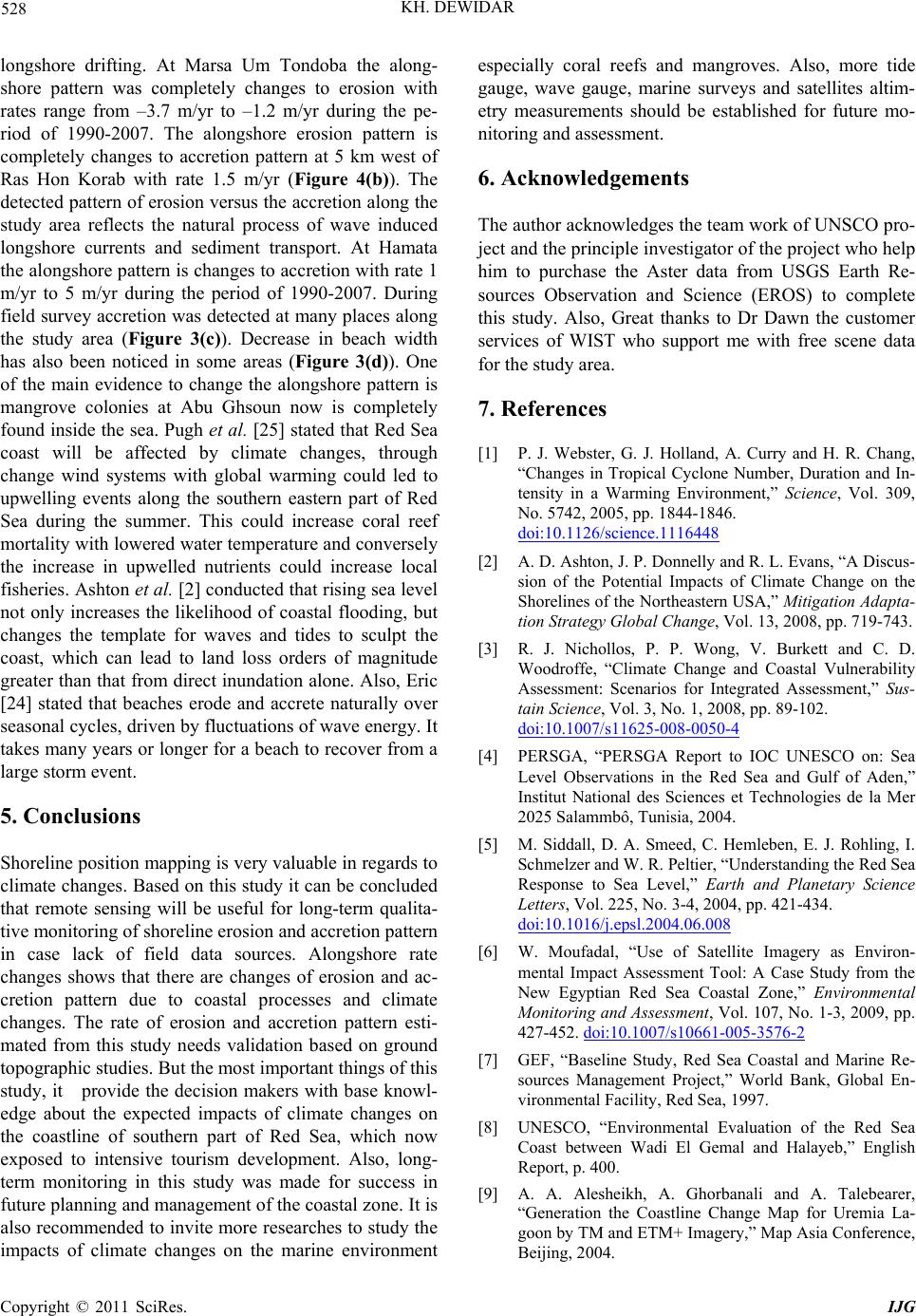
KH. DEWIDAR
528
longshore drifting. At Marsa Um Tondoba the along-
shore pattern was completely changes to erosion with
rates range from –3.7 m/yr to –1.2 m/yr during the pe-
riod of 1990-2007. The alongshore erosion pattern is
completely changes to accretion pattern at 5 km west of
Ras Hon Korab with rate 1.5 m/yr (Figure 4(b)). The
detected pattern of erosion versus the accretion along the
study area reflects the natural process of wave induced
longshore currents and sediment transport. At Hamata
the alongshore pattern is changes to accretion with rate 1
m/yr to 5 m/yr during the period of 1990-2007. During
field survey accretion was detected at many places along
the study area (Figure 3(c)). Decrease in beach width
has also been noticed in some areas (Figure 3(d)). One
of the main evidence to change the alongshore pattern is
mangrove colonies at Abu Ghsoun now is completely
found inside the sea. Pugh et al. [25] stated that Red Sea
coast will be affected by climate changes, through
change wind systems with global warming could led to
upwelling events along the southern eastern part of Red
Sea during the summer. This could increase coral reef
mortality with lowered water temperature and conversely
the increase in upwelled nutrients could increase local
fisheries. Ashton et al. [2] conducted that rising sea level
not only increases the likelihood of coastal flooding, but
changes the template for waves and tides to sculpt the
coast, which can lead to land loss orders of magnitude
greater than that from direct inundation alone. Also, Eric
[24] stated that beaches erode and accrete naturally over
seasonal cycles, driven by fluctuations of wave energy. It
takes many years or longer for a beach to recover from a
large storm event.
5. Conclusions
Shoreline position mapping is very valuable in regards to
climate changes. Based on this study it can be concluded
that remote sensing will be useful for long-term qualita-
tive monitoring of shoreline erosion and accretion pattern
in case lack of field data sources. Alongshore rate
changes shows that there are changes of erosion and ac-
cretion pattern due to coastal processes and climate
changes. The rate of erosion and accretion pattern esti-
mated from this study needs validation based on ground
topographic studies. But the most important things of this
study, it provide the decision makers with base knowl-
edge about the expected impacts of climate changes on
the coastline of southern part of Red Sea, which now
exposed to intensive tourism development. Also, long-
term monitoring in this study was made for success in
future planning and management of the coastal zone. It is
also recommended to invite more researches to study the
impacts of climate changes on the marine environment
especially coral reefs and mangroves. Also, more tide
gauge, wave gauge, marine surveys and satellites altim-
etry measurements should be established for future mo-
nitoring and assessment.
6. Acknowledgements
The author acknowledges the team work of UNSCO pro-
ject and the principle investigator of the project who help
him to purchase the Aster data from USGS Earth Re-
sources Observation and Science (EROS) to complete
this study. Also, Great thanks to Dr Dawn the customer
services of WIST who support me with free scene data
for the study area.
7. References
[1] P. J. Webster, G. J. Holland, A. Curry and H. R. Chang,
“Changes in Tropical Cyclone Number, Duration and In-
tensity in a Warming Environment,” Science, Vol. 309,
No. 5742, 2005, pp. 1844-1846.
doi:10.1126/science.1116448
[2] A. D. Ashton, J. P. Donnelly and R. L. Evans, “A Discus-
sion of the Potential Impacts of Climate Change on the
Shorelines of the Northeastern USA,” Mitigation Adapta-
tion Strategy Global Change, Vol. 13, 2008, pp. 719-743.
[3] R. J. Nichollos, P. P. Wong, V. Burkett and C. D.
Woodroffe, “Climate Change and Coastal Vulnerability
Assessment: Scenarios for Integrated Assessment,” Sus-
tain Science, Vol. 3, No. 1, 2008, pp. 89-102.
doi:10.1007/s11625-008-0050-4
[4] PERSGA, “PERSGA Report to IOC UNESCO on: Sea
Level Observations in the Red Sea and Gulf of Aden,”
Institut National des Sciences et Technologies de la Mer
2025 Salammbô, Tunisia, 2004.
[5] M. Siddall, D. A. Smeed, C. Hemleben, E. J. Rohling, I.
Schmelzer and W. R. Peltier, “Understanding the Red Sea
Response to Sea Level,” Earth and Planetary Science
Letters, Vol. 225, No. 3-4, 2004, pp. 421-434.
doi:10.1016/j.epsl.2004.06.008
[6] W. Moufadal, “Use of Satellite Imagery as Environ-
mental Impact Assessment Tool: A Case Study from the
New Egyptian Red Sea Coastal Zone,” Environmental
Monitoring and Assessment, Vol. 107, No. 1-3, 2009, pp.
427-452. doi:10.1007/s10661-005-3576-2
[7] GEF, “Baseline Study, Red Sea Coastal and Marine Re-
sources Management Project,” World Bank, Global En-
vironmental Facility, Red Sea, 1997.
[8] UNESCO, “Environmental Evaluation of the Red Sea
Coast between Wadi El Gemal and Halayeb,” English
Report, p. 400.
[9] A. A. Alesheikh, A. Ghorbanali and A. Talebearer,
“Generation the Coastline Change Map for Uremia La-
goon by TM and ETM+ Imagery,” Map Asia Conference,
Beijing, 2004.
Copyright © 2011 SciRes. IJG