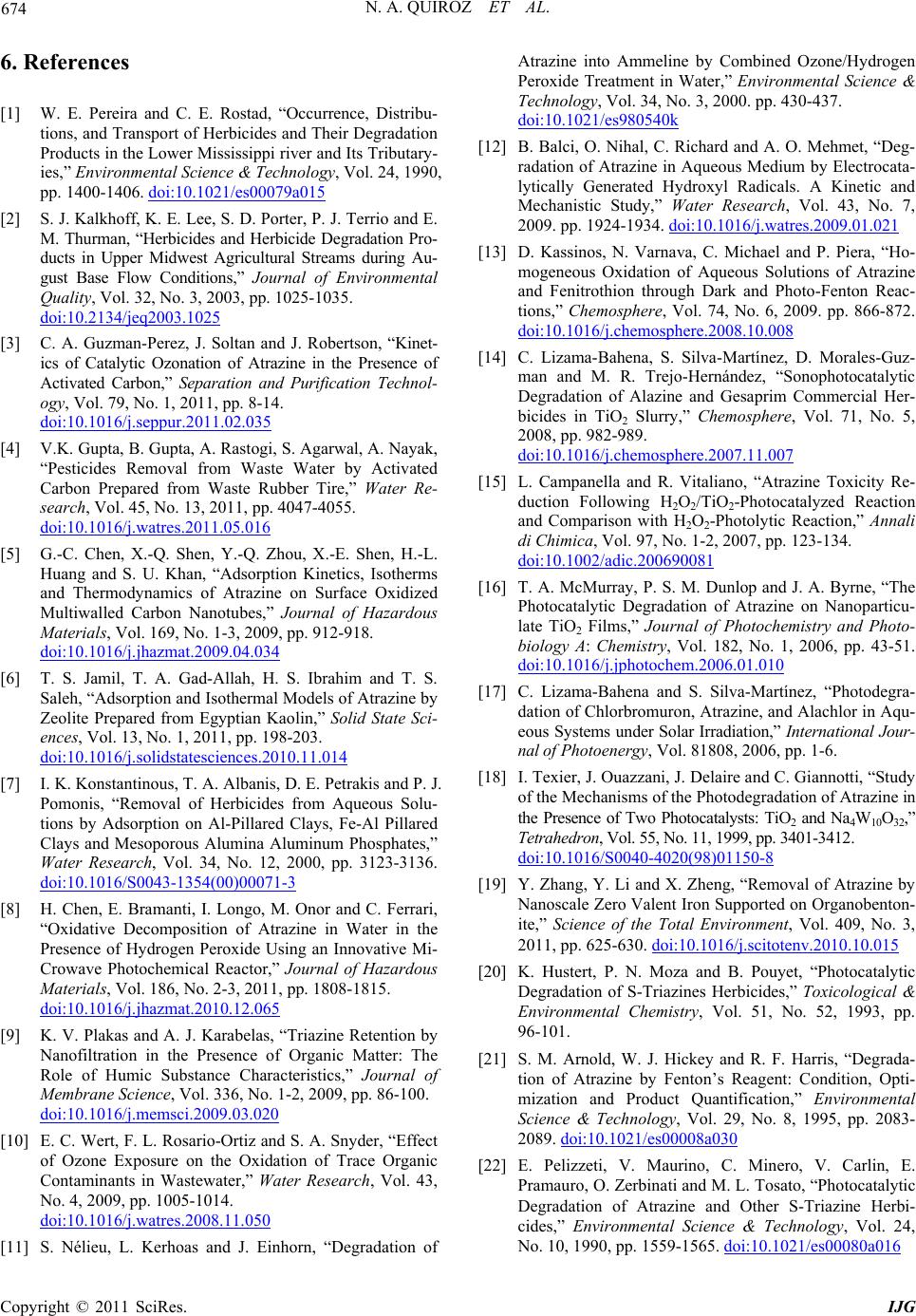
674 N. A. QUIROZ ET AL.
6. References
[1] W. E. Pereira and C. E. Rostad, “Occurrence, Distribu-
tions, and Transport of Herbicides and Their Degradation
Products in the Lower Mississippi river and Its Tributary-
ies,” Environmental Science & Technology, Vol. 24, 1990,
pp. 1400-1406. doi:10.1021/es00079a015
[2] S. J. Kalkhoff, K. E. Lee, S. D. Porter, P. J. Terrio and E.
M. Thurman, “Herbicides and Herbicide Degradation Pro-
ducts in Upper Midwest Agricultural Streams during Au-
gust Base Flow Conditions,” Journal of Environmental
Quality, Vol. 32, No. 3, 2003, pp. 1025-1035.
doi:10.2134/jeq2003.1025
[3] C. A. Guzman-Perez, J. Soltan and J. Robertson, “Kinet-
ics of Catalytic Ozonation of Atrazine in the Presence of
Activated Carbon,” Separation and Purification Technol-
ogy, Vol. 79, No. 1, 2011, pp. 8-14.
doi:10.1016/j.seppur.2011.02.035
[4] V.K. Gupta, B. Gupta, A. Rastogi, S. Agarwal, A. Nayak,
“Pesticides Removal from Waste Water by Activated
Carbon Prepared from Waste Rubber Tire,” Water Re-
search, Vol. 45, No. 13, 2011, pp. 4047-4055.
doi:10.1016/j.watres.2011.05.016
[5] G.-C. Chen, X.-Q. Shen, Y.-Q. Zhou, X.-E. Shen, H.-L.
Huang and S. U. Khan, “Adsorption Kinetics, Isotherms
and Thermodynamics of Atrazine on Surface Oxidized
Multiwalled Carbon Nanotubes,” Journal of Hazardous
Materials, Vol. 169, No. 1-3, 2009, pp. 912-918.
doi:10.1016/j.jhazmat.2009.04.034
[6] T. S. Jamil, T. A. Gad-Allah, H. S. Ibrahim and T. S.
Saleh, “Adsorption and Isothermal Models of Atrazine by
Zeolite Prepared from Egyptian Kaolin,” Solid State Sci-
ences, Vol. 13, No. 1, 2011, pp. 198-203.
doi:10.1016/j.solidstatesciences.2010.11.014
[7] I. K. Konstantinous, T. A. Albanis, D. E. Petrakis and P. J.
Pomonis, “Removal of Herbicides from Aqueous Solu-
tions by Adsorption on Al-Pillared Clays, Fe-Al Pillared
Clays and Mesoporous Alumina Aluminum Phosphates,”
Water Research, Vol. 34, No. 12, 2000, pp. 3123-3136.
doi:10.1016/S0043-1354(00)00071-3
[8] H. Chen, E. Bramanti, I. Longo, M. Onor and C. Ferrari,
“Oxidative Decomposition of Atrazine in Water in the
Presence of Hydrogen Peroxide Using an Innovative Mi-
Crowave Photochemical Reactor,” Journal of Hazardous
Materials, Vol. 186, No. 2-3, 2011, pp. 1808-1815.
doi:10.1016/j.jhazmat.2010.12.065
[9] K. V. Plakas and A. J. Karabelas, “Triazine Retention by
Nanofiltration in the Presence of Organic Matter: The
Role of Humic Substance Characteristics,” Journal of
Membrane Science, Vol. 336, No. 1-2, 2009, pp. 86-100.
doi:10.1016/j.memsci.2009.03.020
[10] E. C. Wert, F. L. Rosario-Ortiz and S. A. Snyder, “Effect
of Ozone Exposure on the Oxidation of Trace Organic
Contaminants in Wastewater,” Water Research, Vol. 43,
No. 4, 2009, pp. 1005-1014.
doi:10.1016/j.watres.2008.11.050
[11] S. Nélieu, L. Kerhoas and J. Einhorn, “Degradation of
Atrazine into Ammeline by Combined Ozone/Hydrogen
Peroxide Treatment in Water,” Environmental Science &
Technology, Vol. 34, No. 3, 2000. pp. 430-437.
doi:10.1021/es980540k
[12] B. Balci, O. Nihal, C. Richard and A. O. Mehmet, “Deg-
radation of Atrazine in Aqueous Medium by Electrocata-
lytically Generated Hydroxyl Radicals. A Kinetic and
Mechanistic Study,” Water Research, Vol. 43, No. 7,
2009. pp. 1924-1934. doi:10.1016/j.watres.2009.01.021
[13] D. Kassinos, N. Varnava, C. Michael and P. Piera, “Ho-
mogeneous Oxidation of Aqueous Solutions of Atrazine
and Fenitrothion through Dark and Photo-Fenton Reac-
tions,” Chemosphere, Vol. 74, No. 6, 2009. pp. 866-872.
doi:10.1016/j.chemosphere.2008.10.008
[14] C. Lizama-Bahena, S. Silva-Martínez, D. Morales-Guz-
man and M. R. Trejo-Hernández, “Sonophotocatalytic
Degradation of Alazine and Gesaprim Commercial Her-
bicides in TiO2 Slurry,” Chemosphere, Vol. 71, No. 5,
2008, pp. 982-989.
doi:10.1016/j.chemosphere.2007.11.007
[15] L. Campanella and R. Vitaliano, “Atrazine Toxicity Re-
duction Following H2O2/TiO2-Photocatalyzed Reaction
and Comparison with H2O2-Photolytic Reaction,” Annali
di Chimica, Vol. 97, No. 1-2, 2007, pp. 123-134.
doi:10.1002/adic.200690081
[16] T. A. McMurray, P. S. M. Dunlop and J. A. Byrne, “The
Photocatalytic Degradation of Atrazine on Nanoparticu-
late TiO2 Films,” Journal of Photochemistry and Photo-
biology A: Chemistry, Vol. 182, No. 1, 2006, pp. 43-51.
doi:10.1016/j.jphotochem.2006.01.010
[17] C. Lizama-Bahena and S. Silva-Martínez, “Photodegra-
dation of Chlorbromuron, Atrazine, and Alachlor in Aqu-
eous Systems under Solar Irradiation,” International Jour-
na l of Photoener gy, Vol. 81808, 2006, pp. 1-6.
[18] I. Texier, J. Ouazzani, J. Delaire and C. Giannotti, “Study
of the Mechanisms of the Photodegradation of Atrazine in
the Presence of Two Photocatalysts: TiO2 and Na4W10O32,”
Tetrahedron, Vol. 55, No. 11, 1999, pp. 3401-3412.
doi:10.1016/S0040-4020(98)01150-8
[19] Y. Zhang, Y. Li and X. Zheng, “Removal of Atrazine by
Nanoscale Zero Valent Iron Supported on Organobenton-
ite,” Science of the Total Environment, Vol. 409, No. 3,
2011, pp. 625-630. doi:10.1016/j.scitotenv.2010.10.015
[20] K. Hustert, P. N. Moza and B. Pouyet, “Photocatalytic
Degradation of S-Triazines Herbicides,” Toxicological &
Environmental Chemistry, Vol. 51, No. 52, 1993, pp.
96-101.
[21] S. M. Arnold, W. J. Hickey and R. F. Harris, “Degrada-
tion of Atrazine by Fenton’s Reagent: Condition, Opti-
mization and Product Quantification,” Environmental
Science & Technology, Vol. 29, No. 8, 1995, pp. 2083-
2089. doi:10.1021/es00008a030
[22] E. Pelizzeti, V. Maurino, C. Minero, V. Carlin, E.
Pramauro, O. Zerbinati and M. L. Tosato, “Photocatalytic
Degradation of Atrazine and Other S-Triazine Herbi-
cides,” Environmental Science & Technology, Vol. 24,
No. 10, 1990, pp. 1559-1565. doi:10.1021/es00080a016
C
opyright © 2011 SciRes. IJG