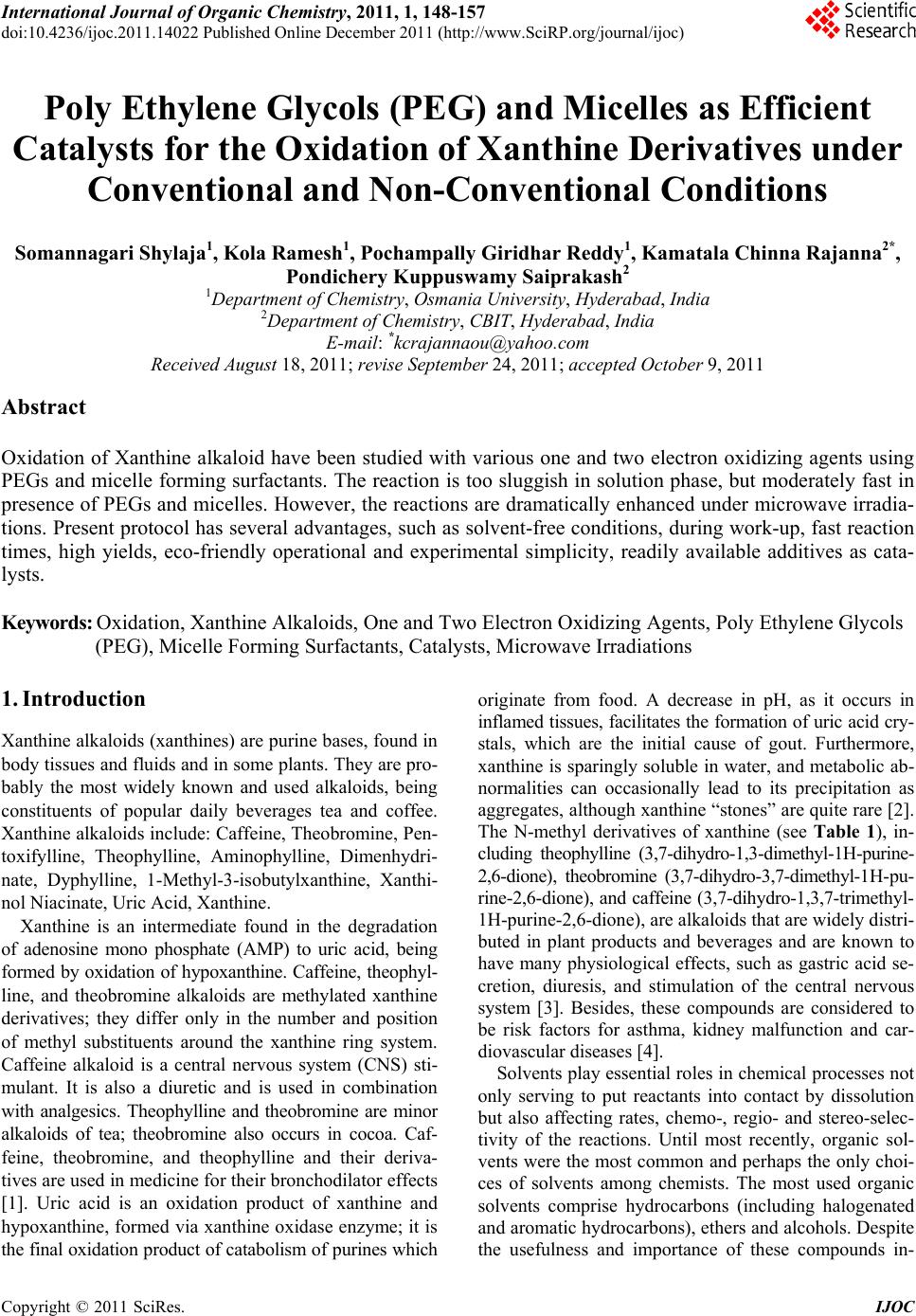 International Journal of Organic Chemistry, 2011, 1, 148-157 doi:10.4236/ijoc.2011.14022 Published Online December 2011 (http://www.SciRP.org/journal/ijoc) Copyright © 2011 SciRes. IJOC Poly Ethylene Glycols (PEG) and Micelles as Efficient Catalysts for the Oxidation of Xanthine Derivatives under Conventional and Non-Conventional Conditions Somannagari Shylaja1, Kola Ramesh1, Pochampally Giridhar Reddy1, Kamatala Chinna Rajanna2*, Pondichery Kuppuswamy Saiprakash2 1Department of Chemistry, Osmania University, Hyderabad, India 2Department of Chemistry, CBIT, Hyderabad, India E-mail: *kcrajannaou@yahoo.com Received August 18, 2011; revise September 24, 2011; accepted October 9, 2011 Abstract Oxidation of Xanthine alkaloid have been studied with various one and two electron oxidizing agents using PEGs and micelle forming surfactants. The reaction is too sluggish in solution phase, but moderately fast in presence of PEGs and micelles. However, the reactions are dramatically enhanced under microwave irradia- tions. Present protocol has several advantages, such as solvent-free conditions, during work-up, fast reaction times, high yields, eco-friendly operational and experimental simplicity, readily available additives as cata- lysts. Keywords: Oxidation, Xanthine Alkaloids, One and Two Electron Oxidizing Agents, Poly Ethylene Glycols (PEG), Micelle Forming Surfactants, Catalysts, Microwave Irradiations 1. Introduction Xanthine alkaloids (xanthines) are purine bases, found in body tissues and fluids and in some plants. They are pro- bably the most widely known and used alkaloids, being constituents of popular daily beverages tea and coffee. Xanthine alkaloids include: Caffeine, Theobromine, Pen- toxifylline, Theophylline, Aminophylline, Dimenhydri- nate, Dyphylline, 1-Methyl-3-isobutylxanthine, Xanthi- nol Niacinate, Uri c A ci d , Xanthine. Xanthine is an intermediate found in the degradation of adenosine mono phosphate (AMP) to uric acid, being formed by oxidation of hypoxanthine. Caffeine, theophyl- line, and theobromine alkaloids are methylated xanthine derivatives; they differ only in the number and position of methyl substituents around the xanthine ring system. Caffeine alkaloid is a central nervous system (CNS) sti- mulant. It is also a diuretic and is used in combination with analgesics. Theophylline and theobromine are minor alkaloids of tea; theobromine also occurs in cocoa. Caf- feine, theobromine, and theophylline and their deriva- tives are used in medicine for their bronchodilator effects [1]. Uric acid is an oxidation product of xanthine and hypoxanthine, formed via xanthine oxidase enzyme; it is the final oxidation product of catabolism of purines which originate from food. A decrease in pH, as it occurs in inflamed tissues, facilitates the formation of uric acid cry- stals, which are the initial cause of gout. Furthermore, xanthine is sparingly soluble in water, and metabolic ab- normalities can occasionally lead to its precipitation as aggregates, although xanthine “stones” are quite rare [2]. The N-methyl derivatives of xanthine (see Table 1), in- cluding theophylline (3,7-dihydro-1,3-dimethyl-1H-purine- 2,6-dione), theobromine (3,7-dihydro-3,7-dimethyl-1H-pu- rine-2,6-dione), and caffeine (3,7-dihydro-1,3,7-trim ethyl- 1H-purine-2,6-d ione), ar e alkaloids th at are widely distri- buted in plant products and beverages and are known to have many physiological effects, such as gastric acid se- cretion, diuresis, and stimulation of the central nervous system [3]. Besides, these compounds are considered to be risk factors for asthma, kidney malfunction and car- diovascular diseases [4]. Solvents play essential roles in ch emical processes not only serving to put reactants into contact by dissolution but also affecting rates, chemo-, regio- and stereo-selec- tivity of the reactions. Until most recently, organic sol- vents were the most common and perhaps the only choi- ces of solvents among chemists. The most used organic solvents comprise hydrocarbons (including halogenated and aromatic hydrocarbons), ethers and alcohols. Despite the usefulness and importance of these compounds in-
 149 S. SHYLAJA ET AL. Table 1. NMR and Mass Spectral data for selected reaction products. Spectral data Entry Substrates Product m/z 1H NMR 1 Caffeine 1,3,7-Try methyl uric acid 210δ 3.35(N-CH3); δ 3.41(N-CH3) δ 3.73(N-CH3); δ 13.49(O-H) 2 Theobromine 3,7-Dimethyl uric acid 196δ 9.45(N-H); δ 3.24(N-CH3) δ 3.73(N-CH3); δ 1 3.49(O-H) 3 Theophyllene 1,3-Dimethyl uric acid 196δ 3.35(N-CH3); δ 3.41(N-CH3) δ 13.43(O-H); δ 13.92(N-H) 4 Xanthine Uric acid 168δ 13.91(N-H); δ 9.48( N-H) δ 13.41(O-H); δ 15.53(N-H) 5 Hypoxanthine Uric acid 168δ 13.91(N-H); δ 9.48(N-H ) δ 13.41(O-H); δ 15.53(N-H) organic reactions they undoubtedly have high flammabil- ity and volatility, their hazardness and toxicity have a detrimental impact on the environment. In recent past this scenario has been substantially changed by environ- mentally benign substitutes (green solvents) for volatile and toxic organic solvents under the concepts of Green Chemistry and green reaction processes [5,6]. Poly (eth- ylene glycol) (PEG) [7-9], a biologically acceptable polymer used extensively in drug delivery and in bio conjugates as tools for diagnostics has been used as a solvent medium support for various transformations. In recent times PEG has surpassed even ionic liquids in forefront of research PEG is economically cheap and environmentally safe. To date some of the more impor- tant reactions have been carried out and investigated in PEG [7-23]. Besides the use of safer solvents, in recent past increasing attention has also been paid for designing eco friend- ly reactions using environmentally safe and economically cheap reagents to prevent environmental pollutions according to the guidelines given by Paul An- astas and John Warner [5]. Recent reviews and publica- tions [24-27] in the field of solvent-free organic synthesis revealed that organic reactions performed under these conditions are not only simple but also satisfy both eco- nomical and environmental demands by replacing the toxic solvents. Solvent free reactions further, have gained much attention because of their enhanced selectivity, mild reaction conditions, and associated ease of manipu- lation. Apart from solvent free organic synthesis, quite some attention has been paid to develop methodologies using ultrasound and microwaves. Methods developed under sonicated [28-33] and microwave irradiated condi- tions [34-36] proved to good tools to save energy and reduce reaction times to a greater extent. Considerable efforts were also diverted to use economically cheap and environmentally safe reagents as catalysts to design or- ganic synthesis. A survey of literature reveals that Mi- celles act as a kind of micro reactors and enhance the rate and selectivity of a variety of chemical and biochemical reactions. A close parallelism between enzymatic reac- tions and micellar reactions has attracted the attention of several synthetic organic chemists and biochemists [37- 40]. Far the past several years, our group has focused its attention in designing synthetic protocols using a variety of eco friendly materials such as micelle-forming surfac- tants and unconventional energy sources (such as mi- crowave irradiation and ultrasound) to enhance Vilsmei- er-Haack (VH) and Hunsdiecker reactions [40-43]. Dr- amatic rate accelerations followed by an increase in the product yield were observed in all these reactions. En- couraged by the striking features and applications of PEGs, micelles, and microwave irradiation in chemical processes and organic synthesis, coupled with zeal to employ atom economy eco-friendly reagents, the author proposes to take up Oxid ation of certain biologically im- portant compounds such as xanthine (XAN), hypoxan- thine (HXAN), caffeine (CAF), theophylline (TPL), theo- bromine (TBR), using commonly available laboratory desktop eco friendly reagents such as hydrogen peroxide (H2O2), tetra butyl Hydrogen peroxide (TBHP), Potas- sium peroxy disulfate (K2S2O8), Potassium peroxy di- phosphate (K4P2O8), Sodium perborate (NaBO4), Potas- sium periodate (KIO4), Pyridinium chloro chromate (PCC) and Quinolonium chloro chromate (QCC). The proposed work is taken up different stages 1) to conduct the reac- tions under and microwav e conditions to save energy; 2) to conduct the reactions in a mortar by grinding with a pestle under solvent-free conditions or by using micro- wave irradiation under solid phase conditi ons. 2. Experimental Details 2.1. Materials and Methods All chemicals used were of analytical grade. Doubly dis- tilled water (distilled over alkaline KMnO4 and acid di- chromate in an all glass apparatus) was used whenever required. Acetonitrile and other solv ents were HPLC gra- de and used as such throug hout the work. Copyright © 2011 SciRes. IJOC
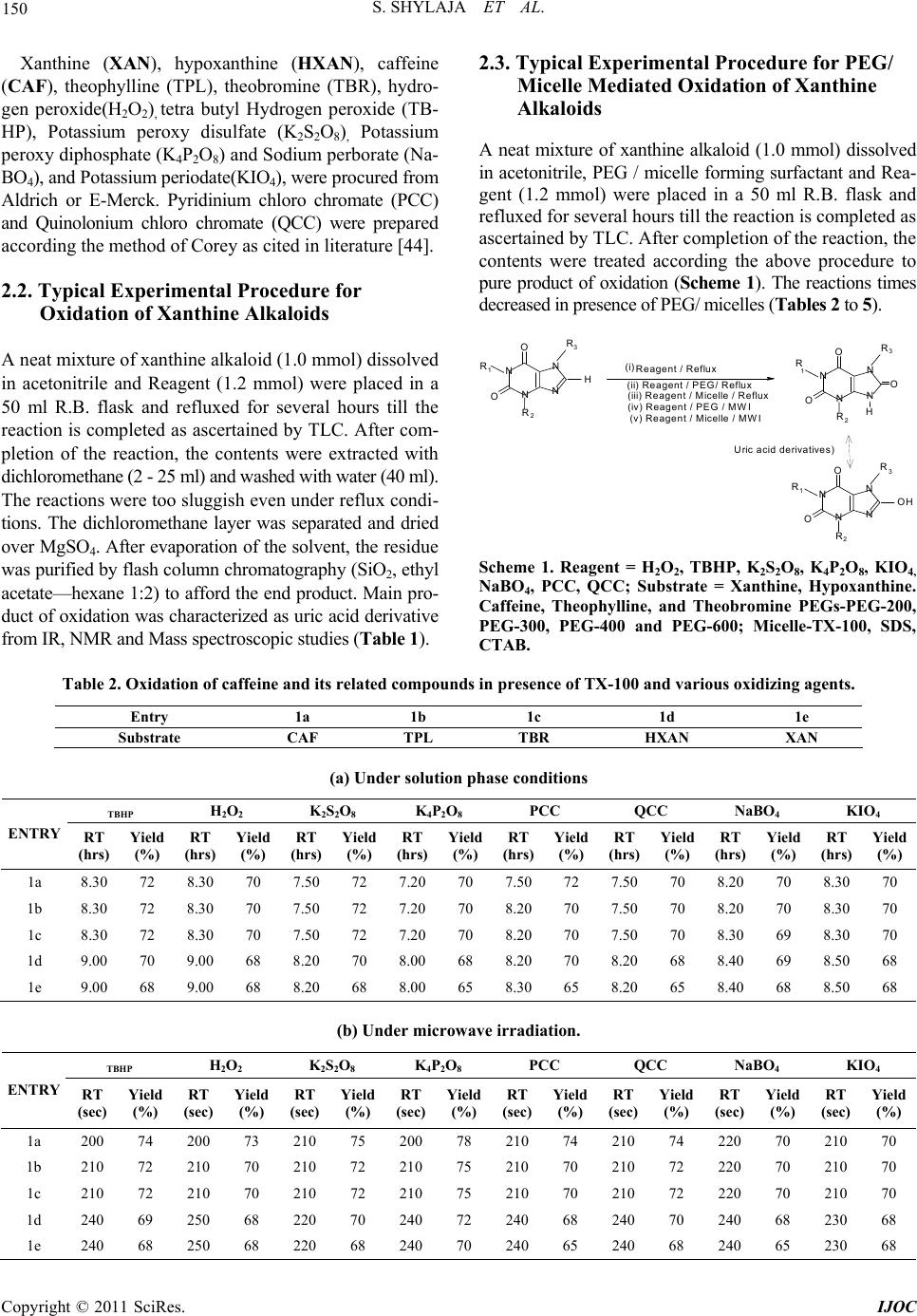 S. SHYLAJA ET AL. 150 Xanthine (XAN), hypoxanthine (HXAN), caffeine (CAF), theophylline (TPL), theobromine (TBR), hydro- gen peroxide(H2O2), tetra butyl Hydrogen peroxide (TB- HP), Potassium peroxy disulfate (K2S2O8), Potassium peroxy diphosphate (K4P2O8) and Sodium perborate (Na- BO4), and Potassium periodate(KIO4), were procured from Aldrich or E-Merck. Pyridinium chloro chromate (PCC) and Quinolonium chloro chromate (QCC) were prepared according the method of Corey as cited in literature [44]. 2.2. Typical Experimental Procedure for Oxidation of Xanthine Alkaloids A neat mixture of xanthine alkaloid (1.0 mmol) dissolved in acetonitrile and Reagent (1.2 mmol) were placed in a 50 ml R.B. flask and refluxed for several hours till the reaction is completed as ascertained by TLC. After com- pletion of the reaction, the contents were extracted with dichlorom ethan e (2 - 25 ml) a nd wash ed with wat er (4 0 ml). The reactions were too sluggish even under reflux condi- tions. The dichloromethane layer was separated and dried over MgSO4. After evaporation of the solvent, the res idue was purified by flash column chromatography (SiO2, ethyl acetate—hexane 1:2) to afford the end product. Main pro- duct of oxidation was characterized as uric acid derivative from IR, NMR and Mass spectroscopi c studies (Table 1). 2.3. Typical Experimental Procedure for PEG/ Micelle Mediated Oxidation of Xanthine Alkaloids A neat mixture of xanthine alkaloid (1.0 mmol) dissolved in acetonitri le, PEG / micelle forming surfactant and Rea- gent (1.2 mmol) were placed in a 50 ml R.B. flask and refluxed for several hours till the reaction is completed as ascertained by TLC. After completion of the reaction, the contents were treated according the above procedure to pure product of oxidation (Scheme 1). The reactions times decreased in presen ce of PEG/ m icelles (Tables 2 to 5). R1 R R N O O N N N R R R N N N N R1 R R N O O N N NOH H O O O 2 3 1 2 3 2 3 H Uric acid derivatives) Reagent / Reflux (i) (ii) Reagent / PEG/ Reflux (iii) Reagent / Micelle / Reflux (iv) Reagent / PEG / MWI (v) Re a ge n t / M ice lle / M WI Scheme 1. Reagent = H2O2, TBHP, K2S2O8, K4P2O8, KIO4, NaBO4, PCC, QCC; Substrate = Xanthine, Hypoxanthine. Caffeine, Theophylline, and Theobromine PEGs-PEG-200, PEG-300, PEG-400 and PEG-600; Micelle-TX-100, SDS, CTAB. Table 2. Oxidation of caffeine and its related compounds in presence of TX-100 and various oxidizing agents. Entry 1a 1b 1c 1d 1e Substrate CAF TPL TBR HXAN XAN (a) Under solution phase conditions TBHP H2O2 K 2S2O8 K 4P2O8 PCC QCC NaBO4 KIO4 ENTRY RT (hrs) Yield (%) RT (hrs) Yield (%) RT (hrs) Yield (%) RT (hrs) Yield (%) RT (hrs) Yield (%) RT (hrs) Yield (%) RT (hrs) Yield (%) RT (hrs) Yield (%) 1a 8.30 72 8.30 70 7.50 72 7.2070 7.5072 7.5070 8.20 70 8.3070 1b 8.30 72 8.30 70 7.50 72 7.2070 8.2070 7.5070 8.20 70 8.3070 1c 8.30 72 8.30 70 7.50 72 7.2070 8.2070 7.5070 8.30 69 8.3070 1d 9.00 70 9.00 68 8.20 70 8.0068 8.2070 8.2068 8.40 69 8.5068 1e 9.00 68 9.00 68 8.20 68 8.0065 8.3065 8.2065 8.40 68 8.5068 (b) Under microwave irradiation. TBHP H2O2 K 2S2O8 K 4P2O8 PCC QCC NaBO4 KIO4 ENTRY RT (sec) Yield (%) RT (sec) Yield (%) RT (sec) Yield (%) RT (sec) Yield (%) RT (sec) Yield (%) RT (sec) Yield (%) RT (sec) Yield (%) RT (sec) Yield (%) 1a 200 74 200 73 210 75 20078 21074 21074 220 70 21070 1b 210 72 210 70 210 72 21075 21070 21072 220 70 21070 1c 210 72 210 70 210 72 21075 21070 21072 220 70 21070 1d 240 69 250 68 220 70 24072 24068 24070 240 68 23068 1e 240 68 250 68 220 68 24070 24065 24068 240 65 23068 Copyright © 2011 SciRes. IJOC
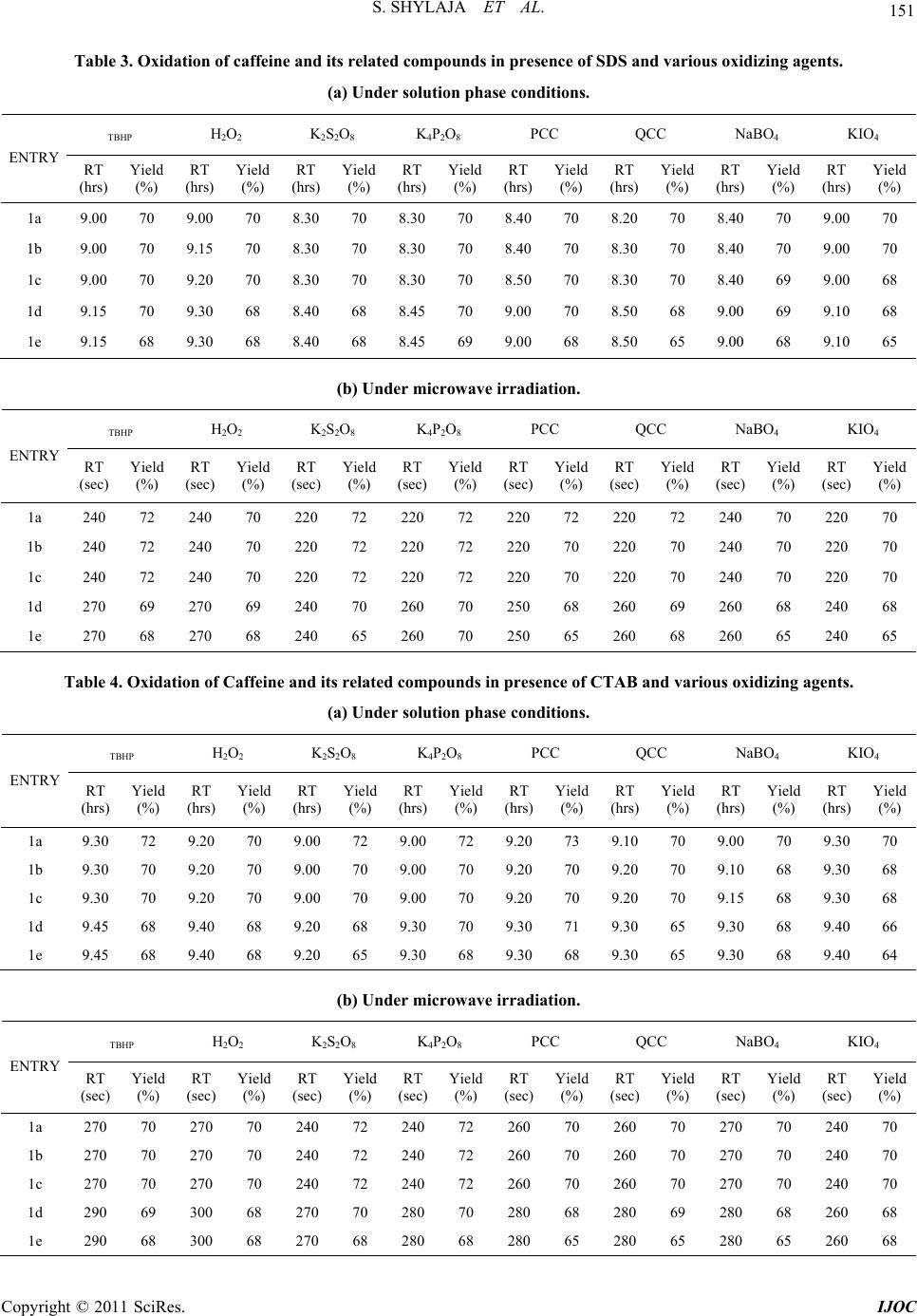 151 S. SHYLAJA ET AL. Table 3. Oxidation of caffeine and its related compounds in presence of SDS and various oxidizing agents. (a) Under solution phase conditions. TBHP H2O2 K 2S2O8 K 4P2O8 PCC QCC NaBO4 KIO4 ENTRY RT (hrs) Yield (%) RT (hrs) Yield (%) RT (hrs) Yield (%) RT (hrs) Yield (%) RT (hrs) Yield (%) RT (hrs) Yield (%) RT (hrs) Yield (%) RT (hrs) Yield (%) 1a 9.00 70 9.00 70 8.30 70 8.3070 8.4070 8.2070 8.40 70 9.0070 1b 9.00 70 9.15 70 8.30 70 8.3070 8.4070 8.3070 8.40 70 9.0070 1c 9.00 70 9.20 70 8.30 70 8.3070 8.5070 8.3070 8.40 69 9.0068 1d 9.15 70 9.30 68 8.40 68 8.4570 9.0070 8.5068 9.00 69 9.1068 1e 9.15 68 9.30 68 8.40 68 8.4569 9.0068 8.5065 9.00 68 9.1065 (b) Under microwave irradiation. TBHP H2O2 K 2S2O8 K 4P2O8 PCC QCC NaBO4 KIO4 ENTRY RT (sec) Yield (%) RT (sec) Yield (%) RT (sec) Yield (%) RT (sec) Yield (%) RT (sec) Yield (%) RT (sec) Yield (%) RT (sec) Yield (%) RT (sec) Yield (%) 1a 240 72 240 70 220 72 220 72 220 72 220 72 240 70 220 70 1b 240 72 240 70 220 72 220 72 220 70 220 70 240 70 220 70 1c 240 72 240 70 220 72 220 72 220 70 220 70 240 70 220 70 1d 270 69 270 69 240 70 260 70 250 68 260 69 260 68 240 68 1e 270 68 270 68 240 65 260 70 250 65 260 68 260 65 240 65 Table 4. Oxidation of Caffeine and its related compounds in presence of CTAB and various oxidizing agents. (a) Under solution phase conditions. TBHP H2O2 K 2S2O8 K 4P2O8 PCC QCC NaBO4 KIO4 ENTRY RT (hrs) Yield (%) RT (hrs) Yield (%) RT (hrs) Yield (%) RT (hrs) Yield (%) RT (hrs) Yield (%) RT (hrs) Yield (%) RT (hrs) Yield (%) RT (hrs) Yield (%) 1a 9.30 72 9.20 70 9.00 72 9.0072 9.2073 9.1070 9.00 70 9.3070 1b 9.30 70 9.20 70 9.00 70 9.0070 9.2070 9.2070 9.10 68 9.3068 1c 9.30 70 9.20 70 9.00 70 9.0070 9.2070 9.2070 9.15 68 9.3068 1d 9.45 68 9.40 68 9.20 68 9.3070 9.3071 9.3065 9.30 68 9.4066 1e 9.45 68 9.40 68 9.20 65 9.3068 9.3068 9.3065 9.30 68 9.4064 (b) Under microwave irradiation. TBHP H2O2 K 2S2O8 K 4P2O8 PCC QCC NaBO4 KIO4 ENTRY RT (sec) Yield (%) RT (sec) Yield (%) RT (sec) Yield (%) RT (sec) Yield (%) RT (sec) Yield (%) RT (sec) Yield (%) RT (sec) Yield (%) RT (sec) Yield (%) 1a 270 70 270 70 240 72 240 72 260 70 260 70 270 70 240 70 1b 270 70 270 70 240 72 240 72 260 70 260 70 270 70 240 70 1c 270 70 270 70 240 72 240 72 260 70 260 70 270 70 240 70 1d 290 69 300 68 270 70 280 70 280 68 280 69 280 68 260 68 1e 290 68 300 68 270 68 280 68 280 65 280 65 280 65 260 68 Copyright © 2011 SciRes. IJOC
 S. SHYLAJA ET AL. Copyright © 2011 SciRes. IJOC 152 Table 5. Oxidation of caffeine and its related compounds in presence of PEG-300 and various oxidizing agents. (a) Under solution phase conditions. TBHP H2O2 K 2S2O8 K 4P2O8 PCC QCC NaBO4 KIO4 ENTRY RT (hrs) Yield (%) RT (hrs) Yield (%) RT (hrs) Yield (%) RT (hrs) Yield (%) RT (hrs) Yield (%) RT (hrs) Yield (%) RT (hrs) Yield (%) RT (hrs) Yield (%) 1a 8.15 70 8.45 70 7.40 72 7.5072 7.4570 7.4572 8.00 70 8.0070 1b 8.15 70 8.45 70 7.40 72 7.5070 8.1570 7.4570 8.20 70 8.0070 1c 8.15 70 8.45 70 7.40 72 7.5070 8.1570 7.4570 8.20 69 8.0070 1d 9.00 68 9.30 68 8.30 70 8.2070 8.3068 8.3068 8.30 68 8.4568 1e 9.00 68 9.30 65 8.30 68 8.2070 8.3068 8.3068 8.30 65 8.4568 (b) Under microwave irradiation. TBHP H2O2 K 2S2O8 K 4P2O8 PCC QCC NaBO4 KIO4 ENTRY RT (sec) Yield (%) RT (sec) Yield (%) RT (sec) Yield (%) RT (sec) Yield (%) RT (sec) Yield (%) RT (sec) Yield (%) RT (sec) Yield (%) RT (sec) Yield (%) 1a 200 74 210 75 200 78 16080 20075 20075 200 73 20073 1b 200 70 210 70 200 72 16076 21072 20074 210 72 20072 1c 200 70 210 70 200 72 16075 21070 20072 200 72 21072 1d 240 68 250 65 220 68 18070 24068 22070 210 68 22068 1e 240 68 250 65 220 65 18068 24065 22068 220 65 22068 2.4. Typical Experimental Procedure for Microwave Irradiated (MWI) Oxidation of Xanthine Alkaloids A neat mixture of xanthine alkaloid (1.0 mmol) dissolved in acetonitrile, PEG/micelle forming surfactant and Re- agent (1.2 mmol) were placed in a 50 ml R.B. flask. The reaction mixture was inserted in a silica gel bath and placed in a laboratory microwave oven and irradiated (700 W) three times for three to five minutes with a pe- riod of 20 seconds time intervals. After completion of the reaction, the contents were treated according the above procedure to pure product of oxidation (Scheme 1). The reactions times decreased from several hours to few mi- nutes under microwave irradiation in presence of PEG/ micelles (Tables 2-5). 3. Results & Discussion Oxidation reactions with xanthine alkaloids were too sluggish in acetonitrile media even under reflux condi- tions with longer reaction times. However, micelle medi- ated and PEG mediated reactions are underwent with moderate progress, which could be seen from the data presented in Table 2-5 and Figures 1-4. These data also depict that nature of Oxidizing agent had significant ef- fect on the rate of oxidation. Peroxide reagents are found superior over other reagents while structural variation of PEG had only a little influence on the rate of oxidation. But when the reactions are conducted in micellar media noticeable rate enhancements were noticed with TX-100 on par with PEG. However, anionic (SDS) and ca- 0 10 20 30 40 50 60 70 80 H2O2 TBHPPDSPDPSPBPCCQCCPPI PEG 200 m edi at ed Caff i ene O xi dat i on R. T (hrs ) Y i eld (%) (a) 0 10 20 30 40 50 60 70 80 H2O2PCC PDPPDSPPIQCC SPBTBHP MWI on PEG 200 mediated oxidation of Caffiene R. T (mi n ) Yield (%) (b) Figure 1. PEG mediated caffeine oxidation with various reagents. (a) Under solution phase; (b) Under Microwave Irradiation.
 153 S. SHYLAJA ET AL. tionic (CTAB) micelle mediated reactions are relatively less effect over TX-100 (Tables 2-4; Figures 3(a), 3(b) 4(a) and 4(b)). It is inter esting to not e that PEG (Table 5; Figures 1, 2(a) and 2(b)), and TX-100 probably be- have in the same way because both of them have poly- oxy ethylene moieties. The efficient catalytic activity o f micelles could be at- tributed to the fact micelles act as “ micro reactors” to fa- cilitate the reactions through electrostatic/hydrophobic interactions operating between reactive species [37-41]. The catalytic activity of PEGs could be explained in similar lines to those of non-ionic micelles due to their structural resemblance. Reaction times are reduced from several hours (6 to 9 hr) to few minutes (6 to 9 minutes) between reactions performed under standard oil-bath conditions (heating under reflux) and microwave irradiations. The observed dramatic rate enhancements under microwave irradiations in the present study could be well explained due to the formation of “molecular radiators” by direct coupling of microwave energy to specific reagents in homogeneous solution (microscopic hotspots) [45-47] and by the elimination of wall effects caused by inverted temperature gradients. 0 10 20 30 40 50 60 70 80 PEG200 PEG300PEG400 PEG600 Caffiene Oxidation with TBHP R.T (hrs ) Yield (%) (a) 0 10 20 30 40 50 60 70 80 PEG200 PEG300PEG400 PEG600 MWI Caffeine Oxidation by TBHP R. T (m i n) Y i el d (% ) (b) Figure 2. Effect of structure of PEG on TBHP oxidation of caffeine (a) Under solution phase; (b) Under microwave Ir- radiation. 0 10 20 30 40 50 60 70 80 H2O2 TBHPPDSPDPSPBPCCQCCPPI Tx medi at ed O x i dat i on of Caff iene R. T (hrs) Yield (%) (a) 0 10 20 30 40 50 60 70 80 H2O2TBHPPDSPDPSPB PCCQCCPPI MW I on TX100 mediat ed Caff i ene O xi dat i on R. T (mi n) Y i el d (% ) (b) Figure 3. TX-100 mediated caffeine oxidation with various reagents. (a) Under solution phase; (b) Under microwave ir- radiation. 0 10 20 30 40 50 60 70 80 TX100 SDS CTAB Mi cel l e m edi at ed TBHP oxidati on of Caff i ene R. T (hrs) Yield (%) (a) 0 10 20 30 40 50 60 70 80 TX100 SDS CTAB MWI on Micelle mediated TBHP Caffiene Oxidation R. T (mi n) Y iel d (%) (b) Figure 4. TBHP oxidation of caffeine under micellar condi- tions. (a) Under micellar conditions in solution phase; (b) Micellar effects under microwave irradiation. Copyright © 2011 SciRes. IJOC
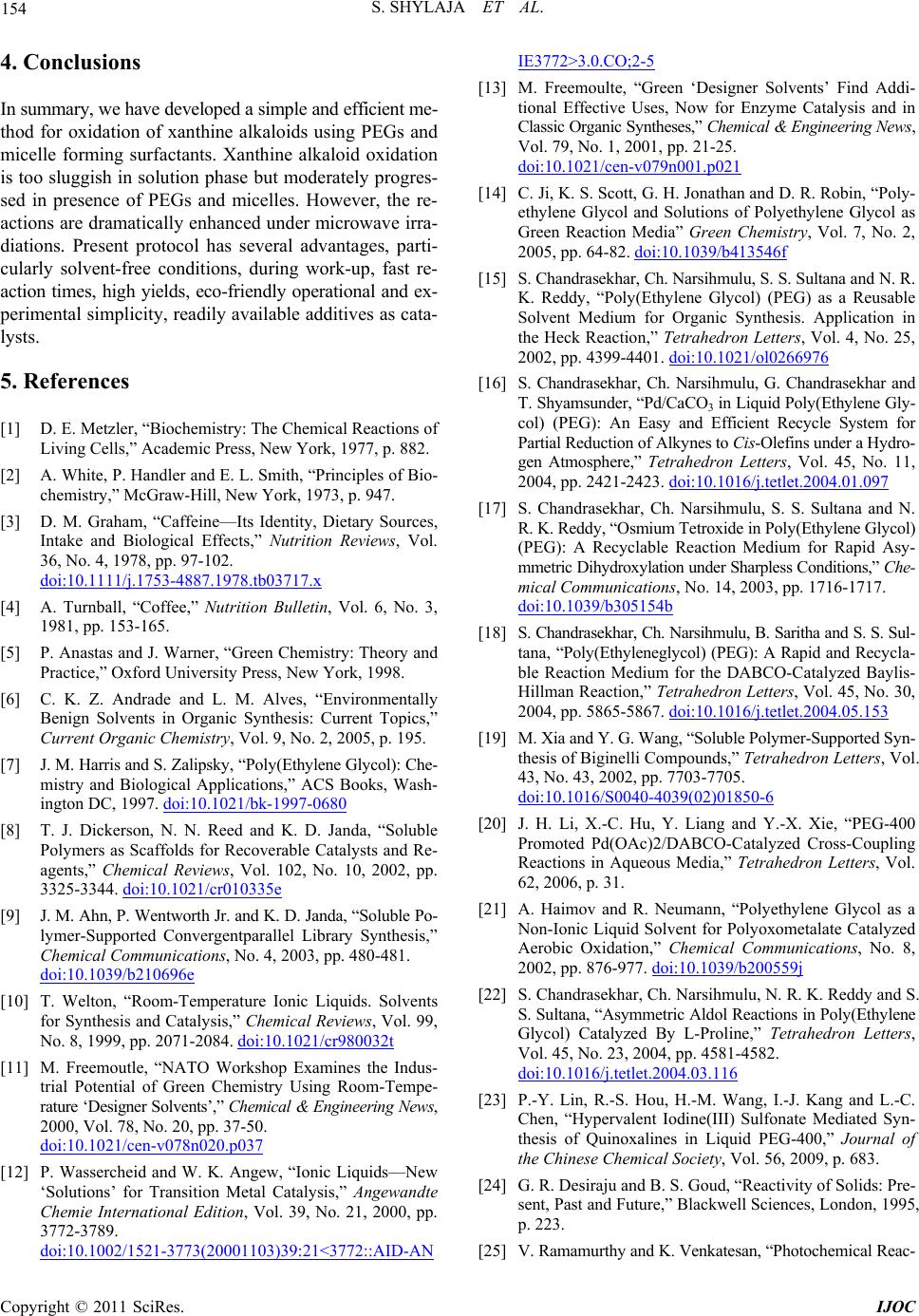 S. SHYLAJA ET AL. 154 4. Conclusions In summary, we have developed a simple and efficient me- thod for oxidation of xanthine alkaloids using PEGs and micelle forming surfactants. Xanthine alkaloid oxidation is too sluggish in solution phase but moderately progres- sed in presence of PEGs and micelles. However, the re- actions are dramatically enhanced under microwave irra- diations. Present protocol has several advantages, parti- cularly solvent-free conditions, during work-up, fast re- action times, high yields, eco-friendly operational and ex- perimental simplicity, readily available additives as cata- lysts. 5. References [1] D. E. Metzler, “Biochemistry: The Chemical Reactions of Living Cells,” Academic Press, New York, 1977, p. 882. [2] A. White, P. Handler and E. L. Smith, “Principles of Bio- chemistry,” McGraw-Hill, New York, 1973, p. 947. [3] D. M. Graham, “Caffeine—Its Identity, Dietary Sources, Intake and Biological Effects,” Nutrition Reviews, Vol. 36, No. 4, 1978, pp. 97-102. doi:10.1111/j.1753-4887.1978.tb03717.x [4] A. Turnball, “Coffee,” Nutrition Bulletin, Vol. 6, No. 3, 1981, pp. 153-165. [5] P. Anastas and J. Warner, “Green Chemistry: Theory and Practice,” Oxford University Press, New York, 1998. [6] C. K. Z. Andrade and L. M. Alves, “Environmentally Benign Solvents in Organic Synthesis: Current Topics,” Current Organic Chemistry, Vol. 9, No. 2, 2005, p. 195. [7] J. M. Harris and S. Zalipsky , “Pol y(Ethylene Gly col): Che- mistry and Biological Applications,” ACS Books, Wash- ington DC, 1997. doi:10.1021/bk-1997-0680 [8] T. J. Dickerson, N. N. Reed and K. D. Janda, “Soluble Polymers as Scaffolds for Recoverable Catalysts and Re- agents,” Chemical Reviews, Vol. 102, No. 10, 2002, pp. 3325-3344. doi:10.1021/cr010335e [9] J. M. Ahn, P. Wentworth Jr. and K. D. Janda, “Soluble Po- lymer-Supported Convergentparallel Library Synthesis,” Chemical Communications, No. 4, 2003, pp. 480-481. doi:10.1039/b210696e [10] T. Welton, “Room-Temperature Ionic Liquids. Solvents for Synthesis and Catalysis,” Chemical Reviews, Vol. 99, No. 8, 1999, pp. 2071-2084. doi:10.1021/cr980032t [11] M. Freemoutle, “NATO Workshop Examines the Indus- trial Potential of Green Chemistry Using Room-Tempe- rature ‘Designer Solvents’,” Chemical & Engineering News, 2000, Vol. 78, No. 20, pp. 37-50. doi:10.1021/cen-v078n020.p037 [12] P. Wassercheid and W. K. Angew, “Ionic Liquids—New ‘Solutions’ for Transition Metal Catalysis,” Angewandte Chemie International Edition, Vol. 39, No. 21, 2000, pp. 3772-3789. doi:10.1002/1521-3773(20001103)39:21<3772::AID-AN IE3772>3.0.CO;2-5 [13] M. Freemoulte, “Green ‘Designer Solvents’ Find Addi- tional Effective Uses, Now for Enzyme Catalysis and in Classic Organic Syntheses,” Chemical & Engineering News, Vol. 79, No. 1, 2001, pp. 21-25. doi:10.1021/cen-v079n001.p021 [14] C. Ji, K. S. Scott, G. H. Jonathan and D. R. Robin, “Poly- ethylene Glycol and Solutions of Polyethylene Glycol as Green Reaction Media” Green Chemistry, Vol. 7, No. 2, 2005, pp. 64-82. doi:10.1039/b413546f [15] S. Chandrasekhar, Ch. Narsih mulu, S. S. Sultana a nd N. R. K. Reddy, “Poly(Ethylene Glycol) (PEG) as a Reusable Solvent Medium for Organic Synthesis. Application in the Heck Reaction,” Tetrahedron Letters, Vol. 4, No. 25, 2002, pp. 4399-4401. doi:10.1021/ol0266976 [16] S. Chandrasekhar, Ch. Narsihmulu, G. Chandrasekhar and T. Shyamsunder, “Pd/CaCO3 in Liquid Poly(Ethy lene Gly- col) (PEG): An Easy and Efficient Recycle System for Partial Reduction of Alkynes to Cis-Olefins under a Hydro- gen Atmosphere,” Tetrahedron Letters, Vol. 45, No. 11, 2004, pp. 2421-2423. doi:10.1016/j.tetlet.2004.01.097 [17] S. Chandrasekhar, Ch. Narsihmulu, S. S. Sultana and N. R. K. Reddy, “Osmium Tetroxide in Poly(Ethylene Glycol) (PEG): A Recyclable Reaction Medium for Rapid Asy- mmetric Di hydroxy lation unde r Shar pless Condi tions,” Che- mical Communications, No. 14, 2003, pp. 1716-1717. doi:10.1039/b305154b [18] S. Chandrasekhar, Ch. Narsihmulu, B. Sari tha and S. S. Sul- ta na, “Poly (Ethyleneglycol) (PEG): A Rapid and Recy cla- ble Reaction Medium for the DABCO-Catalyzed Baylis- Hillman Reaction,” Tetrahedron Letters, Vol. 45, No. 30, 2004, pp. 5865-5867. doi:10.1016/j.tetlet.2004.05.153 [19] M. Xia and Y. G. Wang, “Soluble Polymer-Supported Syn- thesis of Biginelli Compounds,” Tetrahedron Letters, Vol. 43, No. 43, 2002, pp. 7703-7705. doi:10.1016/S0040-4039(02)01850-6 [20] J. H. Li, X.-C. Hu, Y. Liang and Y.-X. Xie, “PEG-400 Promoted Pd(OAc)2/DABCO-Catalyzed Cross-Coupling Reactions in Aqueous Media,” Tetrahedron Letters, Vol. 62, 2006, p. 31. [21] A. Haimov and R. Neumann, “Polyethylene Glycol as a Non-Ionic Liquid Solvent for Polyoxometalate Catalyzed Aerobic Oxidation,” Chemical Communications, No. 8, 2002, pp. 876-977. doi:10.1039/b200559j [22] S. Chandrasekhar, Ch. Narsihmulu, N. R. K. Reddy and S. S. Sultana, “Asymmetric Aldol Reactions in Poly(Ethylene Glycol) Catalyzed By L-Proline,” Tetrahedron Letters, Vol. 45, No. 23, 2004, pp. 4581-4582. doi:10.1016/j.tetlet.2004.03.116 [23] P.-Y. Lin, R.-S. Hou, H.-M. Wang, I.-J. Kang and L.-C. Chen, “Hypervalent Iodine(III) Sulfonate Mediated Syn- thesis of Quinoxalines in Liquid PEG-400,” Journal of the Chinese Chemical Society, Vol. 56, 2009, p. 683. [24] G. R. Desiraju and B. S. Goud, “Reactivity of Solids: Pre- sent, Past and Future,” Blackwell Sciences, London, 1995, p. 223. [25] V. Ramamurthy and K. Venkatesan, “Photochemical Reac- Copyright © 2011 SciRes. IJOC
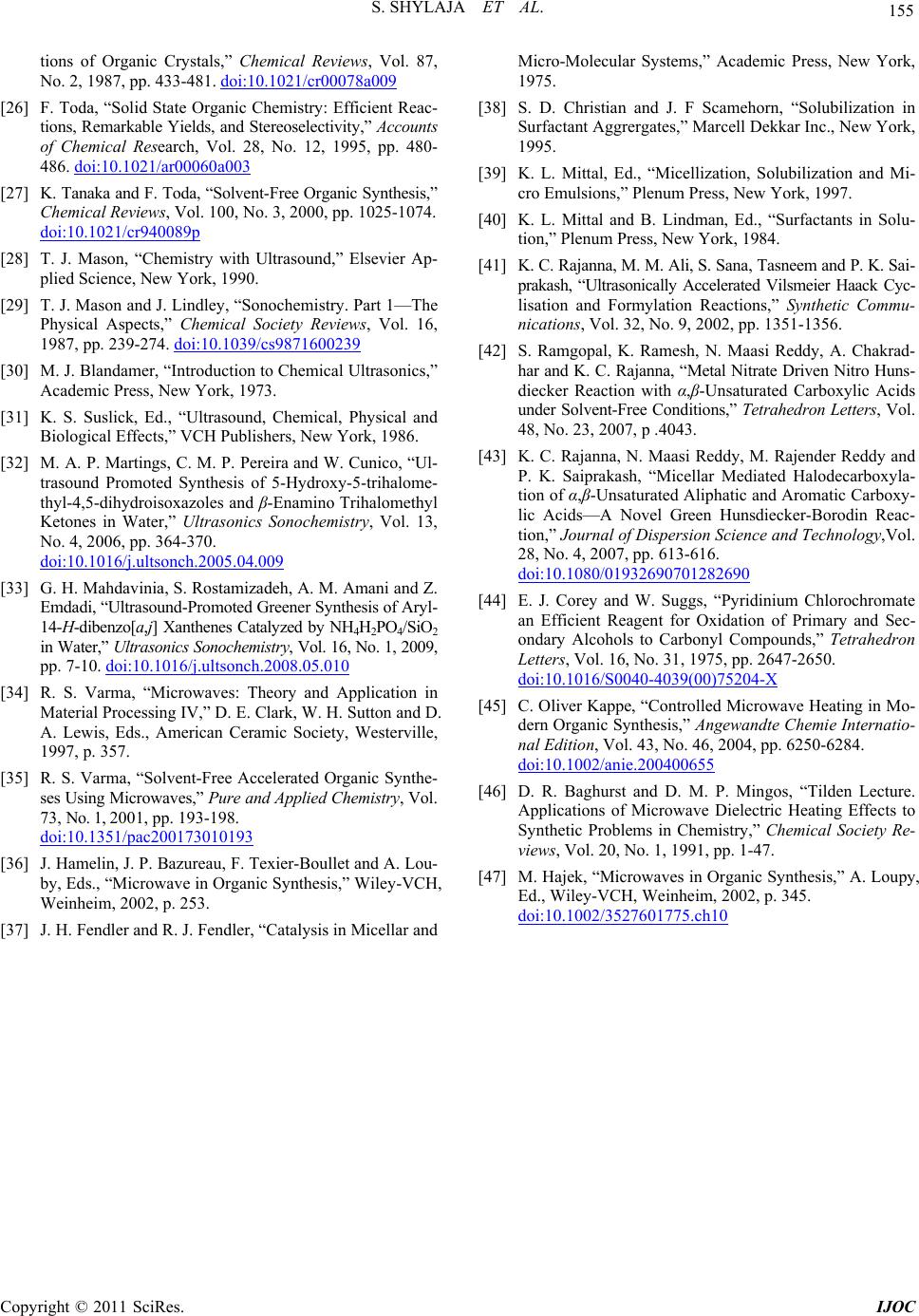 S. SHYLAJA ET AL. Copyright © 2011 SciRes. IJOC 155 tions of Organic Crystals,” Chemical Reviews, Vol. 87, No. 2, 1987, pp. 433-481. doi:10.1021/cr00078a009 [26] F. Toda, “Solid State Organic Chemistry: Effici ent Reac- tions, Remarkable Yields, and Stereoselectivity,” Accounts of Chemical Research, Vol. 28, No. 12, 1995, pp. 480- 486. doi:10.1021/ar00060a003 [27] K. Tanaka and F. Toda, “Solvent-Free Organic Synthesi s,” Chemical Reviews, Vol. 100, No. 3, 2000, pp. 1025-1074. doi:10.1021/cr940089p [28] T. J. Mason, “Chemistry with Ultrasound,” Elsevier Ap- plied Science, New York, 1990. [29] T. J. Mason and J. Lindley, “Sonochemistry. Part 1—The Physical Aspects,” Chemical Society Reviews, Vol. 16, 1987, pp. 239-274. doi:10.1039/cs9871600239 [30] M. J. Blandamer, “Introduction to Chemical Ultrasonics,” Academic Press, New York, 1973. [31] K. S. Suslick, Ed., “Ultrasound, Chemical, Physical and Biological Effects,” VCH Publishers, New York, 1986. [32] M. A. P. Martings, C. M. P. Pereira and W. Cunico, “Ul- trasound Promoted Synthesis of 5-Hydroxy-5-trihalome- thyl-4,5-dihydroisoxazoles and β-Enamino Trihalomethyl Ketones in Water,” Ultrasonics Sonochemistry, Vol. 13, No. 4, 2006, pp. 364-370. doi:10.1016/j.ultsonch.2005.04.009 [33] G. H. Mahdavinia, S. Rostamizadeh, A. M. Amani and Z. Emdadi, “Ultrasound -Promote d Greener Sy nthesis of Ary l- 14-H-dibenzo[a,j] Xanthenes Catalyzed by NH4H2PO4/SiO2 in Water,” Ultrasonics Sonochemistry, Vol. 16, No. 1, 2009, pp. 7-10. doi:10.1016/j.ultsonch.2008.05.010 [34] R. S. Varma, “Microwaves: Theory and Application in Material Processing IV,” D. E. Clark, W. H. Sutton and D. A. Lewis, Eds., American Ceramic Society, Westerville, 1997, p. 357. [35] R. S. Varma, “Solvent-Free Accelerated Organic Synthe- ses Using Microwaves,” Pure and Applied Chemistry, Vol. 73, No . 1, 2 001, pp. 193-198. doi:10.1351/pac200173010193 [36] J. Hamelin, J. P. Baz ureau, F. Texier-Boullet and A. Lou- by, Eds., “Microwave in Organic Synthesis,” Wiley-VCH, Weinheim, 2002, p. 253. [37] J. H. Fendler and R. J. Fendler, “Catalysis in Micellar and Micro-Molecular Systems,” Academic Press, New York, 1975. [38] S. D. Christian and J. F Scamehorn, “Solubilization in Surfactant Aggrergates,” Marcell Dekkar Inc., New York, 1995. [39] K. L. Mittal, Ed., “Micellization, Solubilization and Mi- cro Emulsions,” Plenum Press, New York, 1997. [40] K. L. Mittal and B. Lindman, Ed., “Surfactants in Solu- tion,” Plenum Press, New York, 1984. [41] K. C. Rajanna, M. M. Ali, S. Sana, Tasneem and P. K. Sai- prakash, “Ultrasonically Accelerated Vilsmeier Haack Cyc- lisation and Formylation Reactions,” Synthetic Commu- nications, Vol. 32, No. 9, 2002, pp. 1351-1356. [42] S. Ramgopal, K. Ramesh, N. Maasi Reddy, A. Chakrad- har and K. C. Rajanna, “Metal Nitrate Drive n Nitro Huns- diecker Reaction with α,β-Unsaturated Carboxylic Acids under Solvent-Free Conditions,” Tetrahedron Letters, Vol. 48, No. 23, 2007, p .4043. [43] K. C. Rajanna, N. Maasi Reddy, M. Rajender Reddy and P. K. Saiprakash, “Micellar Mediated Halodecarboxyla- tion of α,β-Unsaturated Aliphatic and Aromatic Carboxy- lic Acids—A Novel Green Hunsdiecker-Borodin Reac- tion,” Journal of Dispersion Science and Technology,Vol. 28, No. 4, 2007, pp. 613-616. doi:10.1080/01932690701282690 [44] E. J. Corey and W. Suggs, “Pyridinium Chlorochromate an Efficient Reagent for Oxidation of Primary and Sec- ondary Alcohols to Carbonyl Compounds,” Tetrahedron Letters, Vol. 16, No. 31, 1975, pp. 2647-2650. doi:10.1016/S0040-4039(00)75204-X [45] C. Oliver Kappe, “Controlled Microwave Heating in Mo- dern Organic Synthesis,” Angewandte Chemie Internatio- nal Edition, Vol. 43, No. 46, 2004, pp. 6250-6284. doi:10.1002/anie.200400655 [46] D. R. Baghurst and D. M. P. Mingos, “Tilden Lecture. Applications of Microwave Dielectric Heating Effects to Synthetic Problems in Chemistry,” Chemical Society Re- views, Vol. 20, No. 1, 1991, pp. 1-47. [47] M. Hajek, “Microwaves in Organic Synthesis,” A. Loupy, Ed., Wiley-VCH, Weinheim, 2002, p. 345. doi:10.1002/3527601775.ch10
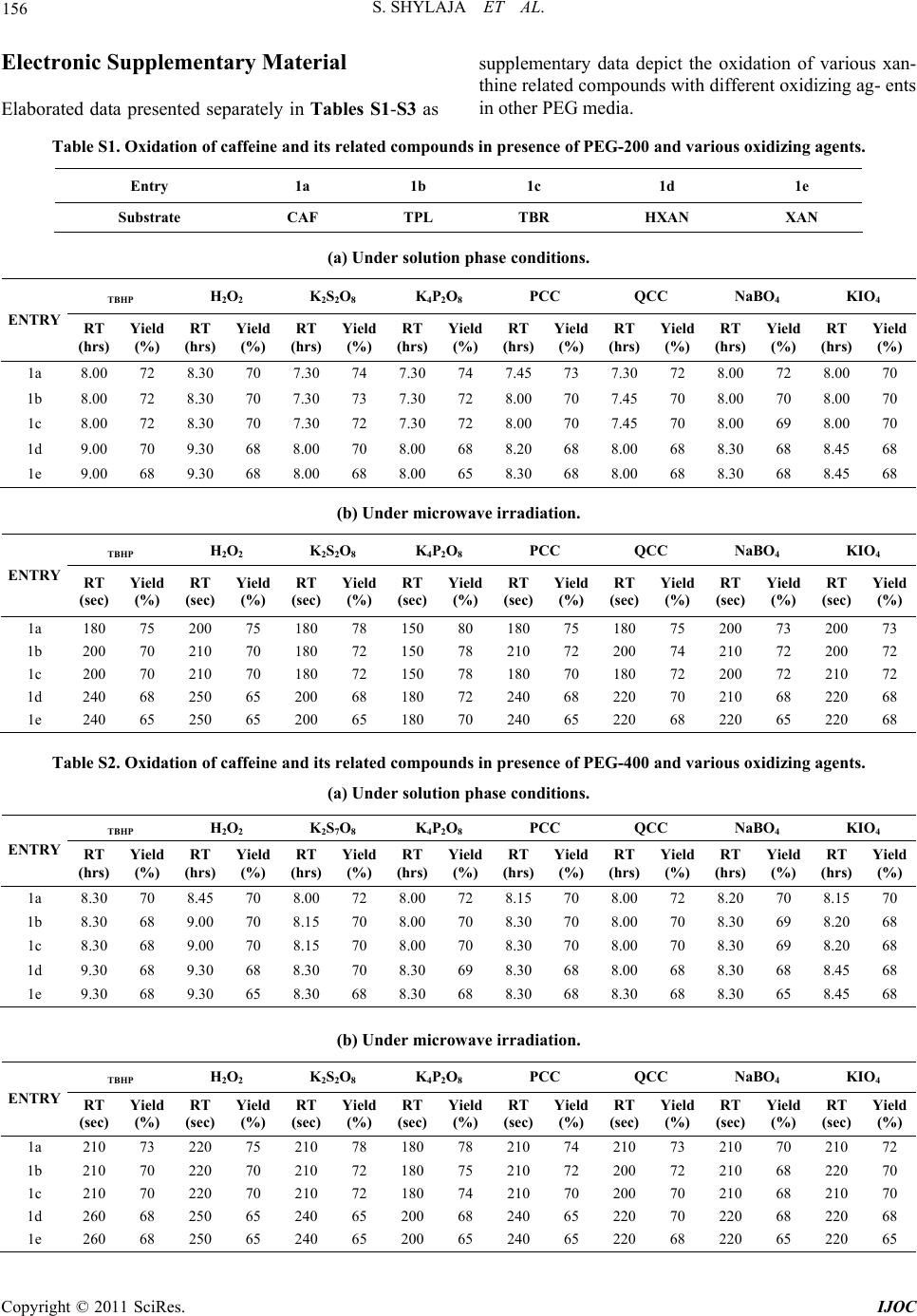 S. SHYLAJA ET AL. 156 Electronic Supplementary Material Elaborated data presented separately in Tables S1-S3 as supplementary data depict the oxidation of various xan- thine related compoun ds with d ifferent oxid izing ag - ents in other PEG media. Table S1. Oxidation of caffeine and its related compounds in presence of PEG-200 and various oxidizing agents. Entry 1a 1b 1c 1d 1e Substrate CAF TPL TBR HXAN XAN (a) Under solution phase conditions. TBHP H2O2 K 2S2O8 K 4P2O8 PCC QCC NaBO4 KIO4 ENTRY RT (hrs) Yield (%) RT (hrs) Yield (%) RT (hrs) Yield (%) RT (hrs) Yield (%) RT (hrs) Yield (%) RT (hrs) Yield (%) RT (hrs) Yield (%) RT (hrs) Yield (%) 1a 8.00 72 8.30 70 7.30 74 7.3074 7.4573 7.3072 8.00 72 8.0070 1b 8.00 72 8.30 70 7.30 73 7.3072 8.0070 7.4570 8.00 70 8.0070 1c 8.00 72 8.30 70 7.30 72 7.3072 8.0070 7.4570 8.00 69 8.0070 1d 9.00 70 9.30 68 8.00 70 8.0068 8.2068 8.0068 8.30 68 8.4568 1e 9.00 68 9.30 68 8.00 68 8.0065 8.3068 8.0068 8.30 68 8.4568 (b) Under microwave irradiation. TBHP H2O2 K 2S2O8 K 4P2O8 PCC QCC NaBO4 KIO4 ENTRY RT (sec) Yield (%) RT (sec) Yield (%) RT (sec) Yield (%) RT (sec) Yield (%) RT (sec) Yield (%) RT (sec) Yield (%) RT (sec) Yield (%) RT (sec) Yield (%) 1a 180 75 200 75 180 78 150 80 180 75 180 75 200 73 200 73 1b 200 70 210 70 180 72 150 78 210 72 200 74 210 72 200 72 1c 200 70 210 70 180 72 150 78 180 70 180 72 200 72 210 72 1d 240 68 250 65 200 68 180 72 240 68 220 70 210 68 220 68 1e 240 65 250 65 200 65 180 70 240 65 220 68 220 65 220 68 Table S2. Oxidation of caffeine and its related compounds in presence of PEG-400 and various oxidizing agents. (a) Under solution phase conditions. TBHP H2O2 K 2S7O8 K 4P2O8 PCC QCC NaBO4 KIO4 ENTRY RT (hrs) Yield (%) RT (hrs) Yield (%) RT (hrs) Yield (%) RT (hrs) Yield (%) RT (hrs) Yield (%) RT (hrs) Yield (%) RT (hrs) Yield (%) RT (hrs) Yield (%) 1a 8.30 70 8.45 70 8.00 72 8.0072 8.1570 8.0072 8.20 70 8.1570 1b 8.30 68 9.00 70 8.15 70 8.0070 8.3070 8.0070 8.30 69 8.2068 1c 8.30 68 9.00 70 8.15 70 8.0070 8.3070 8.0070 8.30 69 8.2068 1d 9.30 68 9.30 68 8.30 70 8.3069 8.3068 8.0068 8.30 68 8.4568 1e 9.30 68 9.30 65 8.30 68 8.3068 8.3068 8.3068 8.30 65 8.4568 (b) Under microwave irradiation. TBHP H2O2 K 2S2O8 K 4P2O8 PCC QCC NaBO4 KIO4 ENTRY RT (sec) Yield (%) RT (sec) Yield (%) RT (sec) Yield (%) RT (sec) Yield (%) RT (sec) Yield (%) RT (sec) Yield (%) RT (sec) Yield (%) RT (sec) Yield (%) 1a 210 73 220 75 210 78 180 78 210 74 210 73 210 70 210 72 1b 210 70 220 70 210 72 180 75 210 72 200 72 210 68 220 70 1c 210 70 220 70 210 72 180 74 210 70 200 70 210 68 210 70 1d 260 68 250 65 240 65 200 68 240 65 220 70 220 68 220 68 1e 260 68 250 65 240 65 200 65 240 65 220 68 220 65 220 65 Copyright © 2011 SciRes. IJOC
 157 S. SHYLAJA ET AL. Table S3. Oxidation of caffeine and its related compounds in presence of PEG-600 and various oxidizing agents. (a) Under solution phase conditions. TBHP H2O2 K 2S2O8 K 4P2O8 PCC QCC NaBO4 KIO4 ENTRY RT (hrs) Yield (%) RT (hrs) Yield (%) RT (hrs) Yield (%) RT (hrs) Yield (%) RT (hrs) Yield (%) RT (hrs) Yield (%) RT (hrs) Yield (%) RT (hrs) Yield (%) 1a 8.45 70 9.00 70 8.20 72 8.2072 8.3070 8.2072 8.30 70 8.3070 1b 8.45 68 9.20 70 8.30 70 8.2070 8.4570 8.2070 8.30 69 8.3068 1c 8.45 68 9.20 70 8.30 70 8.2070 8.4570 8.2070 8.30 69 8.3068 1d 9.45 68 9.40 68 8.45 70 8.4069 8.4568 8.4068 8.40 68 8.4568 1e 9.45 68 9.40 65 8.45 68 8.4068 8.4568 8.4068 8.40 65 8.4568 (b) Under microwave irradiation. TBHP H2O2 K 2S2O8 K 4P2O8 PCC QCC NaBO4 KIO4 ENTRY RT (sec) Yield (%) RT (sec) Yield (%) RT (sec) Yield (%) RT (sec) Yield (%) RT (sec) Yield (%) RT (sec) Yield (%) RT (sec) Yield (%) RT (sec) Yield (%) 1a 240 70 240 72 220 75 200 74 220 74 220 72 220 70 210 70 1b 240 70 250 68 220 70 200 72 220 70 220 70 220 68 220 70 1c 240 70 250 68 220 70 200 72 220 70 220 70 220 68 220 70 1d 280 68 280 65 260 65 220 68 250 65 220 68 240 68 220 68 1e 280 68 280 65 260 65 220 65 250 65 220 68 240 65 220 68 Copyright © 2011 SciRes. IJOC
|