 Advances in Biological Chemistry, 2011, 1, 49-57 doi:10.4236/abc.2011.13007 Published Online November 2011 (http://www.SciRP.org/journal/abc/ ABC ). Published Online November 2011 in SciRes. http://www.scirp.org/journal/ABC Gentian extract induces caspase-independent and mitochondria-modulated cell death Mika Ogata1, Kazushige Matsukawa2, Kiyomi Kogusuri1, Tetsur o Yamashita2, Takashi Hikage2,3, Kikukatsu Ito1,2, Yasushi Saitoh1,2, Ken-ichi Tsutsu mi 1,2* 1Cryobiofrontier Research Center, Iwate University, Morioka, Japan; 2United Graduate School of Agricultural Sciences, Iwate University, Morioka, Japan; 3Hachimantai City Floricultural Research and Development Center, Hachimantai, Japan. Email: *kentsu@iwate-u.ac.jp Received 23 September 2011; revised 25 October 2011; accepted 3 November 2011. ABSTRACT Extracts from the dried roots of gentian plant, Gen- tiana triflora, exhibit an antiproliferative activity a- gainst cultured and implanted tumor cells. However, the underlying mechanism has been unclear. In the present study, we show that the cell death induced by the extract occurs caspase-independently and de- pends on metabolic status of mitochondrial respira- tion. We observed that sensitivity to the extract was considerably lower in HeLa cells, which have a low rate of mitochondrial respiration, in comparison to Y3-Ag1.2.3 cells, which have a higher rate of respira- tion. Furthermore, sensitivity of HeLa cells to the extract increased significantly when they were forced to switch their energy dependency from glycolysis to mitochondrial respiration. These results indicate that the gentian extract targets on mitochondrial respira- tion. Consequently, different respiratory activities in mitochondria confer cells to have different suscepti- bilities to the extract-induced cell death. Keywords: Antiproliferative Activity; Cell Death; Mi- tochondria; Respiration; Gentiana triflora 1. INTRODUCTION As a typical form of cell death, apoptosis has been ex- tensively characterized. However, reports concerning to additional types of cell death are accumulating, and the pathways for cell death are now thought to be far more diverse than predicted [1-3]. Despite such diverse path- ways, the mitochondria act in many cases as a relay point that transmits different signaling for cell death, with the final outcome of the mitoch o ndria depend ing on the cell types. Accordingly, the metabolic condition of the mitochondria modulates both apoptotic [4-7] and non-apoptotic cell death [8-12]. Highly proliferative can- cer cells derive most their energy from glycolysis ra- ther than from mitochondrial respiration [13-19]. Those cancer cells are adapted for survival by using various pathways to avoid cell death [20,21]. Upregulated gly- colysis, for example, activates the serine/threonine ki- nase Akt, conferring resistance to antiproliferative drugs [6,22]. Susceptibility of cells to antipro liferative drugs is also modulated by mitochondrial respiration. A high con- centration of glucose in culture strongly inhibits mito- chondrial respiration in most cancer cells, a phenomenon called the Crabtree effect [23]. Those cells exhibit resis- tance to mitochondrial toxicants such as antimycin and oligomycin. Circumventing the Crabtree effect by grow- ing in glucose-deprivated galactose media [24,25], cells become susceptible to the toxicants [26]. Collectively, glycolysis and mitochondrial respiration are directly or indirectly involved in cell death and survival signaling. Previously, we reported that extracts from dried roots of Gentiana triflora, an ingred ient in Chinese herbal me- dicine [27], exhibit cell death-inducing activity against cultured tumor cells and antiproliferative activity agains t implanted tumor cells [28]. Th e underlining mechanism, however, has been unknown. Here, we show that the ex- tract-induced cell death occurs caspase-independently and depends on mitochondrial state of energy metabo- lism. 2. MATERIALS AND METHODS 2.1. Plant Materials and Extract Preparation The gentian plant used in this study was Gentiana tri- flora var. japonica, which was provided by the Hachi- mantai City Floricultural Research and Development Center, Hachimantai, Iwate 028-7592, Japan. Prepara- tion of gentian root extract and its cell antiproliferative activity has been described previously [28]. Dried roots were powdered and extracted sequentially with 10 times volume (w/v) of chloroform at 50˚C, methanol at 50˚C,
 M. Ogata et al. / Advances in Biological Chemistry 1 (2011) 49-57 50 and boiling water for 30 min. Each extraction was re- peated twice. After cooling, the extract was vacuum- dried, dissolved in methanol or water (for boiling-water extract) at a concentration of 20 mg/ml, and centrifuged at 14.000 ×g for 30 min to discard insoluble fibrou s ma- terials. The boiling-water extract was autoclaved for 20 min at 121˚C, and passed through a 0.22 mm filter. The extracts were stored at 4˚C in the dark. 2.2. Cells and Culture Conditions Y3-Ag1.2.3 cells derived from rat myeloma were pur- chased from Health Science Research Resources Bank (JCRB9091, Osaka, Japan) and were cultured in Dul- becco’s modified Eagle’s medium (DMEM) containing 25 mM glucose and supplemented with 5% fetal bovine serum (FBS). Human HeLa and rat dRLh84 cells were cultured in DMEM supplemented with 10% FBS and newborn bovine serum (NBS), respectively. Daudi and 3Y1-B1-6 cells were cultured in DMEM supplemented with 5% FBS. For culturing HeLa and Y3-Ag1.2.3 cells in galactose, the glucose in each medium was replaced by 10 mM galactose and supplemented with 1 mM so- dium pyruvate [18]. Cells were maintained in the galac- tose medium for more than 10 passages prior to the ex- periments. 2.3. Cell Proliferation Assay Cell proliferation was measured either by counting vi- able cells with a trypan blue exclusion assay, or by a WST-1 (4-[3-(4-iodophenyl)-2-(4-nitrophenyl)-2H-5-tetra- zolio]-1, 3-benzene disulfonate) assay according to the supplier’s protocol (Cell Counting Kit, DOJINDO, Ja- pan). Briefly, cells (1 to 2 × 104) were seeded in 100 ml of medium in a 96-well plate in the presence or absence of gentian root extracts. After cultur ing for 24 to 48 hr at 37˚C in a CO2 incubator, 10 ml of WST-1 reagent was added, followed by additional 2 to 4 hr of incubation at 37˚C. After that, colorimetric measurements were per- formed at 450 nm and 650 nm (for reference) using a plate reader apparatus (Multiscan JX, Thermob io Analy- sis Co., Tokyo). The relative number of viable cells was expressed as a percentage of the control, based on the absorbance at 450 nm. 2.4. Inhibition of Caspase Activity and Detection of Cytochrome C The pan-caspase inhibitor Z-VAD-fmk [benzyloxycar- bonylvalyl-alanyl-aspartic acid (O-methyl)-fluoro-me- thylketone, Peptide Institute Inc., Osaka, Japan] was used to determine the caspase dependency of cell death. Cells were plated at a density of 1 to 2 × 104 per 100 ml in a 96-well plate and cultured in th e presence of gentian extract and 0 to 50 mM Z-VAD-fmk for the indicated times in a CO2 incubator. The number of viable cells was measured with either the WST-1 assay or trypan blue exclusion, as described above. Cytoplasmic and mito- chondrial cytochrome c were detected by a Cytochrome C Release Apoptosis Assay Kit (Oncogene, San Diego, USA), according to the manufacturer’s protocol. Equal amounts (10 mg) of cytoplasmic and mitochondrial pro- teins were subjected to SDS-polyacrylamide gel elec- trophoresis, followed by Western blotting using an anti-cytochrome c antibody, and the ECL Western Blot- ting Analysis System (Amersham Pharmacia Biotech). 2.5. Flow Cytometric Analysis of Mitochondrial Transmembrane Potential () and Mitochondrial Mass m Mitochondrial transmembrane poten tial () and mi- tochondrial mass were analyzed by staining cells with 200 nM MitoTracker Red CMXRos [Molecular Probes Inc. (Invitrogen)] and 200 nM MitoTracker Green FM (Molecular Probes Inc.), respectively, according to the manufacturer’s protocols. MitoTracker Red accumulates in the mitochondrial matrix under the influence of the changes in mitochondrial membrane potential. Mito- Tracker Green selectively accumulates in the mitochon- drial matrix where it binds covalently to mitochondrial proteins. Mitochondrial m m and mass-related fluo- rescence was measured using an FL1 photomultiplier and a FACS Calibur flow cytometer. The measurements were performed at least in triplicate for each dye, and the values were expressed as arbitrary scanning units of fluorescence at the peak of cell populations. 2.6. Measurements of Oxygen Consumption Oxygen consumption in intact cells was measured as an activity of mitochondrial respiration as previously de- scribed [29]. After the growth under different culture conditions, 1 to 5 × 106 cells were resuspended in 1 ml of fresh culture medium and placed in the sealed respira- tion chamber equipped with a thermostat and micro- stirrer. Oxygen consumption was measured polaro- graphically at 37˚C using the Clark-type oxygen elec- trode disk, according to the procedures recommended by the manufacturer (Oxy1, Hansatech Instrument Inc., UK). The respiration rate was expressed as nmoles of O2 consumed per 106 cells as a function of time (min). 2.7. Statistical Analysis In most cases, data are expressed as mean ± S.D. from at least three independent experiments. Statistical com- parisons were performed by one-way ANOVA. The re- sults were considered significant at a value of p < 0.05. C opyright © 2011 SciRes. ABC
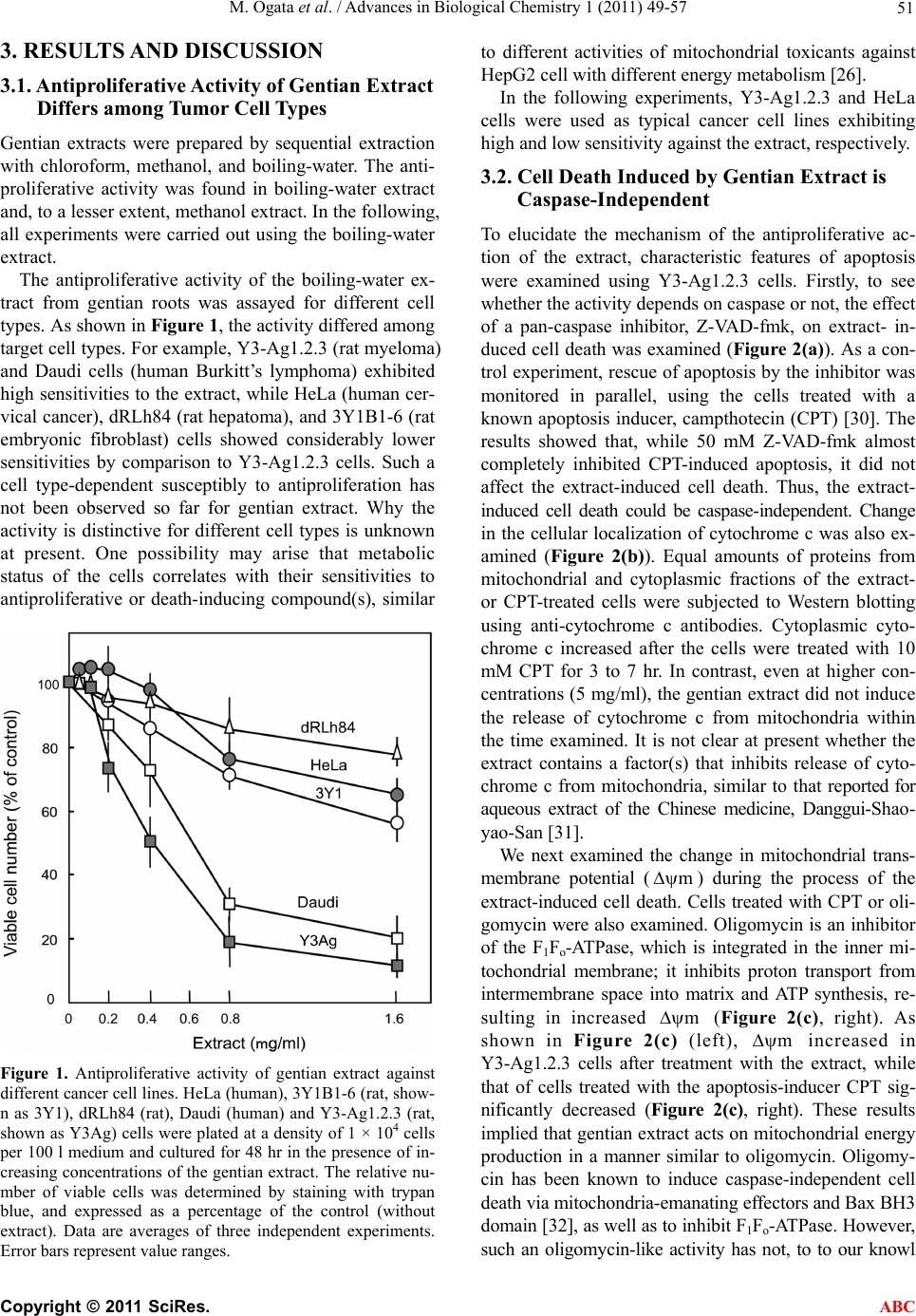 M. Ogata et al. / Advances in Biological Chemistry 1 (2011) 49-57 Copyright © 2011 SciRes. 51 3. RESULTS AND DISCUSSION to different activities of mitochondrial toxicants against HepG2 cell with different energy metabolism [26]. 3.1. Antiproliferative Activity of Gentian Extract Differs among Tumor Cell Types In the following experiments, Y3-Ag1.2.3 and HeLa cells were used as typical cancer cell lines exhibiting high and low sensitiv ity against the extract, respectively. Gentian extracts were prepared by sequential extraction with chloroform, methanol, and boiling-water. The anti- proliferative activity was found in boiling-water extract and, to a lesser extent, methanol extract. In the following, all experiments were carried out using the boiling-water extract. 3.2. Cell Death Induced by Gentian Extract is Caspase-Independent To elucidate the mechanism of the antiproliferative ac- tion of the extract, characteristic features of apoptosis were examined using Y3-Ag1.2.3 cells. Firstly, to see whether the activity depends on caspase or not, the effect of a pan-caspase inhibitor, Z-VAD-fmk, on extract- in- duced cell death was examined (Figure 2(a)). As a con- trol experiment, rescue of apoptosis by the inhibitor was monitored in parallel, using the cells treated with a known apoptosis inducer, campthotecin (CPT) [30]. The results showed that, while 50 mM Z-VAD-fmk almost completely inhibited CPT-induced apoptosis, it did not affect the extract-induced cell death. Thus, the extract- induced cell death could be caspase-independent. Change in the cellular localization of cytochrome c was also ex- amined (Figure 2(b)). Equal amounts of proteins from mitochondrial and cytoplasmic fractions of the extract- or CPT-treated cells were subjected to Western blotting using anti-cytochrome c antibodies. Cytoplasmic cyto- chrome c increased after the cells were treated with 10 mM CPT for 3 to 7 hr. In contrast, even at higher con- centrations (5 mg/ml), the g entian extract did not induce the release of cytochrome c from mitochondria within the time examined. It is not clear at present whether the extract contains a factor(s) that inhibits release of cyto- chrome c from mitochondria, similar to that reported for aqueous extract of the Chinese medicine, Danggui-Shao- yao-San [31]. The antiproliferative activity of the boiling-water ex- tract from gentian roots was assayed for different cell types. As shown in Figure 1, the activity differed among target cell types. For example, Y3-Ag1.2 .3 (rat myelo ma) and Daudi cells (human Burkitt’s lymphoma) exhibited high sensitivities to th e extract, while HeLa (human cer- vical cancer), dRLh84 (rat hepatoma), and 3Y1 B1-6 (rat embryonic fibroblast) cells showed considerably lower sensitivities by comparison to Y3-Ag1.2.3 cells. Such a cell type-dependent susceptibly to antiproliferation has not been observed so far for gentian extract. Why the activity is distinctive for different cell types is unknown at present. One possibility may arise that metabolic status of the cells correlates with their sensitivities to antiproliferative or death-inducing compound(s), similar We next examined the change in mitochondrial trans- membrane potential (m m ) during the process of the extract-induced cell death. Cells treated with CPT or oli- gomycin were also examined. Oligo mycin is an inh ibitor of the F1Fo-ATPase, which is integrated in the inner mi- tochondrial membrane; it inhibits proton transport from intermembrane space into matrix and ATP synthesis, re- sulting in increased (Figure 2(c), right). As shown in Figure 2(c) (left), increased in Y3-Ag1.2.3 cells after treatment with the extract, while that of cells treated with the apoptosis-inducer CPT sig- nificantly decreased (Figure 2(c), right). These results implied that gentian extract acts on mitochondrial energy production in a manner similar to oligomycin. Oligomy- cin has been known to induce caspase-independent cell death via mitochondria-emanating effectors and Bax BH3 domain [32], as well as to inhibit F1Fo-ATPase. However, such an oligomycin-like activity has not, to to our knowl m Figure 1. Antiproliferative activity of gentian extract against different cancer cell lines. HeLa (human), 3Y1B1-6 (rat, show- n as 3Y1), dRLh84 (rat), Daudi (human) and Y3-Ag1.2.3 (rat, shown as Y3Ag) cells were plated at a density of 1 × 104 cells per 100 l medium and cultured for 48 hr in the presence of in- creasing concentrations of the gentian extract. The relative nu- mber of viable cells was determined by staining with trypan blue, and expressed as a percentage of the control (without extract). Data are averages of three independent experiments. Error bars represent value ranges. ABC
 M. Ogata et al. / Advances in Biological Chemistry 1 (2011) 49-57 52 Figure 2. Cell death induced by gentian extract does not proceed via typical apoptotic pathways. (a) Effect of a pan-caspase inhibitor, Z-VAD-fmk, on the extract-induced cell death. Y3-Ag1.2.3 cells (Y3Ag) were cultured for 48 hr in the presence of gentian extract (extract) and Z-VAD-fmk at the concentrations indicated. As a control experiment, Y3-Ag1.2.3 cells were treated with a known apoptosis inducer, campthotecin (CPT), at 10 M for 24 hr in the presence or absence of Z-VAD-fmk. The relative number of viable cells was determined by a WST-1 assay and expressed as a percentage of the control. (b) Release of cytochrome c from mitochondria after incubation with gentian extract (left) or CPT (right). Y3-Ag1.2.3 cells were cultured in the presence or absence of gentian ex- tract or CPT for the indicated times. Equal amounts (10 g) of proteins from mitochondrial (M) and cytoplasmic (c) fractions (see Materials and methods) were subjected to Western blotting with an anti-cytochrome c antibody. (c) Changes in mitochondrial trans- membrane potential () in Y3-Ag1.2.3 cells after growth in the presence of gentian extract, camptothecin (CPT) and oligomy- cin. Cells were cultured in the presence of 0.6 mg/ml and 2 mg/ml gentian extract for 21 hr, 10 M CPT for 7 hr, and 25 M oligomycin for 3 hr. m was measured using MitoTracker Red CMXRos as described in Materials and methods. Data are averages of four to six independent experiments, and are expressed as mean ± S.D. *Statistically significant, P < 0.05, ***P < 0.001.50. m edge, been reported for extracts/compounds from plants. The results obtained from the HeLa cells were co nsisten t with a previous report [25]. We examined whether mito- chondrial energy metabolism, such as oxidative phospho- rylation, affects the antiproliferative activity of the extract. 3.3. Acti vation of Mitocho ndrial Respiration Af ter Adaptation t o Gr ow in Galactose Medium Cancer cells are generally characterized by a high gly- colytic activity and a low oxidative phosphorylation (respiration) in mitochondria [13,14,24]. From the re- sults described above, we hypothesized that low levels of mitochondrial energy metabolism affect the suscepti- bility of cells to extract-induced death. To test this, the sensitivities of the cells to the extract were examined after mitochondrial respiratory activity was forced to increase by culture conditions. This experiment was conducted based on the observations that the lowered activity of mitochondrial respiration in cancer cells can be reactivated by growth in a medium in which glucose is replaced by gal actose [25,26]. To confirm that growth in galactose medium alters mitochondrial functions in HeLa and Y3-Ag1.2.3, the cells grown in galactose were assayed for their growth rates, oxygen consumption, mitochondrial mass and tran- smembrane potential (m ). As shown in Figure 3, HeLa cells showed different growth rates when cultured in glucose and galactose media (about 2.6-fold prolong- ed doubling time in galactose). However, Y3-Ag1.2.3 cells exhibited a similar doubling time in glucose and galactose during the exponentially growing phase. The rate of oxygen consumption differed between HeLa and Y3-Ag1.2.3 cells grown in standard glucose medium (Figure 4(a)). Y3-Ag1.2.3 cells exhibited a higher rate of oxygen consumption than HeLa cells by about 1.7-fold. When grown in glucose-deprivated galactose medium, oxygen consumption rate increased by about 2.1- and 1.3-fold in HeLa and Y3-Ag1.2.3 cells, respec- tively. Galactose-grown HeLa cells reached to a similar range in the oxygen consumption rate to that of glu- cose-grown Y3-Ag1.2.3 cells. Thus, the cells grown in galactose medium enhanced their aerobic metabolism in C opyright © 2011 SciRes. ABC
 M. Ogata et al. / Advances in Biological Chemistry 1 (2011) 49-57 53 Figure 3. Proliferation of cells in different sugar media. HeLa (left) and Y3-Ag- 1.2.3 cells (right) were grown in DMEM containing glucose (Glc, circle) or galac- tose (Gal, triangle). Glucose concentrations in media for HeLa and Y3-Ag1.2.3 cells were 5.5 mM and 25 mM, respectively. Galactose concentration was 10 mM for both cell lines. The number of viable cells was counted by trypan blue staining, and the presented values are the averages of three independent experiments. Error bars denote value ranges. Figure 4. Mitochondrial activities of HeLa and Y3-Ag1.2.3 cells grown in glucose (Glc) or ga- lactose media (Gal). Concentrations of glucose and galactose were as described in the legend to Figure 3. Oxygen consumption (a), mitochondrial transmembrane potential (m , b), and mitochondrial mass (c) were measured as described in Materials and Methods. Mitochondrial and mass-related fluorescences were measured by FCM using an FL1 photomultiplier. Presented values were the averages of three to six independent experiments, and are expressed as mean ± S.D. *Statistically significant, P < 0.05, ***P < 0.001. m mitochondria. The results obtained from the HeLa cells were consistent with a previous report [25]. m in galactose-grown HeLa cells increased slig- htly, while Y3-Ag1.2.3 cells showed no significan t chan- ge, as compared to their glucose-grown counterparts (Fi- gure 4(b)). Cells grown in glucose and galactose had no statistically significant difference in their mitochondrial masses (Figure 4(c)). C opyright © 2011 SciRes. ABC
 M. Ogata et al. / Advances in Biological Chemistry 1 (2011) 49-57 54 3.4. Cells with Increased Respiration Rates Become More Sensitive to Gentian Extract To determine whether an increased rate of mitochondrial respiration affects the susceptibility of the cells to ex- tract-induced death, HeLa and Y3-Ag1.2.3 cells were cultured in glucose and in galactose media in the pres- ence or absence of the extract. As shown in Figure 5 (left), HeLa cells cultured in galactose medium exhibited a significantly higher susceptibility to extract-induced cell death, as compared with those cultured in glucose. The 50% effective concentration (EC50) of the extract for HeLa cells in glucose media was calculated to be ap- proximately 2.0 mg/ml. This value was significantly re- duced (0.6 mg/ml - 0.7 mg/ml) after the cells were ada- pted to grow in galactose. Based on the EC50 values, the sensitivity of HeLa cells to the extract-triggered death in galactose medium had increased nearly 3-fold higher than that in glucose medium. The increased sensitivity of HeLa cells is due to altered energy metabolism in galac- tose medium, since the extract by itself did not affect oxygen consumption in galactose medium (data not shown). Proliferation of Y3-Ag1.2.3 cells was also in- hibited more when the cells were grown in galactose medium. However, this increased sensitivity was seen only at higher concentrations of the extract, and the lev- els of increase were not as high as that of HeLa cells (Figure 5, right). The results presented here indicated that the gentian extract does neither induce release of cytochrome c from the mitochondria, decrease in mitochondrial transmem- brane potential, n or activation of caspases. Previous data showed that the extract triggers large-scale chromosomal fragmentation but not oligonucleosomal DNA fragmen- tation (DNA ladder) [28]. These observations do not entirely exclude a possibility that the extract ind uces cell death via apoptosis, since several caspase-independent apoptotic pathw ays have also b een kno wn [33,34 ]. How- ever, increase in m (Figure 2(c)) implies the ex- tract-induced cell death to be other than apoptosis, be- cause, apoptotic pathway involves dissipation (not in- crease) of m , which means mitochondrial transmem- brane permeabilization, i.e., one of the biochemical as- pects of distinct modalities of apoptosis [35]. Using HeLa and Y3-Ag1.2.3 cells as the representa- tive cell lines with low and high sensitivity to th e extract, respectively, correlation of the antiproliferative activity of the extract to the metabolic circumstances in the mi- tochondria was examined. The sensitivity of HeLa cells was approximately 5-fold lower than Y3-Ag1.2.3 cells. However, HeLa cells become more sensitive after adap- tation for growth in galactose medium, a conditio n under which mitochondrial respiration (oxidative phosphoryla- tion) increased by about two-fold. Thus, the resistance of HeLa cells to the extract seems to correlate with low levels of oxidative phosphorylation. The extract most Figure 5. Effect of gentian extract on the growth of cells cultured in different sugar media. HeLa (left) or Y3-Ag1.2.3 cells (right) were grown in glucose or galactose media for 48 hr in the presence of increasing concentrations of the extract. The rela- tive number of viable cells was counted by trypan blue exclusion. Values were the averages of three to four independent experiments, and were expressed as a per- centage of the control. Error bars represent value ranges. C opyright © 2011 SciRes. ABC
 M. Ogata et al. / Advances in Biological Chemistry 1 (2011) 49-57 55 likely abrogated a step(s) in the mitochondrial energy me- tabolism, and the extract- induced cell death proceeded via increased in a way similar to the action of oligo- mycin [36]. Therefore, the extrac t did not efficiently act on cells with low levels of oxidative phosphorylation. m In contrast, the cell death-inducing effects of the ex- tract on Y3-Ag1.2.3 cells increased only slightly after the cells were adapted for growth in galactose medium. The following explanation may be plausible for this. As described earlier, oxygen consumption in Y3-Ag1.2.3 cells is considerably higher than in HeLa cells in stan- dard growth conditions (glucose medium), and the in- crease of that in galactose medium is less significant as compared to that in HeLa cells. In addition, the rate of oxygen consumption in Y3-Ag1.2.3 cells in glucose is in a similar range to that in HeLa cells in galactose medium. Thus, Y3-Ag1.2.3 cells per se appear to have an innate physiological state in mitochondrial energy metabolism, which is responsible for a nearly maximal level of sus- ceptibility to the gentian extract, hence the limited in- crease in susceptibility to death in galactose medium. Critical roles for the mitochondrial respiratory chain in cell death have been demonstrated by several groups. A recent report showed that defects in the ability to carry out oxidative phosphorylation render HepG2 cells be- come more resistant to mitochondrial toxicants such as rotenone, antimycin and oligomycin [26]. In cell death triggered by ER stress and DNA damage, oxidative pho- sphorylation is required for the activation of Bax and Bak, which are essential for alteration of mitochondrial membrane permeability [37]. Consequently, cancer cells with very low levels of oxidative phosphorylation are unable to activate Bax and Bak, and thus, are able to evade cell death signals. Kwong et al. [7] also reported that cells lacking respiratory chain were protected against both mitochondrial- and ER stress-induced apo- ptosis. The action of the gentian extract on HeLa and Y3-Ag1.2.3 cells does not contradict the observations described above. The responsive gentian compound is yet to be identi- fied, although it has small molecular mass and is wa- ter-soluble. Gentian plants contain several characteristic small compounds, including gentiopicrosides, gentisic acid, oligosaccharides (gentianose and gentiobiose), and glucosides. Those are known for various activities such as inhibitor of aldose reductase, inhibitor of platelet ac- tivating factor (PAF), and scavenger of free radicals [27]. However, cell death-inducing or antiproliferative activity has not been reported for those compounds. Chroma- tographic behavior and mass spectroscopic analysis su- ggested that the cell death -inducing compound relates to gentiopicroside or its derivatives. However, commercia- lly available gentiopicroside did not show such an a- ctivity. Structure of the responsive compound (s) remains to be elucidated in the future. 4. CONCLUSIONS The gentian extract-induced cell death occurs in a cas- pase-independent pathway and in a manner depending on energy metabolism, i.e., cells with low rate of mito- chondrial respiration had lower susceptibility to the ex- tract-induced cell death as compared to those with higher level of mitochondrial respiration. This was confirmed by the fact that sensitivity to the extract increased sig- nificantly when cells were forced to switch their energy dependency from glycolysis to mitochondrial resp iration. Thus, the extract targets on mitochondrial respiration , so that different mitochondrial activities confer cells to have different susceptibilities to the extract-induced cell death. 5. ACKNOWLEDGEMENTS We thank the Hachimantai City Floricultural Research and Develop- ment Center for providing gentian plants and dried roots. We also thank Dr. R. Tsutsumi at Iwate Medical University School of Medicine for her help and discussion. REFERENCES [1] Kumar, A. and Rothman, J.H. (2007) Cell death: Hook, line and linker. Current Biology, 17, R286-R289. doi:10.1016/j.cub.2007.02.032 [2] Yuan, J. and Kroemer, G. (2010) Alternative cell death mechanisms in development and beyond. Genes & De- velopment, 24, 2592-2602. doi:10.1101/gad.1984410 [3] Orrenius, S., Nicotera, P. and Zhivotovsky, B. (2011) Cell death mechanisms and their implications in toxicology. Toxicological Sciences, 119, 3-9. doi:10.1093/toxsci/kfq268 [4] Pelicano, H. Feng, L., Zhou, Y., Carew, J.S., Hileman, E. O., Plunkett, W., Keating, M. J. and Huang, P. (2003) In- hibition of mitochondrial respiration: A novel strategy to enhance drug-induced apoptosis in human leukemia cells by a reactive oxygen species-mediated mechanism. Jour- nal of Biological Chemistry, 278, 37832-37839. doi:10.1074/jbc.M301546200 [5] Gatenby, R.A. and Gillies, R.J. (2004) Why do cancers have high aerobic glycolysis? Nature Review of Cancer, 4, 891-899. doi:10.1038/nrc1478 [6] Pelicano, H., Xu, R.H., Du, M., Feng, L., Sasaki, R., Carew, J.S., Hu, Y., Ramdas, L., Hu, L., Keating, M.J., Zhang, W., Plunkett, W. and Huang, P. (2006) Mitochon- drial respiration defects in cancer cells cause activation of Akt survival pathway through a redox-mediated me- chanism. Journal of Cell Biology, 175, 913-923. doi:10.1083/jcb.200512100 [7] Kwong, J.Q., Henning, M.S., S tar kov A.A. and M anf r edi, G. (2007) The mitochondrial respiratory chain is a mo- dulator of apoptosis. Journal of Cell Biology, 179, 1163- 1177. doi:10.1083/jcb.200704059 [8] Majno, G. and Joris, I. (1995) Apoptosis, oncosis and necrosis: An overview of cell death. American Journal of Pathology, 146, 3-15. C opyright © 2011 SciRes. ABC
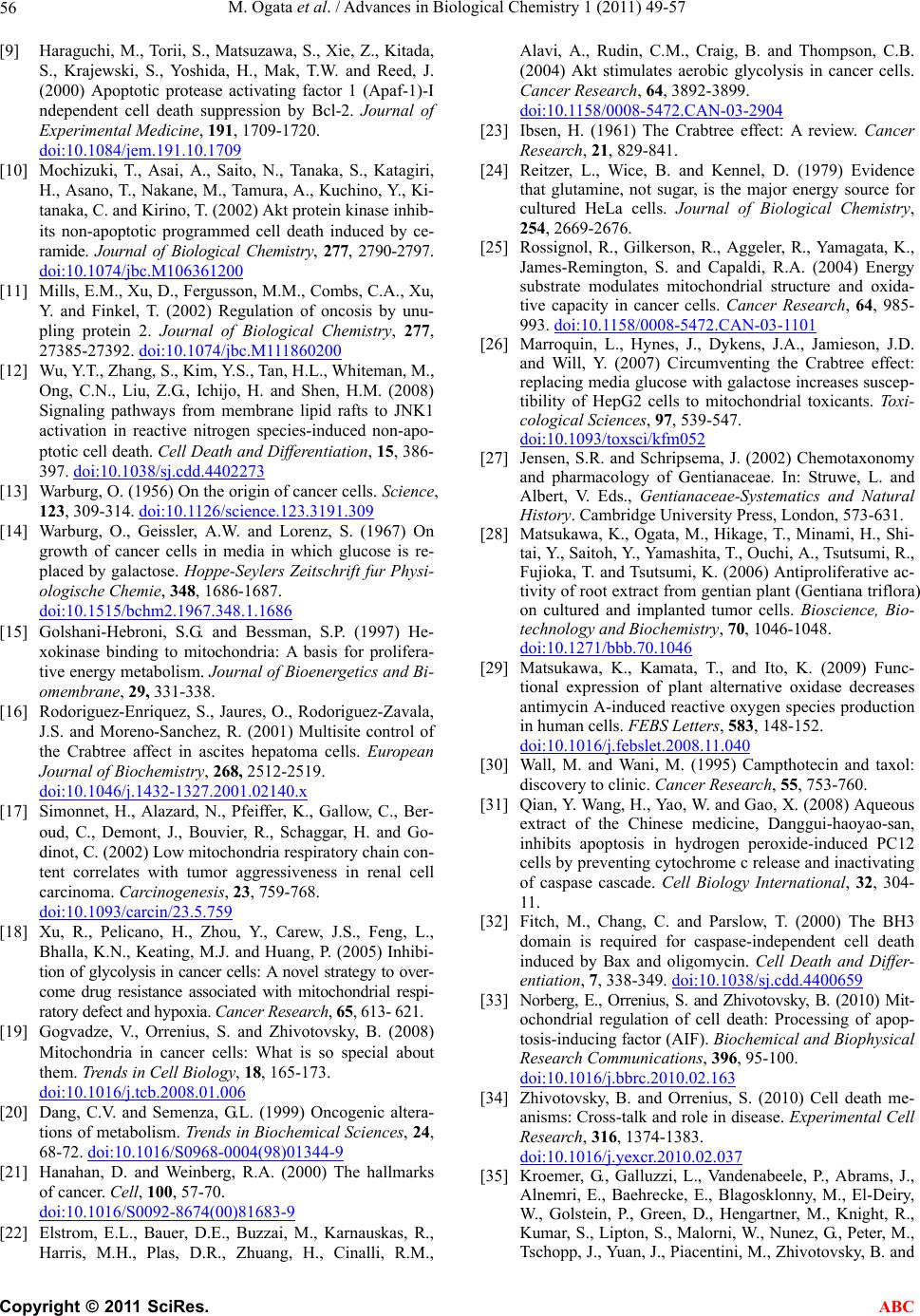 M. Ogata et al. / Advances in Biological Chemistry 1 (2011) 49-57 56 [9] Haraguchi, M., Torii, S., Matsuzawa, S., Xie, Z., Kitada, S., Krajewski, S., Yoshida, H., Mak, T.W. and Reed, J. (2000) Apoptotic protease activating factor 1 (Apaf-1)-I ndependent cell death suppression by Bcl-2. Journal of Experimental Medicine, 191, 1709-1720. doi:10.1084/jem.191.10.1709 [10] Mochizuki, T., Asai, A., Saito, N., Tanaka, S., Katagiri, H., Asano, T., Nakane, M., Tamura, A., Kuchino, Y., Ki- tanaka, C. and Kirino, T. (2002) Akt protein kinase inhib- its non-apoptotic programmed cell death induced by ce- ramide. Journal of Biological Chemistry, 277, 2790-2797. doi:10.1074/jbc.M106361200 [11] Mills, E.M., Xu, D., Fergusson, M.M., Combs, C.A., Xu, Y. and Finkel, T. (2002) Regulation of oncosis by unu- pling protein 2. Journal of Biological Chemistry, 277, 27385-27392. doi:10.1074/jbc.M111860200 [12] Wu, Y.T., Zhang, S., Kim, Y.S., Tan, H.L., Whiteman, M., Ong, C.N., Liu, Z.G., Ichijo, H. and Shen, H.M. (2008) Signaling pathways from membrane lipid rafts to JNK1 activation in reactive nitrogen species-induced non-apo- ptotic cell death. Cell Death and Differentiation, 15, 386- 397. doi:10.1038/sj.cdd.4402273 [13] Warburg, O. (1956) On the origin of cancer cells. Science, 123, 309-314. doi:10.1126/science.123.3191.309 [14] Warburg, O., Geissler, A.W. and Lorenz, S. (1967) On growth of cancer cells in media in which glucose is re- placed by galactose. Hoppe-Seylers Zeitschrift fur Physi- ologische Chemie, 348, 1686-1687. doi:10.1515/bchm2.1967.348.1.1686 [15] Golshani-Hebroni, S.G. and Bessman, S.P. (1997) He- xokinase binding to mitochondria: A basis for prolifera- tive energy metabolism. Journal of Bioenergetics and Bi- omembrane, 29, 331-338. [16] Rodoriguez-Enriquez, S., Jaures, O., Rodoriguez-Zavala, J.S. and Moreno-Sanchez, R. (2001) Multisite control of the Crabtree affect in ascites hepatoma cells. European Journal of Biochemistry, 268, 2512-2519. doi:10.1046/j.1432-1327.2001.02140.x [17] Simonnet, H., Alazard, N., Pfeiffer, K., Gallow, C., Ber- oud, C., Demont, J., Bouvier, R., Schaggar, H. and Go- dinot, C. (2002) Low mitochondria respiratory chain con- tent correlates with tumor aggressiveness in renal cell carcinoma. Carcinogenesis, 23, 759-768. doi:10.1093/carcin/23.5.759 [18] Xu, R., Pelicano, H., Zhou, Y., Carew, J.S., Feng, L., Bhalla, K.N., Keating, M.J. and Huang, P. (2005) Inhibi- tion of glycoly sis in cancer cells: A novel strategy to over- come drug resistance associated with mitochondrial respi- ratory defect and hypoxia. Cancer Re s earch, 65, 613 - 621 . [19] Gogvadze, V., Orrenius, S. and Zhivotovsky, B. (2008) Mitochondria in cancer cells: What is so special about them. Trends in Cell Biology, 18, 165-173. doi:10.1016/j.tcb.2008.01.006 [20] Dang, C.V. and Semenza, G.L. (1999) Oncogenic altera- tions of metabolism. Trends in Biochemical Sciences, 24, 68-72. doi:10.1016/S0968-0004(98)01344-9 [21] Hanahan, D. and Weinberg, R.A. (2000) The hallmarks of cancer. Cell, 100, 57-70. doi:10.1016/S0092-8674(00)81683-9 [22] Elstrom, E.L., Bauer, D.E., Buzzai, M., Karnauskas, R., Harris, M.H., Plas, D.R., Zhuang, H., Cinalli, R.M., Alavi, A., Rudin, C.M., Craig, B. and Thompson, C.B. (2004) Akt stimulates aerobic glycolysis in cancer cells. Cancer Research, 64, 3892-3899. doi:10.1158/0008-5472.CAN-03-2904 [23] Ibsen, H. (1961) The Crabtree effect: A review. Cancer Research, 21, 829-841. [24] Reitzer, L., Wice, B. and Kennel, D. (1979) Evidence that glutamine, not sugar, is the major energy source for cultured HeLa cells. Journal of Biological Chemistry, 254, 2669-2676. [25] Rossignol, R., Gilkerson, R., Aggeler, R., Yamagata, K., James-Remington, S. and Capaldi, R.A. (2004) Energy substrate modulates mitochondrial structure and oxida- tive capacity in cancer cells. Cancer Research, 64, 985- 993. doi:10.1158/0008-5472.CAN-03-1101 [26] Marroquin, L., Hynes, J., Dykens, J.A., Jamieson, J.D. and Will, Y. (2007) Circumventing the Crabtree effect: replacing media glucose with galactose increases suscep- tibility of HepG2 cells to mitochondrial toxicants. Toxi- cological Sciences, 97, 539-547. doi:10.1093/toxsci/kfm052 [27] Jensen, S.R. and Schripsema, J. (2002) Chemotaxonomy and pharmacology of Gentianaceae. In: Struwe, L. and Albert, V. Eds., Gentianaceae-Systematics and Natural History. Cambridge University Press, London, 573-631. [28] Matsukawa, K., Ogata, M., Hikage, T., Minami, H., Shi- tai, Y., Saitoh, Y., Yamashita, T., Ouchi, A., Tsutsumi, R., Fujioka, T. and Tsutsumi, K. (2006) Antiproliferative ac- tivity of root extract from gentian plant (Gentiana triflora) on cultured and implanted tumor cells. Bioscience, Bio- technology and Biochemistry, 70, 1046-1048. doi:10.1271/bbb.70.1046 [29] Matsukawa, K., Kamata, T., and Ito, K. (2009) Func- tional expression of plant alternative oxidase decreases antimycin A-induced reactive oxygen species production in human cells. FEBS Letters, 583, 148-152. doi:10.1016/j.febslet.2008.11.040 [30] Wall, M. and Wani, M. (1995) Campthotecin and taxol: discovery to clinic. Cancer Research, 55, 753-760. [31] Qian, Y. Wang, H., Yao, W. and Gao, X. (2008) Aqueous extract of the Chinese medicine, Danggui-haoyao-san, inhibits apoptosis in hydrogen peroxide-induced PC12 cells by preventing cytochrome c release and inactivating of caspase cascade. Cell Biology International, 32, 304- 11. [32] Fitch, M., Chang, C. and Parslow, T. (2000) The BH3 domain is required for caspase-independent cell death induced by Bax and oligomycin. Cell Death and Differ- entiation, 7, 338-349. doi:10.1038/sj.cdd.4400659 [33] Norberg, E., Orrenius, S. and Zhivotovsky, B. (2010) Mit- ochondrial regulation of cell death: Processing of apop- tosis-inducing factor (AIF). Biochemical and Biophysical Research Communications, 396, 95-100. doi:10.1016/j.bbrc.2010.02.163 [34] Zhivotovsky, B. and Orrenius, S. (2010) Cell death me- anisms: Cross-talk and role in disease. Experimental Cell Research, 316, 1 374-1 383. doi:10.1016/j.yexcr.2010.02.037 [35] Kroemer, G., Galluzzi, L., Vandenabeele, P., Abrams, J., Alnemri, E., Baehrecke, E., Blagosklonny, M., El-Deiry, W., Golstein, P., Green, D., Hengartner, M., Knight, R., Kumar, S., Lipton, S., Ma lorni, W., Nunez, G., Pet er, M., Tschopp, J., Yuan, J., Piacentini, M., Zhivotovsky, B. and C opyright © 2011 SciRes. ABC
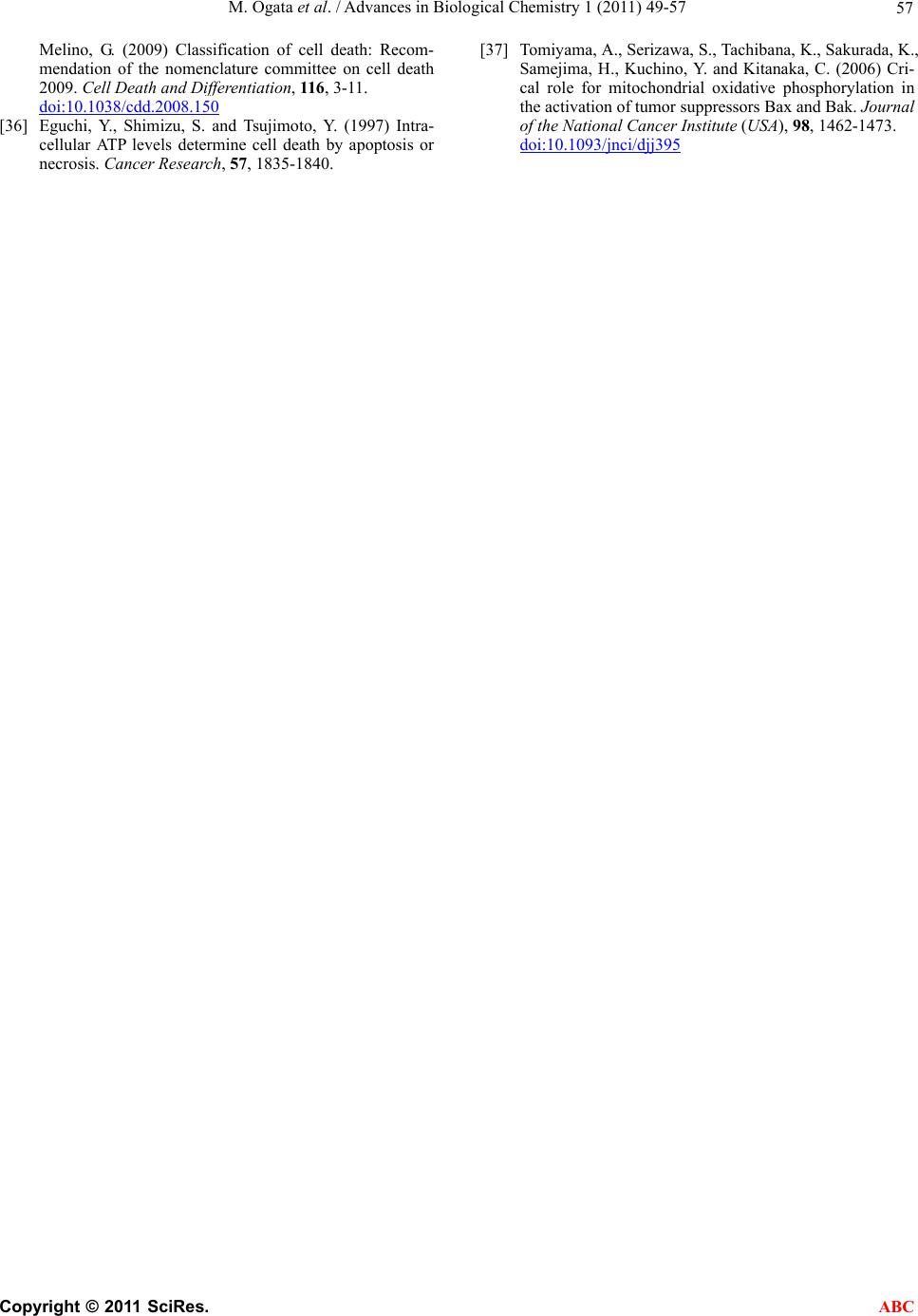 M. Ogata et al. / Advances in Biological Chemistry 1 (2011) 49-57 Copyright © 2011 SciRes. 57 ABC Melino, G. (2009) Classification of cell death: Recom- mendation of the nomenclature committee on cell death 2009. Cell Death and Differentiation, 116, 3-11. doi:10.1038/cdd.2008.150 [36] Eguchi, Y., Shimizu, S. and Tsujimoto, Y. (1997) Intra- cellular ATP levels determine cell death by apoptosis or necrosis. Cancer Research, 57, 1835-1840. [37] Tomiyama, A., Serizawa, S., Tachibana, K., Sakurada, K., Samejima, H., Kuchino, Y. and Kitanaka, C. (2006) Cri- cal role for mitochondrial oxidative phosphorylation in the activation of tumor suppressors Bax and Bak. Journal of the National Cancer Institute (USA), 98, 1462-1473. doi:10.1093/jnci/djj395
|