nanovesicles Released by Dictyostelium Cells: A Potential Carrier for Drug Delivery,” International Journal of Pharmaceutics, Vol. 380, No. 1-2, 2009, pp. 206-215. doi:10.1016/j.ijpharm.2009.06.039 [10] D. J. Watts and M. Ashworth, “Growth of Myxameobae of the Cellular Slime Mould Dictyostelium discoideum in Axenic Culture,” Biochemical Journal, Vol. 119, No. 2, 1970, pp. 71-174. [11] J. Gruenberg, “Lipids in Endocytic Membrane Transport and Sorting,” Current Opinion in Cell Biology, Vol. 15, No. 4, 2003, pp. 382-388. doi:10.1016/S0955-0674(03)00078-4 [12] T. Kobayashi, K. Startchev, A. J. Whitney and J. Gruen- berg, “Localization of Lysobisphosphatidic Acid-Rich Membrane Domains in Late Endosomes,” Biological Chemistry, Vol. 382, No. 3, 2001, pp. 483-485. doi:10.1515/BC.2001.059 [13] T. Kobayashi, M. H. Beuchat, J. Chevallier, A. Makino, N. Mayran, J. M. Escola, C. Lebrand, P. Cosson, T. Ko- Copyright © 2011 SciRes. JBNB
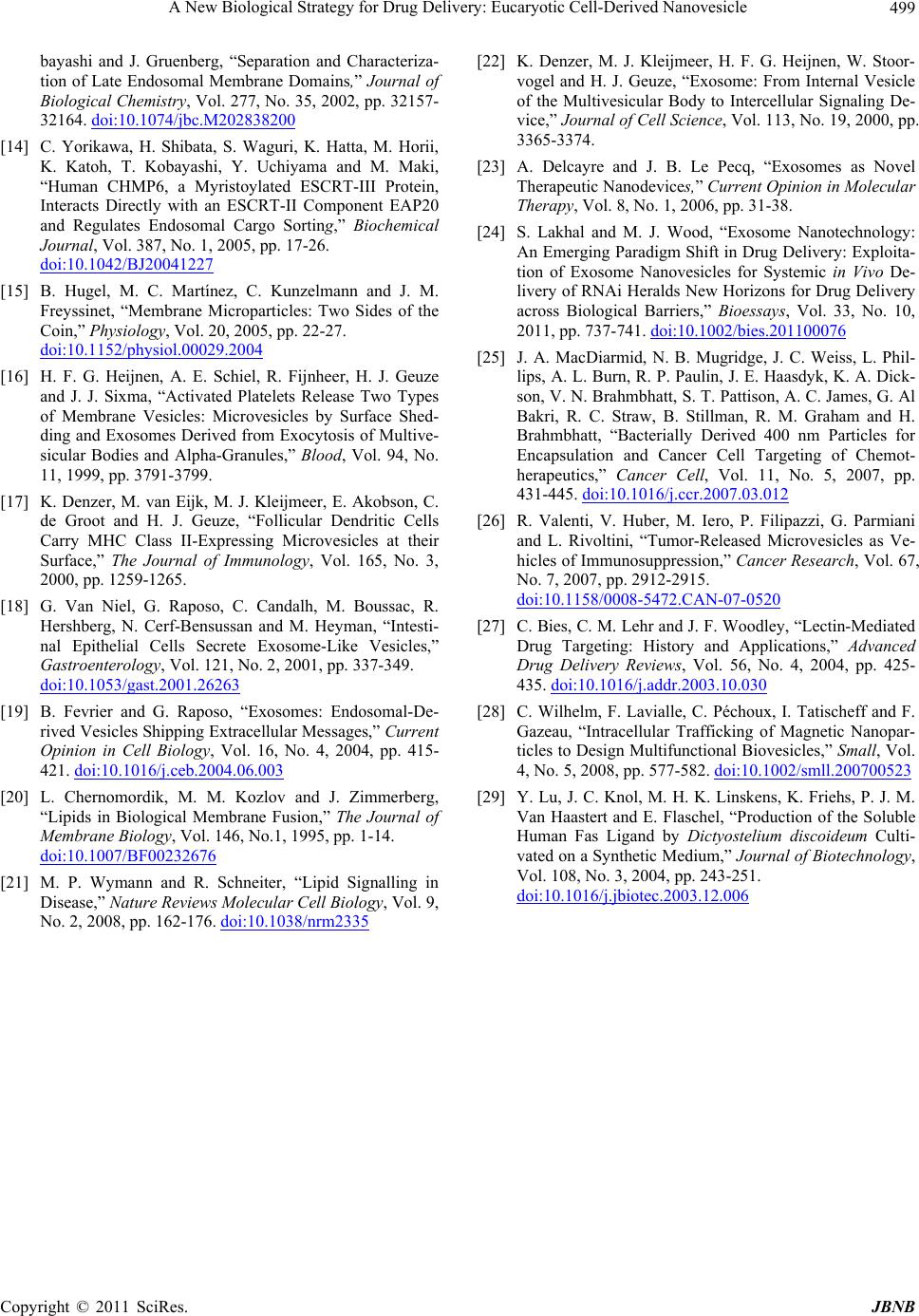 A New Biological Strategy for Drug Delivery: Eucaryotic Cell-Derived Nanovesicle Copyright © 2011 SciRes. JBNB 499 bayashi and J. Gruenberg, “Separation and Characteriza- tion of Late Endosomal Membrane Domains,” Journal of Biological Chemistry, Vol. 277, No. 35, 2002, pp. 32157- 32164. doi:10.1074/jbc.M202838200 [14] C. Yorikawa, H. Shibata, S. Waguri, K. Hatta, M. Horii, K. Katoh, T. Kobayashi, Y. Uchiyama and M. Maki, “Human CHMP6, a Myristoylated ESCRT-III Protein, Interacts Directly with an ESCRT-II Component EAP20 and Regulates Endosomal Cargo Sorting,” Biochemical Journal, Vol. 387, No. 1, 2005, pp. 17-26. doi:10.1042/BJ20041227 [15] B. Hugel, M. C. Martínez, C. Kunzelmann and J. M. Freyssinet, “Membrane Microparticles: Two Sides of the Coin,” Physiology, Vol. 20, 2005, pp. 22-27. doi:10.1152/physiol.00029.2004 [16] H. F. G. Heijnen, A. E. Schiel, R. Fijnheer, H. J. Geuze and J. J. Sixma, “Activated Platelets Release Two Types of Membrane Vesicles: Microvesicles by Surface Shed- ding and Exosomes Derived from Exocytosis of Multive- sicular Bodies and Alpha-Granules,” Blood, Vol. 94, No. 11, 1999, pp. 3791-3799. [17] K. Denzer, M. van Eijk, M. J. Kleijmeer, E. Akobson, C. de Groot and H. J. Geuze, “Follicular Dendritic Cells Carry MHC Class II-Expressing Microvesicles at their Surface,” The Journal of Immunology, Vol. 165, No. 3, 2000, pp. 1259-1265. [18] G. Van Niel, G. Raposo, C. Candalh, M. Boussac, R. Hershberg, N. Cerf-Bensussan and M. Heyman, “Intesti- nal Epithelial Cells Secrete Exosome-Like Vesicles,” Gastroenterology, Vol. 121, No. 2, 2001, pp. 337-349. doi:10.1053/gast.2001.26263 [19] B. Fevrier and G. Raposo, “Exosomes: Endosomal-De- rived Vesicles Shipping Extracellular Messages,” Current Opinion in Cell Biology, Vol. 16, No. 4, 2004, pp. 415- 421. doi:10.1016/j.ceb.2004.06.003 [20] L. Chernomordik, M. M. Kozlov and J. Zimmerberg, “Lipids in Biological Membrane Fusion,” The Journal of Membran e Biolog y, Vol. 146, No.1, 1995, pp. 1-14. doi:10.1007/BF00232676 [21] M. P. Wymann and R. Schneiter, “Lipid Signalling in Disease,” Nature Reviews Molecular Cell Biology, Vol. 9, No. 2, 2008, pp. 162-176. doi:10.1038/nrm2335 [22] K. Denzer, M. J. Kleijmeer, H. F. G. Heijnen, W. Stoor- vogel and H. J. Geuze, “Exosome: From Internal Vesicle of the Multivesicular Body to Intercellular Signaling De- vice,” Journal of Cell Science, Vol. 113, No. 19, 2000, pp. 3365-3374. [23] A. Delcayre and J. B. Le Pecq, “Exosomes as Novel Therapeutic Nanodevices,” Current Opinion in Molecular Therapy, Vol. 8, No. 1, 2006, pp. 31-38. [24] S. Lakhal and M. J. Wood, “Exosome Nanotechnology: An Emerging Paradigm Shift in Drug Delivery: Exploita- tion of Exosome Nanovesicles for Systemic in Vivo De- livery of RNAi Heralds New Horizons for Drug Delivery across Biological Barriers,” Bioessays, Vol. 33, No. 10, 2011, pp. 737-741. doi:10.1002/bies.201100076 [25] J. A. MacDiarmid, N. B. Mugridge, J. C. Weiss, L. Phil- lips, A. L. Burn, R. P. Paulin, J. E. Haasdyk, K. A. Dick- son, V. N. Brahmbhatt, S. T. Pattison, A. C. James, G. Al Bakri, R. C. Straw, B. Stillman, R. M. Graham and H. Brahmbhatt, “Bacterially Derived 400 nm Particles for Encapsulation and Cancer Cell Targeting of Chemot- herapeutics,” Cancer Cell, Vol. 11, No. 5, 2007, pp. 431-445. doi:10.1016/j.ccr.2007.03.012 [26] R. Valenti, V. Huber, M. Iero, P. Filipazzi, G. Parmiani and L. Rivoltini, “Tumor-Released Microvesicles as Ve- hicles of Immunosuppression,” Cancer Research, Vol. 67, No. 7, 2007, pp. 2912-2915. doi:10.1158/0008-5472.CAN-07-0520 [27] C. Bies, C. M. Lehr and J. F. Woodley, “Lectin-Mediated Drug Targeting: History and Applications,” Advanced Drug Delivery Reviews, Vol. 56, No. 4, 2004, pp. 425- 435. doi:10.1016/j.addr.2003.10.030 [28] C. Wilhelm, F. Lavialle, C. Péchoux, I. Tatischeff and F. Gazeau, “Intracellular Trafficking of Magnetic Nanopar- ticles to Design Multifunctional Biovesicles,” Small, Vol. 4, No. 5, 2008, pp. 577-582. doi:10.1002/smll.200700523 [29] Y. Lu, J. C. Knol, M. H. K. Linskens, K. Friehs, P. J. M. Van Haastert and E. Flaschel, “Production of the Soluble Human Fas Ligand by Dictyostelium discoideum Culti- vated on a Synthetic Medium,” Journal of Biotechnology, Vol. 108, No. 3, 2004, pp. 243-251. doi:10.1016/j.jbiotec.2003.12.006
|