Engineering
Vol. 5 No. 2 (2013) , Article ID: 27821 , 6 pages DOI:10.4236/eng.2013.52031
Effect of Clay Fines on the Behavior of Self-Compacting Concrete
Laboratory of Building in Environment, Faculty of Civil Engeenering, University of Sciences and Technology Houari Boumedienne (USTHB), Algiers, Algeria
Email: abibzineeddine@yahoo.fr
Received October 16, 2012; revised November 30, 2012; accepted December 20, 2012
Keywords: Waste Crushed Brick; Pozzolanic Activity; Heat of Hydration; Workability; Strength; Self Compacting Concrete
ABSTRACT
The technology of concrete has significantly increased in recent years through the use of superplasticizer and availability of mineral additions. One of the most recent materials used as an additive, replacing a portion of cement in concrete, is fine clay fired at a temperature of 800˚C to 900˚C. This research is based on trials that complied with artificial pozzolan (waste crushed brick), and their effect on the rheological and mechanical behavior of mortar. The addition of 5% of a waste crushed brick has helped not only to improve the strength (tensile and compression), but also to foster a better rheological behavior in terms of fluidity and stability, with a low heat of hydration compared to control. However, tests of optimizing the content of self-compacting concrete (SCC) in coarse aggregates, sand and binder, led us to confirm that the combined mass of more optimal (better workability and stability) is that based on low in volumetric percentage of sand/paste with a granular skeleton richest gravel low dimensions (2/3 of G 3/8 and 1/3 of G 8/15).
1. Introduction
Currently, the use of treated clays, particularly that are rich in SiO2 and Al2O3, is a new development in concrete technology. Many activities have been done are related to the use of clay minerals such as kaolinite, and other mixtures of clay minerals that have pozzolic activity which promote sustainable development of mechanical performance of grout, mortar or concrete with cement [1,2].
In Algeria, kaolinitic clay are mainly used for the manufacture of building materials (bricks, tiles, sanitary) as well as for the manufacture of many articles of pottery.
In general, bricks made in kilns at 800˚C contain a fairly high content of Kaolinite. Chinje Melo and all [3] have worked on three samples of tiles and bricks produced in Cameroon, they found that substitution of portion of cement type CEM I 42.5 in mortars by ground waste bricks and tiles to a diameter less than 100 µm gave pouzzolanic activity index which vary from 77% to 96% and 67% to 84% respectively for 10% and 20% of substitution.
The role of the paste (cement + water + air occluded + additives) is to remove the agglomeration of aggregates, and to reduce friction that causes the limitation towards the flowability of concrete and the ability to fill the moulds [4].
The development of self compacting concrete requires a large volume of paste (≈330 to 400 l/m3) in order to satisfy the stability of concrete [4]. Using a high content of cement in concrete may cause a significant shrinkage (result of a high heat of hydration) [5,6], which affects the durability of concrete structures. Hence it is strongly recommended to add more of a mineral additive to meet the rheological and mechanical requirements of SCC. Furthermore the uses of additives help to reduce emissions of greenhouse gases.
Fine siliceous material when added to SCC lead to similar properties of high-performance concrete (Clergue and Monachon, 1998) [7], in term of increase the compactness and thus higher strength.
In general, the criteria of fluidity-stability is quite difficult to meet, by means of using admixtures, high levels of mineral additives or appropriate levels of sand and coarse aggregates, stability and fluidity are ensured [8,9]. According to A. Tagnit-Hamou [10], adding a quantity of a mineral powder in the concrete, reduces the sweating and segregation in the concrete.
The objective of this research is to analyze, on the basis of experimental tests, the effect of adding fines fired clay (the case of crushed brick) on the rheological and mechanical properties of mortar. In this context, it is proposed to identify the nature and physico-chemistry of the fines minerals, to determine their pouzzolanicity by mechanical tests and to study the influence of the addition on the rheological behavior and mechanical mortar’s.
2. Materials
Materials used in this research are:
1) Cement CPJ CEM II/A-L 42.5 N is conform to EN 197-1 manufactured by Algerian Cement Company.
2) Three fractions of aggregates: Sand 0/3, G 3/8; G 8/15).
3) Waste crushed brick (WCB): produced by the brickyard of Bejaia, Algeria, and was ignited at 900˚C (<80 µm).
4) Silica fume (SF) produced by the Canadian company SKW.
5) Lime used in “study of pozzolanic effect” has been crushed and then sieved at 80 μm.
6) Superplasticizer (SP) Polynaphtalènes sulfone (PNS) base, with a Density: 1.22 (Color: Brown; active ingredient: 40%; PH: 7.5).
Chemical compositions of different materials are presented in Tables 1-3.
The chemical analysis of the WCB (Table 2) shows that it contains a significant percentage in silica and Alumina. Hence it is categorized as aluminosilicates.
According to Figure 1, XRD analysis confirms that amorphous phase is dominant with the presence of some crystalline elements such as quartz, calcite and dolomite.
3. Experimental Program
3.1. Evaluation of Mechanical Strength of Mortars
To assess the pozzolanic reaction of WCB, the pozzolanic activity index was calculated as follows:
Ratio between the compressive strength of cement’s mortars containing WCB and mortar without addition (Table 4).
Table 1. Chemical and mineralogical of cement content.
Table 2. Chemical analysis of waste crushed brick and silica fume.
Table 3. Mineralogical content of the WCB.
Table 4. Mortar mix proportions.
To perform the test• Waste bricks were grounded to reduce size to 80 μm sieve.
• WCB powders were used as an addition of 5% to 15% by weight of cement.
• Chemical additive’s mortar by superplasticizer at 2% of the weight of cement.
• W/C of 0.4.
• Sand fineness of 2.7 with a volume of sand/paste varies between 0.72 and 0.76.
• The mortars were cast in the form of prismatic specimens 40 × 40 × 160 mm, kept under standard conditions.
3.2. Effect of the Addition of WCB on the Rheology of Cement Grout
Poly-naphtalene sulfonate polymers are the most used in recent years. They allow until 30% of water reduction [11].
The method of disposing of Marsh cone has been used in order to verify the cement-superplasticizer’s compatibility and to determine the mix at the point of saturation.
This optimization is aimed also to assess changes in
Figure 1. X-ray diffraction pattern of WCB.
the fluidity of grout with addition of WCB after 1 h and 1 h 30 mn, this time is generally estimated for the delivery of concrete on construction sites. The trial has also highlighted the existence of critical addition ratio of superplasticizer that beyond this addition ratio no improvement of the rheology of the grout. This particular ratio is called dosing at saturation point, beyond that, we can see very quickly develop the segregation, sweating and delayed in setting.
On parallel, the rheological behavior of the grout has been compared to that of cement control (without addition).
3.3. Optimization of Contents in Coarse Aggregates, Sand, Binder
KHAYAT and YURUGI [12,13], consider that the content of coarse aggregates is one of the most important parameters in the optimization of SCC. They found that the filling capacity decreases with increasing of coarse aggregate content. Usually, we use the same volume of sand and gravel [14].
In order to optimize the granular skeleton and to select an adequate combination of coarse aggregates which improves the setting and the deformability of the self compacting concrete, we study the effect of the variation between sand (S)/paste (P), using three combination granular. It is a mass combination of:
Combination 1) with 2/3 of aggregates of fraction G 3/8 and 1/3 of G 8/15, Combination 2) with 50% of G 3/8 and 50% of G 8/15 Combination 3) with 1/3 of G 3/8 and 2/3 of G 8/15 (Table 5).
Table 5. Formulations of different variants of SCC.
We use the sieve stability test for assessment and measurement of the segregation resistance of SCC mixes [15].
3.4. Mechanical Performance
In order to study the influence of the use of WCB on the mechanical behavior of a SCC (compression and splitting tensile), three experiments has been performed (Table 6), 5% of SF, 5% of WCB and a third experiment without addition (control) with respect of the same composition SCC granular and same ratio Water/Binder (cement + additive).
4. Results and Discussion
4.1. Optimization of WCB Content
The pozzolanic activity index of mortar samples containing 10% or more of the WCB is lower than samples
Table 6. Composition of concrete (kg/m3).
without the waste (control sample). This could be explained by the slow pozzolanic reaction. Because the higher the addition percentage of cement with the pozzolan, the greater the resistance of mortars obtained.
Cements with pozzolanic additions have the advantage of providing increase of mortars resistance in the longterm (lasting mortars). This study aims to optimize the content of WCB, including the development of compression and flexural strength of these variants of mortars was monitored for 28 days of age.
According to the Figures 2 and 3, it is found that the mortar specimens with 5% of WCB have contributed to better performance in terms of compressive strength and flexural strength compared to other variants.
Overall, the pozzolanic activity index of variants are above 0.9 and the variant of the mortar at 5% of WCB has results higher than 1 during the first 28 days (Table 7).
4.2. Heat of Hydration Measurement
Following this study (Figure 4), it was found that the brick powder addition reduce heat of hydration compared to limestone addition. A decrease of 7.2% was recorded after 120 hours, whereas before 24 hours, heat of hydration is considered higher in brick powder added to clinker than the limestone, justified by the presence of aluminates.
4.3. Binder Optimization
Figure 5 shows that flow time of a cement grout to the Marsh cone decreases with the increase of the content of superplasticizer until a certain proportioning, from which the time of flow of the grout remains constant or increases slightly.
This point of intersection of the two linear parts of the curve “point of saturation”, i.e. the point from which, under the experimental conditions of measurement, any increase in proportioning while super plasticizer does not have any more significant effect on rheology of the grout. On the other hand, we notice that for a grout without WCB, the point of saturation of super plasticizer is 1.4%
Figure 3. Effect of WCB content on the compressive strength evolution of mortar.
Table 7. Pozzolanic activity index.
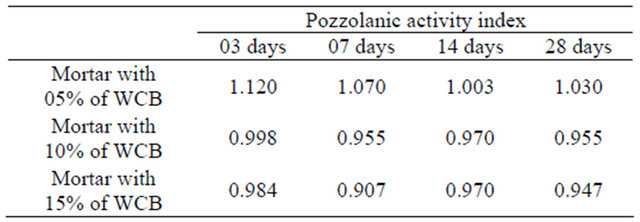
Figure 4. Effect of WCB on the heat of hydration evolution.
of the cement weight (in dry basis), the point of saturation while super plasticizer of a grout with WCB is 1.5% of the cement weight (in dry basis), with a reduction in flow time of 16% compared to that of a grout without WCB.
Figure 5. Flow time variation of a cement grout with 5% of WCB according to the superplasticizer content.
Table 8. Effect of coarse aggregates content and sand/paste ratio on the concrete rheology.
4.4. Optimization of Coarse Aggregates Content, Sand, Paste
According to Table 8, we find that the combination 1 (2/3 of G 3/8 and 1/3 of G 8/15) supported the best workability and deformability compared to the others combinations.
It is noted that for a sand/paste ratio of 0.8, combination 1 has an improvement of flowability of 2.8% and 5.9% compared to those of combinations 2 (1/2 G 3/8 and 1/2 G 8/15) and 3 (1/3 of G 3/8 and 2/3 of G 8/15) respectively.
It is observed that the reduction of the volume of coarse aggregates and consequently the increase in the volume of mortar, contribute significantly to the improvement of the workability. This improvement is mainly due to reduction of coarse aggregate content G 8/15, because the increase in the volume of the coarse aggregates especially it inhibits the workability in the case of the crushed aggregates, where friction between the aggregates is important.
Concerning the effect of the variation of S/P ratio, on the plasticity of self compacting concretes, Table 6 shows that the formulations of series C (S/P = 0.67), supported the best plasticity and deformability compared to the formulations of series A (S/P = 0.8) and B (S/P = 0.75).
Variation of the sand/paste ratio from 0.75 to 0.67 is more effective for the improvement of fluidity, in the case of the low contents in coarse aggregates. On the other side this effect is reduced if we work with a high volume of coarse aggregates, this improvement is due to the increase in the volume of the cement paste, for the series C the cement content increased 13% and 19% compared to those of the series B and A respectively.
The reduction in the content of coarse aggregates and sand/paste ratio is accompanied by an increase in the volume of mortar and paste and consequently the content of cement. This increase in the cement content leads to elevation of concrete temperature because increasing of heat of hydration; it was therefore replaced by a portion of cement with an addition WCB to overcome the disadvantage of the presence of significant cement content in concrete.
We notice according to Table 8, that the SCC with a sand/paste ratio of 0.67 supported the best stability and resistance to the segregation (2.5%) compared to those of the two others SCC, and that the SCC with S/P = 0.8 developed a critical stability and segregation rate exceeded 15%. This segregation is mainly due to the reduction in volume of paste compared to that of sand.
4.5. Mechanical Performance
The results of mechanical tests (compression and splitting tensile) carried out on SCC are indicated by the Figures 6 and 7 as below.
Various results presented in Figures 6 and 7, show that evolution of mechanical resistance to compression
Figure 6. Evolution of the compressive strength of SCC.
Figure 7. Evolution of the splitting tensile strength of SCC.
and splitting tensile strength of the SCC with WCB is similar to a SCC control (without addition).
However, the strength of SCC with silica fume has higher results than the other two variants of SCC.
5. Conclusions
The chemical analysis of the waste crushed bricks showed that it contains a significant percentage in silica and Alumina. Hence it is categorized as aluminosilicates. XRD analysis confirmed that amorphous phase is dominant with the presence of some crystalline elements such as quartz, calcite and dolomite.
Results obtained on mortars with additions of a filler from fired clay are encouraging.
First, it is noted that the use of 5% of waste crushed bricks in the mixture has strength greater than those of the reference mortar. Hydrated lime (Portlandite) formed during hydration of cement compounds reacts with the minerals additions rich in silicates and aluminates. Hydrated calcium aluminosilicates are responsible for this increase in resistance. This is the recovery of a waste product to provide a particularly powerful.
However, the addition of filler from fired clay in the composition of a self compacting concrete promotes improvement of the rheological performance (workability and stability of the mixture) and the mechanical (strength).
The reduction of sand/paste ratio from 0.8 to 0.67 achieved by the use of brick waste in the SCC composition allows obtaining the best rheological performances observed by the Abrams cone with low levels of segregation estimated by stability on sieve test.
Also, the mass composition of 2/3 of the granular fraction 3/8 and 1/3 of the granular fraction 8/15 has developed best rheological performance of SCC.
Finally, it is possible to say that the activation of white clay, with a high content of kaolinite, a cook at a temperature of 800˚C, is to form a metakaolin with properties of type pozzolanic reactivity are widely known.
This approach is currently under study and initial results are encouraging.
REFERENCES
- H. Changling, E. Makovicky and B. Osaeck, “Thermal Stability and Pozzolanic Activity of Calcined Kaolin,” Applied Clay Science, Vol. 9, No. 3, 1994, pp. 165-187.
- J. Ambroise, M. Murat and J. Pera, “Investigations on Synthetic Binders Obtained by Middle-Temperature Thermal Dissication of Clay Minerals,” Silicates Industries, Vol. 7, No. 8, 1986, pp. 99-107.
- U. Chinjemelo and N. Billong, “Activité Pouzzolanique des Déchets de Briques et Tuiles Cuite,” African Journal of Science and Technology (AJST) Science and Engineering Series, Vol. 5, No. 1, 2004, pp. 92-100.
- K. H. Hayat and K. H. Guizani, “Use of Viscosity Modifying Admixtures to Enchance Stability of Highly Fluid Concrete,” ACI Materials Journal, Vol. 94, No. 4, 1997, pp. 332-340.
- K. H. Khayat, J. Bickley and M. Lessard, “Performance of Self-Consolidating Concrete for Casting Basement and Foundation Walls,” ACI Materials Journal, Vol. 97, No. 3, 2000, pp. 374-380.
- N. A. Henderson, “Self-Compacting Concrete at Millennium Point,” Concrete, Vol. 34, No. 4, 2000, pp. 26-27.
- K. H. Khayat, “Colloques sur les Bétons Autonivelants,” Centre de Recherche Interuniversitaire sur le Béton (CRIB) Université de Sherbrooke, Universitéavall Québec, Québec, 1996.
- F. De Larrard, C. F. Ferraris and T. Sedran, “Fresh Concrete: A Hershel Bulkley Material,” Materials and Structures, Vol. 31, No. 7, 1998, pp. 494-498. doi:10.1007/BF02480474
- C. F. Ferraris and N. S. Martys, “De la Pâte de Ciment au béton: ModéLisation et Mesures Expérimentales des Propriétés Rhéologiques,” Proceedings of 36éme Colloque du Groupe Français de Rhéologie, Marne la Vallée, 10- 12 October 2001, pp. 226-230.
- A. Tagnit-Hamou, “Microstructure et Physico-Chimie des Ciments et des Bétons,” Université de Sherbrooke, Qué- bec, Janvier 1995.
- Projet National de Recherche Centre d’Études et de Recherches de l’Industrie du Béton, “Self-Compacting Concrete,’’ Epernon, October 2001.
- M. Yurugi, N. Sakata, M. Iwai and G. Sakai, “Mix Proportion for Highly Workable Concrete,” Proceedings of the International Conference of Concrete, Dundee, 7-9 September 1993, pp. 579-589.
- K. H. Khayat, “Viscosity-Enhancing Admixtures for Cement-Based Materials—An Overview,’’ Cement and Concrete Composites, Vol. 20, No. 2-3, 1998, pp. 171-188. doi:10.1016/S0958-9465(98)80006-1
- T. Sedran and F. Delarrard, “Mix Design of Self-Compacting Concrete,” Proceedings of the International RILEM Conference on Production Methods and Workability of Concrete, Paisley, June 1996, pp. 439-450.
- N. Miura, N. Takeda, R. Chikamatsu and S. Sogo, “Application of Super Workable Concrete to Reinforced Concrete Structure with Difficult Construction Conditions,” American Concrete Institute, Vol. 140, 1993, pp. 163-186.