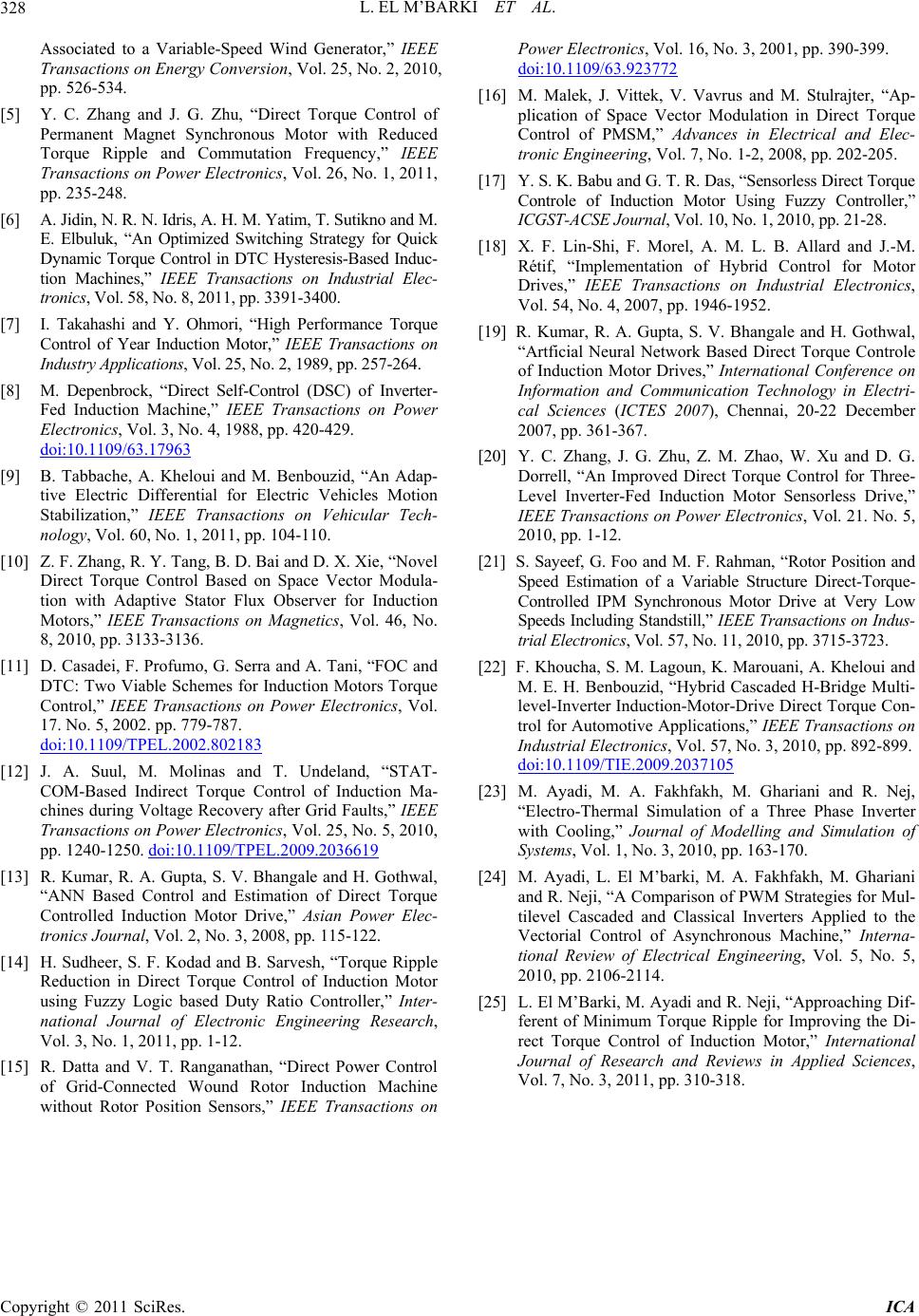
L. EL M’BARKI ET AL.
Copyright © 2011 SciRes. ICA
328
Associated to a Variable-Speed Wind Generator,” IEEE
Transactions on Energy Conversion, Vol. 25, No. 2, 2010,
pp. 526-534.
[5] Y. C. Zhang and J. G. Zhu, “Direct Torque Control of
Permanent Magnet Synchronous Motor with Reduced
Torque Ripple and Commutation Frequency,” IEEE
Transactions on Power Electronics, Vol. 26, No. 1, 2011,
pp. 235-248.
[6] A. Jidin, N. R. N. Idris, A. H. M. Yatim, T. Sutikno and M.
E. Elbuluk, “An Optimized Switching Strategy for Quick
Dynamic Torque Control in DTC Hysteresis-Based Induc-
tion Machines,” IEEE Transactions on Industrial Elec-
tronics, Vol. 58, No. 8, 2011, pp. 3391-3400.
[7] I. Takahashi and Y. Ohmori, “High Performance Torque
Control of Year Induction Motor,” IEEE Transactions on
Industry Applications, Vol. 25, No. 2, 1989, pp. 257-264.
[8] M. Depenbrock, “Direct Self-Control (DSC) of Inverter-
Fed Induction Machine,” IEEE Transactions on Power
Electronics, Vol. 3, No. 4, 1988, pp. 420-429.
doi:10.1109/63.17963
[9] B. Tabbache, A. Kheloui and M. Benbouzid, “An Adap-
tive Electric Differential for Electric Vehicles Motion
Stabilization,” IEEE Transactions on Vehicular Tech-
nology, Vol. 60, No. 1, 2011, pp. 104-110.
[10] Z. F. Zhang, R. Y. Tang, B. D. Bai and D. X. Xie, “Novel
Direct Torque Control Based on Space Vector Modula-
tion with Adaptive Stator Flux Observer for Induction
Motors,” IEEE Transactions on Magnetics, Vol. 46, No.
8, 2010, pp. 3133-3136.
[11] D. Casadei, F. Profumo, G. Serra and A. Tani, “FOC and
DTC: Two Viable Schemes for Induction Motors Torque
Control,” IEEE Transactions on Power Electronics, Vol.
17. No. 5, 2002. pp. 779-787.
doi:10.1109/TPEL.2002.802183
[12] J. A. Suul, M. Molinas and T. Undeland, “STAT-
COM-Based Indirect Torque Control of Induction Ma-
chines during Voltage Recovery after Grid Faults,” IEEE
Transactions on Power Electronics, Vol. 25, No. 5, 2010,
pp. 1240-1250. doi:10.1109/TPEL.2009.2036619
[13] R. Kumar, R. A. Gupta, S. V. Bhangale and H. Gothwal,
“ANN Based Control and Estimation of Direct Torque
Controlled Induction Motor Drive,” Asian Power Elec-
tronics Journal, Vol. 2, No. 3, 2008, pp. 115-122.
[14] H. Sudheer, S. F. Kodad and B. Sarvesh, “Torque Ripple
Reduction in Direct Torque Control of Induction Motor
using Fuzzy Logic based Duty Ratio Controller,” Inter-
national Journal of Electronic Engineering Research,
Vol. 3, No. 1, 2011, pp. 1-12.
[15] R. Datta and V. T. Ranganathan, “Direct Power Control
of Grid-Connected Wound Rotor Induction Machine
without Rotor Position Sensors,” IEEE Transactions on
Power Electronics, Vol. 16, No. 3, 2001, pp. 390-399.
doi:10.1109/63.923772
[16] M. Malek, J. Vittek, V. Vavrus and M. Stulrajter, “Ap-
plication of Space Vector Modulation in Direct Torque
Control of PMSM,” Advances in Electrical and Elec-
tronic Engineering, Vol. 7, No. 1-2, 2008, pp. 202-205.
[17] Y. S. K. Babu and G. T. R. Das, “Sensorless Direct Torque
Controle of Induction Motor Using Fuzzy Controller,”
ICGST-ACSE Journal, Vol. 10, No. 1, 2010, pp. 21-28.
[18] X. F. Lin-Shi, F. Morel, A. M. L. B. Allard and J.-M.
Rétif, “Implementation of Hybrid Control for Motor
Drives,” IEEE Transactions on Industrial Electronics,
Vol. 54, No. 4, 2007, pp. 1946-1952.
[19] R. Kumar, R. A. Gupta, S. V. Bhangale and H. Gothwal,
“Artficial Neural Network Based Direct Torque Controle
of Induction Motor Drives,” International Conference on
Information and Communication Technology in Electri-
cal Sciences (ICTES 2007), Chennai, 20-22 December
2007, pp. 361-367.
[20] Y. C. Zhang, J. G. Zhu, Z. M. Zhao, W. Xu and D. G.
Dorrell, “An Improved Direct Torque Control for Three-
Level Inverter-Fed Induction Motor Sensorless Drive,”
IEEE Transactions on Power Electronics, Vol. 21. No. 5,
2010, pp. 1-12.
[21] S. Sayeef, G. Foo and M. F. Rahman, “Rotor Position and
Speed Estimation of a Variable Structure Direct-Torque-
Controlled IPM Synchronous Motor Drive at Very Low
Speeds Including Standstill,” IEEE Transactions on Indus-
trial Electronics, Vol. 57, No. 11, 2010, pp. 3715-3723.
[22] F. Khoucha, S. M. Lagoun, K. Marouani, A. Kheloui and
M. E. H. Benbouzid, “Hybrid Cascaded H-Bridge Multi-
level-Inverter Induction-Motor-Drive Direct Torque Con-
trol for Automotive Applications,” IEEE Transactions on
Industrial Electronics, Vol. 57, No. 3, 2010, pp. 892-899.
doi:10.1109/TIE.2009.2037105
[23] M. Ayadi, M. A. Fakhfakh, M. Ghariani and R. Nej,
“Electro-Thermal Simulation of a Three Phase Inverter
with Cooling,” Journal of Modelling and Simulation of
Systems, Vol. 1, No. 3, 2010, pp. 163-170.
[24] M. Ayadi, L. El M’barki, M. A. Fakhfakh, M. Ghariani
and R. Neji, “A Comparison of PWM Strategies for Mul-
tilevel Cascaded and Classical Inverters Applied to the
Vectorial Control of Asynchronous Machine,” Interna-
tional Review of Electrical Engineering, Vol. 5, No. 5,
2010, pp. 2106-2114.
[25] L. El M’Barki, M. Ayadi and R. Neji, “Approaching Dif-
ferent of Minimum Torque Ripple for Improving the Di-
rect Torque Control of Induction Motor,” International
Journal of Research and Reviews in Applied Sciences,
Vol. 7, No. 3, 2011, pp. 310-318.