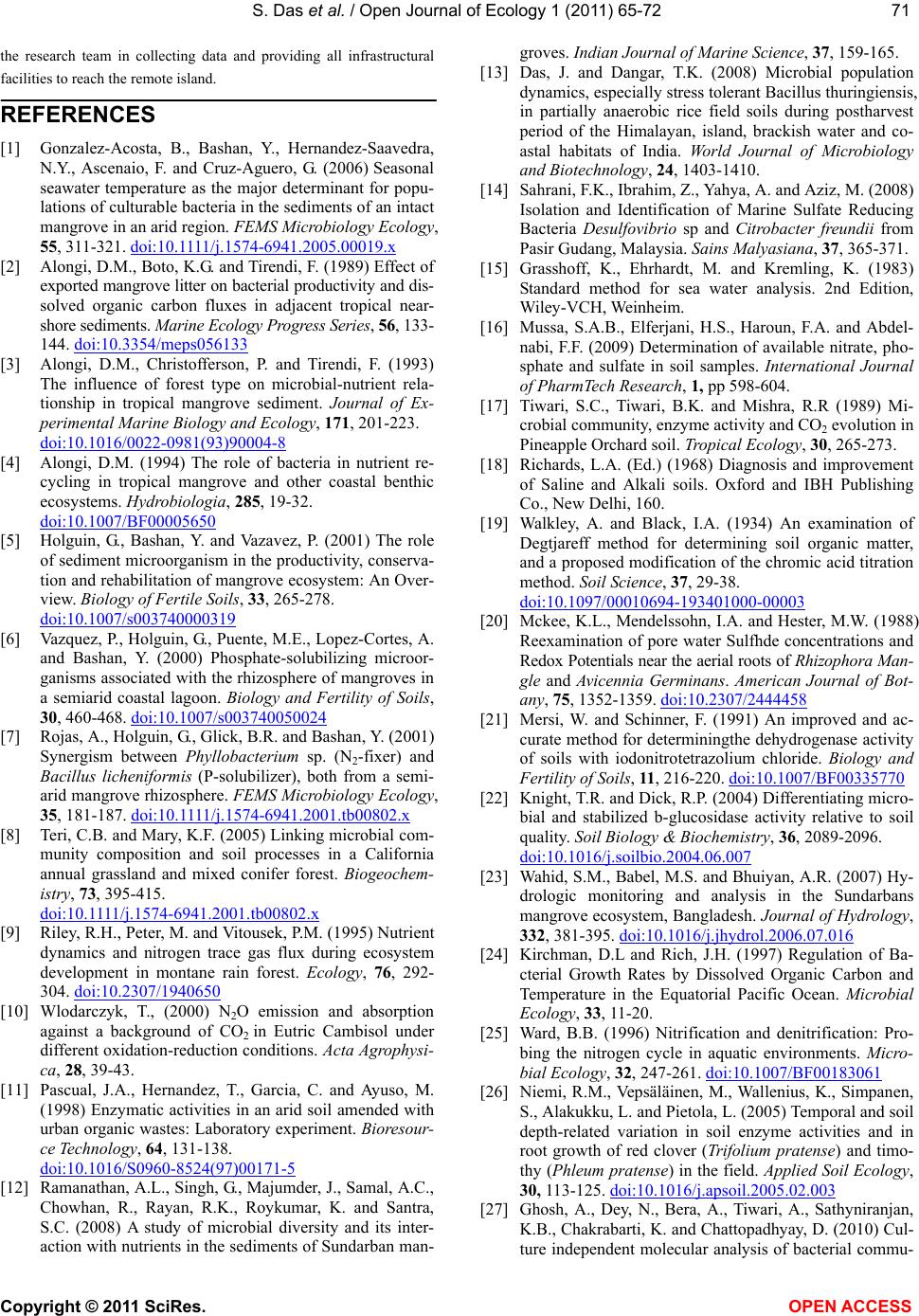
S. Das et al. / Open Journal of Ecology 1 (2011) 65-72
Copyright © 2011 SciRes. OPEN ACCESS
7171
the research team in collecting data and providing all infrastructural
facilities to reach the remote island.
REFERENCES
[1] Gonzalez-Acosta, B., Bashan, Y., Hernandez-Saavedra,
N.Y., Ascenaio, F. and Cruz-Aguero, G. (2006) Seasonal
seawater temperature as the major determinant for popu-
lations of culturable bacteria in the sediments of an intact
mangrove in an arid region. FEMS Microbiology Ecology,
55, 311-321. doi:10.1111/j.1574-6941.2005.00019.x
[2] Alongi, D.M., Boto, K.G. and Tirendi, F. (1989) Effect of
exported mangrove litter on bacterial productivity and dis-
solved organic carbon fluxes in adjacent tropical near-
shore sediments. Marine Ecology Progress Series, 56, 133-
144. doi:10.3354/meps056133
[3] Alongi, D.M., Christofferson, P. and Tirendi, F. (1993)
The influence of forest type on microbial-nutrient rela-
tionship in tropical mangrove sediment. Journal of Ex-
perimental Marine Biology and Ecology, 171, 201-223.
doi:10.1016/0022-0981(93)90004-8
[4] Alongi, D.M. (1994) The role of bacteria in nutrient re-
cycling in tropical mangrove and other coastal benthic
ecosystems. Hydrobiologia, 285, 19-32.
doi:10.1007/BF00005650
[5] Holguin, G., Bashan, Y. and Vazavez, P. (2001) The role
of sediment microorganism in the productivity, conserva-
tion and rehabilitation of mangrove ecosystem: An Over-
view. Biology of Fertile Soils, 33, 265-278.
doi:10.1007/s003740000319
[6] Vazquez, P., Holguin, G., Puente, M.E., Lopez-Cortes, A.
and Bashan, Y. (2000) Phosphate-solubilizing microor-
ganisms associated with the rhizosphere of mangroves in
a semiarid coastal lagoon. Biology and Fertility of Soils,
30, 460-468. doi:10.1007/s003740050024
[7] Rojas, A., Holguin, G., Glick, B.R. and Bashan, Y. (2001)
Synergism between Phyllobacterium sp. (N2-fixer) and
Bacillus licheniformis (P-solubilizer), both from a semi-
arid mangrove rhizosphere. FEMS Microbiology Ecology,
35, 181-187. doi:10.1111/j.1574-6941.2001.tb00802.x
[8] Teri, C.B. and Mary, K.F. (2005) Linking microbial com-
munity composition and soil processes in a California
annual grassland and mixed conifer forest. Biogeochem-
istry, 73, 395-415.
doi:10.1111/j.1574-6941.2001.tb00802.x
[9] Riley, R.H., Peter, M. and Vitousek, P.M. (1995) Nutrient
dynamics and nitrogen trace gas flux during ecosystem
development in montane rain forest. Ecology, 76, 292-
304. doi:10.2307/1940650
[10] Wlodarczyk, T., (2000) N2O emission and absorption
against a background of CO2 in Eutric Cambisol under
different oxidation-reduction conditions. Acta Agrophysi-
ca, 28, 39-43.
[11] Pascual, J.A., Hernandez, T., Garcia, C. and Ayuso, M.
(1998) Enzymatic activities in an arid soil amended with
urban organic wastes: Laboratory experiment. Bioresour-
ce Technology, 64, 131-138.
doi:10.1016/S0960-8524(97)00171-5
[12] Ramanathan, A.L., Singh, G., Majumder, J., Samal, A.C.,
Chowhan, R., Rayan, R.K., Roykumar, K. and Santra,
S.C. (2008) A study of microbial diversity and its inter-
action with nutrients in the sediments of Sundarban man-
groves. Indian Journal of Marine Science, 37, 159-165.
[13] Das, J. and Dangar, T.K. (2008) Microbial population
dynamics, especially stress tolerant Bacillus thuringiensis,
in partially anaerobic rice field soils during postharvest
period of the Himalayan, island, brackish water and co-
astal habitats of India. World Journal of Microbiology
and Biotechnology, 24, 1403-1410.
[14] Sahrani, F.K., Ibrahim, Z., Yahya, A. and Aziz, M. (2008)
Isolation and Identification of Marine Sulfate Reducing
Bacteria Desulfovibrio sp and Citrobacter freundii from
Pasir Gudang, Malaysia. Sains Malyasiana, 37, 365-371.
[15] Grasshoff, K., Ehrhardt, M. and Kremling, K. (1983)
Standard method for sea water analysis. 2nd Edition,
Wiley-VCH, Weinheim.
[16] Mussa, S.A.B., Elferjani, H.S., Haroun, F.A. and Abdel-
nabi, F.F. (2009) Determination of available nitrate, pho-
sphate and sulfate in soil samples. International Journal
of PharmTech Research, 1, pp 598-604.
[17] Tiwari, S.C., Tiwari, B.K. and Mishra, R.R (1989) Mi-
crobial community, enzyme activity and CO2 evolution in
Pineapple Orchard soil. Tropical Ecology, 30, 265-273.
[18] Richards, L.A. (Ed.) (1968) Diagnosis and improvement
of Saline and Alkali soils. Oxford and IBH Publishing
Co., New Delhi, 160.
[19] Walkley, A. and Black, I.A. (1934) An examination of
Degtjareff method for determining soil organic matter,
and a proposed modification of the chromic acid titration
method. Soil Science, 37, 29-38.
doi:10.1097/00010694-193401000-00003
[20] Mckee, K.L., Mendelssohn, I.A. and Hester, M.W. (1988)
Reexamination of pore water Sulfhde concentrations and
Redox Potentials near the aerial roots of Rhizophora Man-
gle and Avicennia Germinans. American Journal of Bot-
any, 75, 1352-1359. doi:10.2307/2444458
[21] Mersi, W. and Schinner, F. (1991) An improved and ac-
curate method for determiningthe dehydrogenase activity
of soils with iodonitrotetrazolium chloride. Biology and
Fertility of Soils, 11, 216-220. doi:10.1007/BF00335770
[22] Knight, T.R. and Dick, R.P. (2004) Differentiating micro-
bial and stabilized b-glucosidase activity relative to soil
quality. Soil Biology & Biochemistry, 36, 2089-2096.
doi:10.1016/j.soilbio.2004.06.007
[23] Wahid, S.M., Babel, M.S. and Bhuiyan, A.R. (2007) Hy-
drologic monitoring and analysis in the Sundarbans
mangrove ecosystem, Bangladesh. Journal of Hydrology,
332, 381-395. doi:10.1016/j.jhydrol.2006.07.016
[24] Kirchman, D.L and Rich, J.H. (1997) Regulation of Ba-
cterial Growth Rates by Dissolved Organic Carbon and
Temperature in the Equatorial Pacific Ocean. Microbial
Ecology, 33, 11-20.
[25] Ward, B.B. (1996) Nitrification and denitrification: Pro-
bing the nitrogen cycle in aquatic environments. Micro-
bial Ecology, 32, 247-261. doi:10.1007/BF00183061
[26] Niemi, R.M., Vepsäläinen, M., Wallenius, K., Simpanen,
S., Alakukku, L. and Pietola, L. (2005) Temporal and soil
depth-related variation in soil enzyme activities and in
root growth of red clover (Trifolium pratense) and timo-
thy (Phleum pratense) in the field. Applied Soil Ecology,
30, 113-125. doi:10.1016/j.apsoil.2005.02.003
[27] Ghosh, A., Dey, N., Bera, A., Tiwari, A., Sathyniranjan,
K.B., Chakrabarti, K. and Chattopadhyay, D. (2010) Cul-
ture independent molecular analysis of bacterial commu-