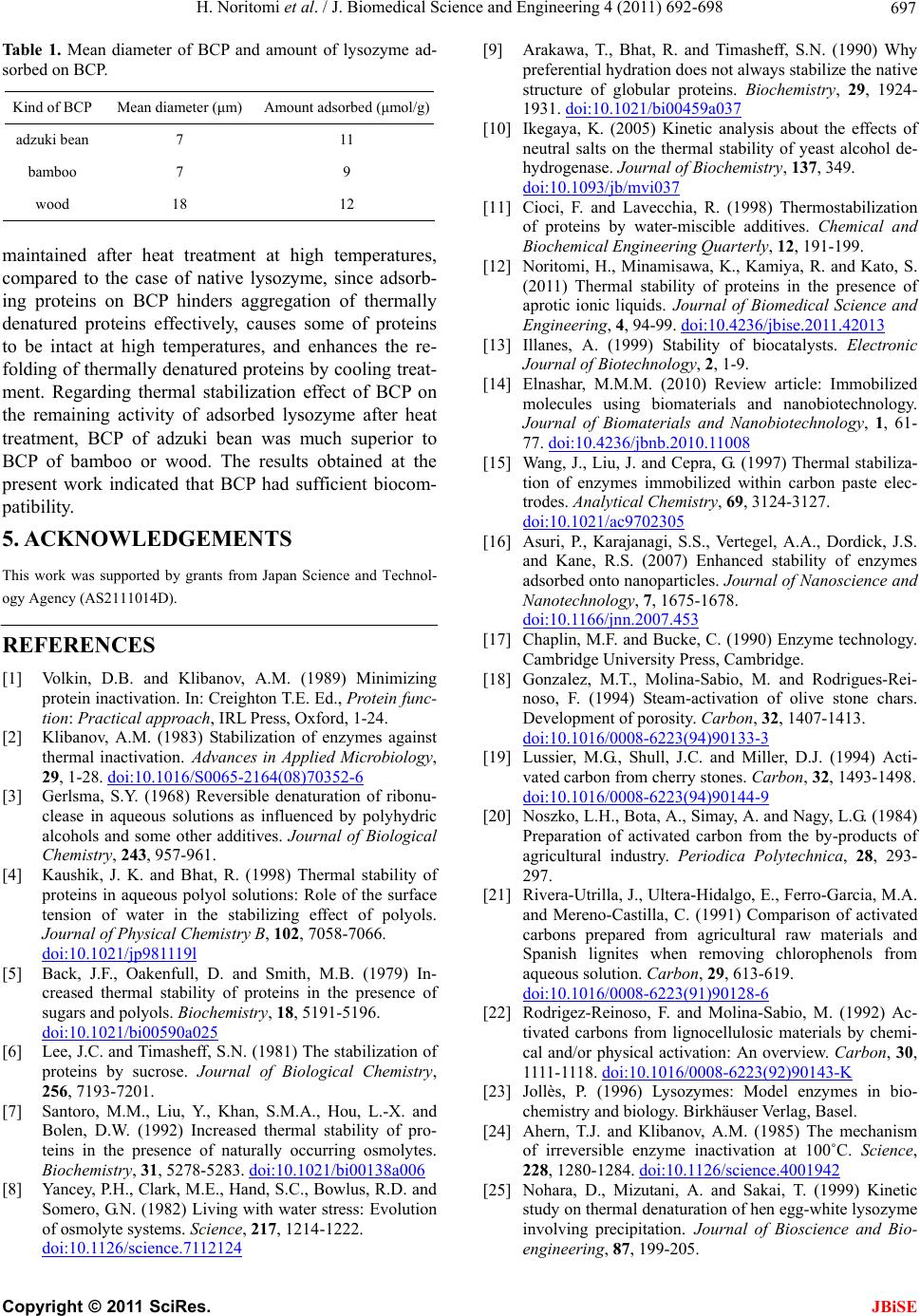
H. Noritomi et al. / J. Biomedical Science and Engineering 4 (2011) 692-698 697
Ta b le 1 . Mean diameter of BCP and amount of lysozyme ad-
sorbed on BCP.
Kind of BCP Mean diameter (μm) Amount adsorbed (μmol/g)
adzuki bean 7 11
bamboo 7 9
wood 18 12
maintained after heat treatment at high temperatures,
compared to the case of native lysozyme, since adsorb-
ing proteins on BCP hinders aggregation of thermally
denatured proteins effectively, causes some of proteins
to be intact at high temperatures, and enhances the re-
folding of thermally denatured proteins by cooling treat-
ment. Regarding thermal stabilization effect of BCP on
the remaining activity of adsorbed lysozyme after heat
treatment, BCP of adzuki bean was much superior to
BCP of bamboo or wood. The results obtained at the
present work indicated that BCP had sufficient biocom-
patibility.
5. ACKNOWLEDGEMENTS
This work was supported by grants from Japan Science and Technol-
ogy Agency (AS2111014D).
REFERENCES
[1] Volkin, D.B. and Klibanov, A.M. (1989) Minimizing
protein inactivation. In: Creighton T.E. Ed., Protein func-
tion: Practical approach, IRL Press, Oxford, 1-24.
[2] Klibanov, A.M. (1983) Stabilization of enzymes against
thermal inactivation. Advances in Applied Microbiology,
29, 1-28. doi:10.1016/S0065-2164(08)70352-6
[3] Gerlsma, S.Y. (1968) Reversible denaturation of ribonu-
clease in aqueous solutions as influenced by polyhydric
alcohols and some other additives. Journal of Biological
Chemistry, 243, 957-961.
[4] Kaushik, J. K. and Bhat, R. (1998) Thermal stability of
proteins in aqueous polyol solutions: Role of the surface
tension of water in the stabilizing effect of polyols.
Journal of Physical Chemistry B, 102, 7058-7066.
doi:10.1021/jp981119l
[5] Back, J.F., Oakenfull, D. and Smith, M.B. (1979) In-
creased thermal stability of proteins in the presence of
sugars and polyols. Biochemistry, 18, 5191-5196.
doi:10.1021/bi00590a025
[6] Lee, J.C. and Timasheff, S.N. (1981) The stabilization of
proteins by sucrose. Journal of Biological Chemistry,
256, 7193-7201.
[7] Santoro, M.M., Liu, Y., Khan, S.M.A., Hou, L.-X. and
Bolen, D.W. (1992) Increased thermal stability of pro-
teins in the presence of naturally occurring osmolytes.
Biochemistry, 31, 5278-5283. doi:10.1021/bi00138a006
[8] Yancey, P.H., Clark, M.E., Hand, S.C., Bowlus, R.D. and
Somero, G.N. (1982) Living with water stress: Evolution
of osmolyte systems. Science, 217, 1214-1222.
doi:10.1126/science.7112124
[9] Arakawa, T., Bhat, R. and Timasheff, S.N. (1990) Why
preferential hydration does not always stabilize the native
structure of globular proteins. Biochemistry, 29, 1924-
1931. doi:10.1021/bi00459a037
[10] Ikegaya, K. (2005) Kinetic analysis about the effects of
neutral salts on the thermal stability of yeast alcohol de-
hydrogenase. Journal of Biochemistry, 137, 349.
doi:10.1093/jb/mvi037
[11] Cioci, F. and Lavecchia, R. (1998) Thermostabilization
of proteins by water-miscible additives. Chemical and
Biochemical Engineering Quarterly, 12, 191-199.
[12] Noritomi, H., Minamisawa, K., Kamiya, R. and Kato, S.
(2011) Thermal stability of proteins in the presence of
aprotic ionic liquids. Journal of Biomedical Science and
Engineering, 4, 94-99. doi:10.4236/jbise.2011.42013
[13] Illanes, A. (1999) Stability of biocatalysts. Electronic
Journal of Biotechnology, 2, 1-9.
[14] Elnashar, M.M.M. (2010) Review article: Immobilized
molecules using biomaterials and nanobiotechnology.
Journal of Biomaterials and Nanobiotechnology, 1, 61-
77. doi:10.4236/jbnb.2010.11008
[15] Wang, J., Liu, J. and Cepra, G. (1997) Thermal stabiliza-
tion of enzymes immobilized within carbon paste elec-
trodes. Analytical Chemistry, 69, 3124-3127.
doi:10.1021/ac9702305
[16] Asuri, P., Karajanagi, S.S., Vertegel, A.A., Dordick, J.S.
and Kane, R.S. (2007) Enhanced stability of enzymes
adsorbed onto nanoparticles. Journal of Nanoscience and
Nanotechnology, 7, 1675-1678.
doi:10.1166/jnn.2007.453
[17] Chaplin, M.F. and Bucke, C. (1990) Enzyme technology.
Cambridge University Press, Cambridge.
[18] Gonzalez, M.T., Molina-Sabio, M. and Rodrigues-Rei-
noso, F. (1994) Steam-activation of olive stone chars.
Development of porosity. Carbon, 32, 1407-1413.
doi:10.1016/0008-6223(94)90133-3
[19] Lussier, M.G., Shull, J.C. and Miller, D.J. (1994) Acti-
vated carbon from cherry stones. Carbon, 32, 1493-1498.
doi:10.1016/0008-6223(94)90144-9
[20] Noszko, L.H., Bota, A., Simay, A. and Nagy, L.G. (1984)
Preparation of activated carbon from the by-products of
agricultural industry. Periodica Polytechnica, 28, 293-
297.
[21] Rivera-Utrilla, J., Ultera-Hidalgo, E., Ferro-Garcia, M.A.
and Mereno-Castilla, C. (1991) Comparison of activated
carbons prepared from agricultural raw materials and
Spanish lignites when removing chlorophenols from
aqueous solution. Carbon, 29, 613-619.
doi:10.1016/0008-6223(91)90128-6
[22] Rodrigez-Reinoso, F. and Molina-Sabio, M. (1992) Ac-
tivated carbons from lignocellulosic materials by chemi-
cal and/or physical activation: An overview. Carbon, 30,
1111-1118. doi:10.1016/0008-6223(92)90143-K
[23] Jollès, P. (1996) Lysozymes: Model enzymes in bio-
chemistry and biology. Birkhäuser Verlag, Basel.
[24] Ahern, T.J. and Klibanov, A.M. (1985) The mechanism
of irreversible enzyme inactivation at 100˚C. Science,
228, 1280-1284. doi:10.1126/science.4001942
[25] Nohara, D., Mizutani, A. and Sakai, T. (1999) Kinetic
study on thermal denaturation of hen egg-white lysozyme
involving precipitation. Journal of Bioscience and Bio-
engineering, 87, 199-205.
C
opyright © 2011 SciRes. JBiSE