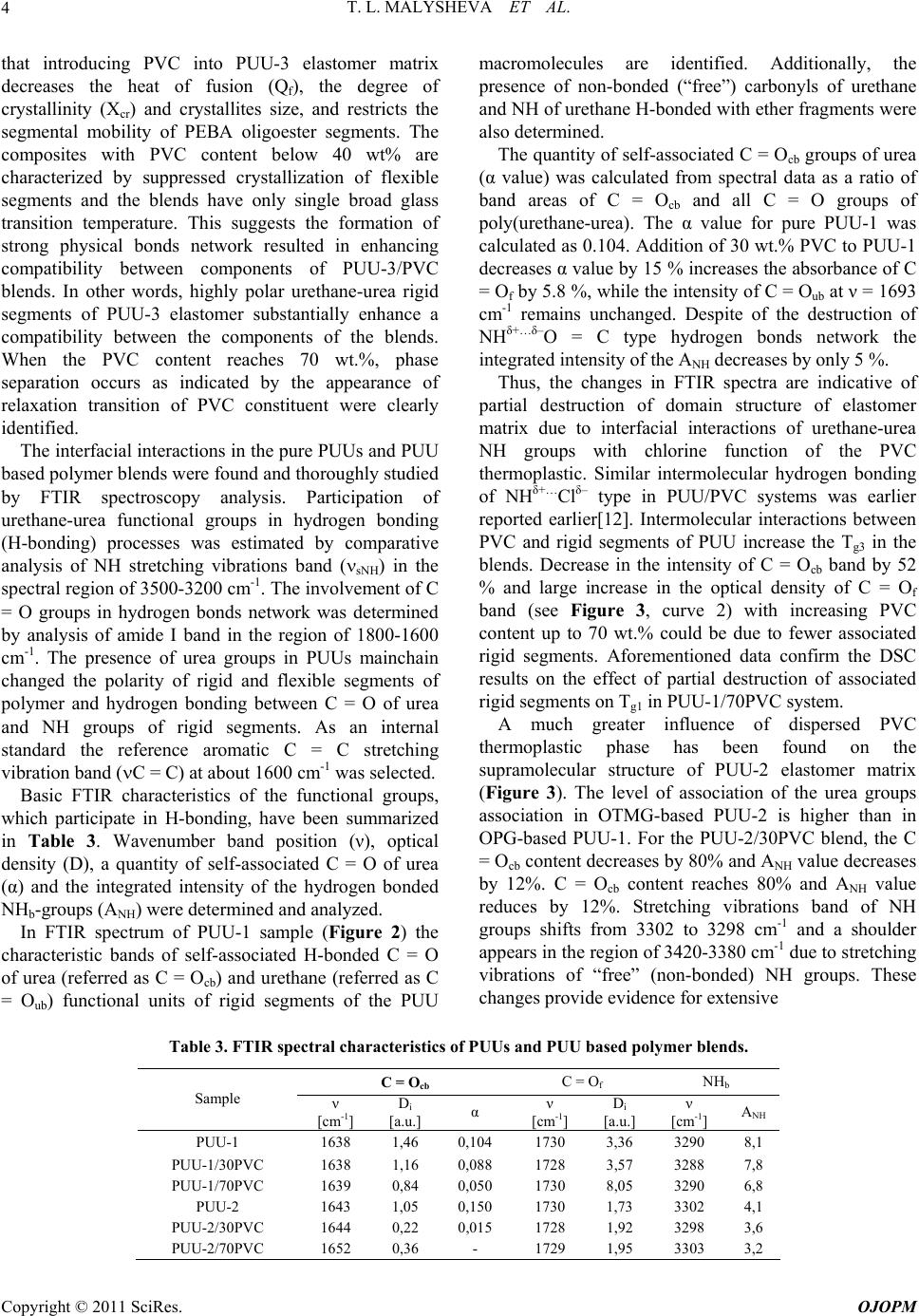
T. L. MALYSHEVA ET AL.
4
that introducing PVC into PUU-3 elastomer matrix
decreases the heat of fusion (Qf), the degree of
crystallinity (Xcr) and crystallites size, and restricts the
segmental mobility of PEBA oligoester segments. The
composites with PVC content below 40 wt% are
characterized by suppressed crystallization of flexible
segments and the blends have only single broad glass
transition temperature. This suggests the formation of
strong physical bonds network resulted in enhancing
compatibility between components of PUU-3/PVC
blends. In other words, highly polar urethane-urea rigid
segments of PUU-3 elastomer substantially enhance a
compatibility between the components of the blends.
When the PVC content reaches 70 wt.%, phase
separation occurs as indicated by the appearance of
relaxation transition of PVC constituent were clearly
identified.
The interfacial interactions in the pure PUUs and PUU
based polymer blends were found and thoroughly studied
by FTIR spectroscopy analysis. Participation of
urethane-urea functional groups in hydrogen bonding
(H-bonding) processes was estimated by comparative
analysis of NH stretching vibrations band (sNH) in the
spectral region of 3500-3200 cm-1. The involvement of C
= O groups in hydrogen bonds network was determined
by analysis of amide I band in the region of 1800-1600
cm-1. The presence of urea groups in PUUs mainchain
changed the polarity of rigid and flexible segments of
polymer and hydrogen bonding between C = O of urea
and NH groups of rigid segments. As an internal
standard the reference aromatic C = C stretching
vibration band (C = C) at about 1600 cm-1 was selected.
Basic FTIR characteristics of the functional groups,
which participate in H-bonding, have been summarized
in Table 3. Wavenumber band position (ν), optical
density (D), a quantity of self-associated C = O of urea
(α) and the integrated intensity of the hydrogen bonded
NHb-groups (ANH) were determined and analyzed.
In FTIR spectrum of PUU-1 sample (Figure 2) the
characteristic bands of self-associated H-bonded C = O
of urea (referred as C = Ocb) and urethane (referred as C
= Oub) functional units of rigid segments of the PUU
macromolecules are identified. Additionally, the
presence of non-bonded (“free”) carbonyls of urethane
and NH of urethane H-bonded with ether fragments were
also determined.
The quantity of self-associated C = Ocb groups of urea
(α value) was calculated from spectral data as a ratio of
band areas of C = Ocb and all C = O groups of
poly(urethane-urea). The α value for pure PUU-1 was
calculated as 0.104. Addition of 30 wt.% PVC to PUU-1
decreases α value by 15 % increases the absorbance of C
= Of by 5.8 %, while the intensity of C = Oub at ν = 1693
cm-1 remains unchanged. Despite of the destruction of
NHδ+…δ–O = C type hydrogen bonds network the
integrated intensity of the ANH decreases by only 5 %.
Thus, the changes in FTIR spectra are indicative of
partial destruction of domain structure of elastomer
matrix due to interfacial interactions of urethane-urea
NH groups with chlorine function of the PVC
thermoplastic. Similar intermolecular hydrogen bonding
of NHδ+…Clδ– type in PUU/PVC systems was earlier
reported earlier[12]. Intermolecular interactions between
PVC and rigid segments of PUU increase the Tg3 in the
blends. Decrease in the intensity of C = Ocb band by 52
% and large increase in the optical density of C = Of
band (see Figure 3, curve 2) with increasing PVC
content up to 70 wt.% could be due to fewer associated
rigid segments. Aforementioned data confirm the DSC
results on the effect of partial destruction of associated
rigid segments on Tg1 in PUU-1/70PVC system.
A much greater influence of dispersed PVC
thermoplastic phase has been found on the
supramolecular structure of PUU-2 elastomer matrix
(Figure 3). The level of association of the urea groups
association in OTMG-based PUU-2 is higher than in
OPG-based PUU-1. For the PUU-2/30PVC blend, the C
= Ocb content decreases by 80% and ANH value decreases
by 12%. C = Ocb content reaches 80% and ANH value
reduces by 12%. Stretching vibrations band of NH
groups shifts from 3302 to 3298 cm-1 and a shoulder
appears in the region of 3420-3380 cm-1 due to stretching
vibrations of “free” (non-bonded) NH groups. These
changes provide evidence for extensive
Table 3. FTIR spectral characteristics of PUUs and PUU based polymer blends.
С = Ocb C = Of NHb
Sample ν
[сm-1]
Di
[a.u.] α ν
[сm-1]
Di
[a.u.]
ν
[сm-1] АNH
PUU-1 1638 1,46 0,104 1730 3,36 3290 8,1
PUU-1/30PVC 1638 1,16 0,088 1728 3,57 3288 7,8
PUU-1/70PVC 1639 0,84 0,050 1730 8,05 3290 6,8
PUU-2 1643 1,05 0,150 1730 1,73 3302 4,1
PUU-2/30PVC 1644 0,22 0,015 1728 1,92 3298 3,6
PUU-2/70PVC 1652 0,36 - 1729 1,95 3303 3,2
Copyright © 2011 SciRes. OJOPM