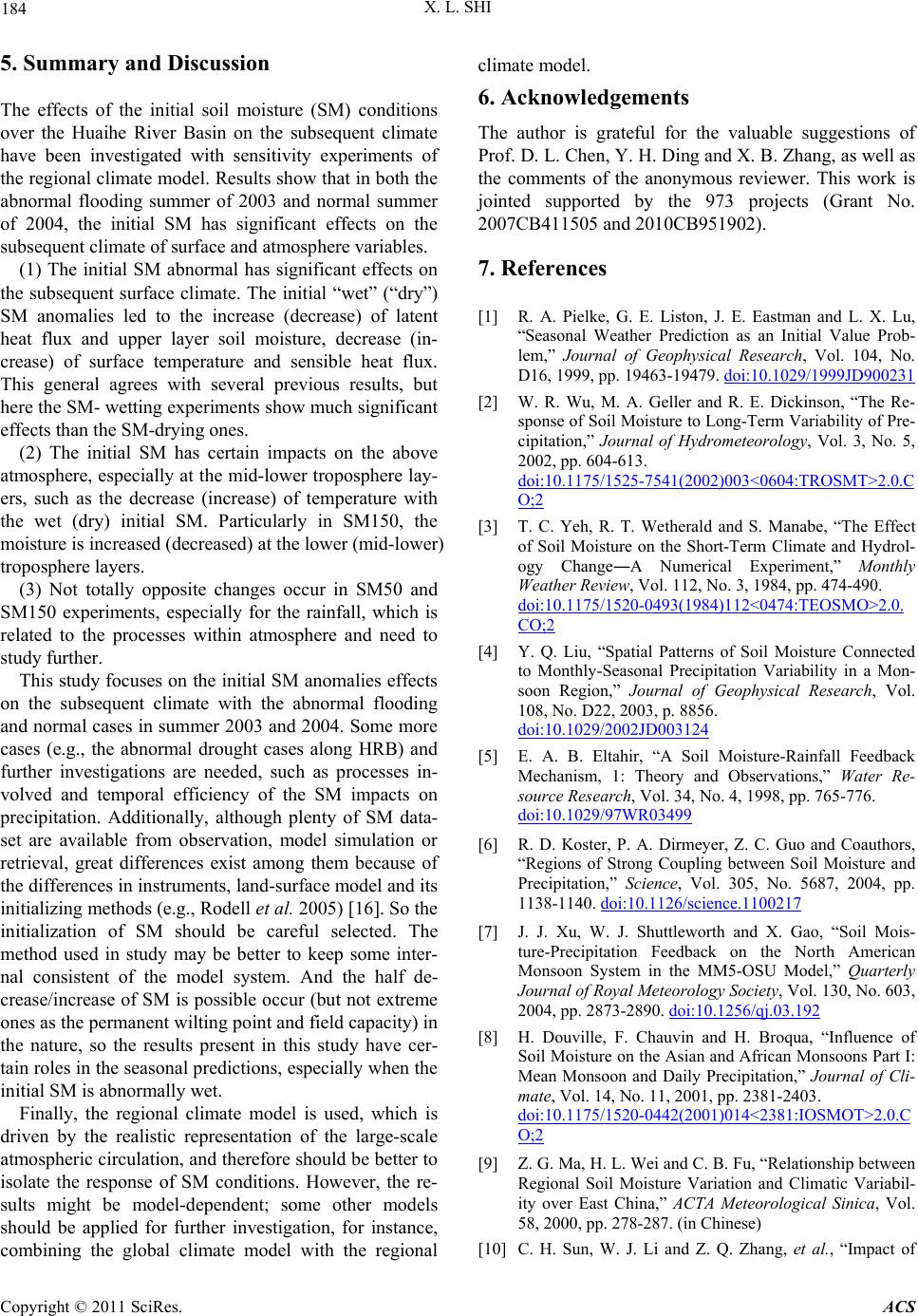
X. L. SHI
Copyright © 2011 SciRes. ACS
184
5. Summary and Discussion
The effects of the initial soil moisture (SM) conditions
over the Huaihe River Basin on the subsequent climate
have been investigated with sensitivity experiments of
the regional climate model. Results show that in both the
abnormal flooding summer of 2003 and normal summer
of 2004, the initial SM has significant effects on the
subsequent climate of surface and atmosphere variables.
(1) The initial SM abnormal has significant effects on
the subsequent surface climate. The initial “wet” (“dry”)
SM anomalies led to the increase (decrease) of latent
heat flux and upper layer soil moisture, decrease (in-
crease) of surface temperature and sensible heat flux.
This general agrees with several previous results, but
here the SM- wetting experiments show much significant
effects than the SM-drying ones.
(2) The initial SM has certain impacts on the above
atmosphere, especially at the mid-lower troposphere lay-
ers, such as the decrease (increase) of temperature with
the wet (dry) initial SM. Particularly in SM150, the
moisture is increased (decreased) at the lower (mid-lower)
troposphere layers.
(3) Not totally opposite changes occur in SM50 and
SM150 experiments, especially for the rainfall, which is
related to the processes within atmosphere and need to
study further.
This study focuses on the initial SM anomalies effects
on the subsequent climate with the abnormal flooding
and normal cases in summer 2003 and 2004. Some more
cases (e.g., the abnormal drought cases along HRB) and
further investigations are needed, such as processes in-
volved and temporal efficiency of the SM impacts on
precipitation. Additionally, although plenty of SM data-
set are available from observation, model simulation or
retrieval, great differences exist among them because of
the differences in instruments, land-surface model and its
initializing methods (e.g., Rodell et al. 2005) [16]. So the
initialization of SM should be careful selected. The
method used in study may be better to keep some inter-
nal consistent of the model system. And the half de-
crease/increase of SM is possible occur (but not extreme
ones as the permanent wilting point and field capacity) in
the nature, so the results present in this study have cer-
tain roles in the seasonal predictions, especially when the
initial SM is abnormally wet.
Finally, the regional climate model is used, which is
driven by the realistic representation of the large-scale
atmospheric circulation, and therefore should be better to
isolate the response of SM conditions. However, the re-
sults might be model-dependent; some other models
should be applied for further investigation, for instance,
combining the global climate model with the regional
climate model.
6. Acknowledgements
The author is grateful for the valuable suggestions of
Prof. D. L. Chen, Y. H. Ding and X. B. Zhang, as well as
the comments of the anonymous reviewer. This work is
jointed supported by the 973 projects (Grant No.
2007CB411505 and 2010CB951902).
7. References
[1] R. A. Pielke, G. E. Liston, J. E. Eastman and L. X. Lu,
“Seasonal Weather Prediction as an Initial Value Prob-
lem,” Journal of Geophysical Research, Vol. 104, No.
D16, 1999, pp. 19463-19479. doi:10.1029/1999JD900231
[2] W. R. Wu, M. A. Geller and R. E. Dickinson, “The Re-
sponse of Soil Moisture to Long-Term Variability of Pre-
cipitation,” Journal of Hydrometeorology, Vol. 3, No. 5,
2002, pp. 604-613.
doi:10.1175/1525-7541(2002)003<0604:TROSMT>2.0.C
O;2
[3] T. C. Yeh, R. T. Wetherald and S. Manabe, “The Effect
of Soil Moisture on the Short-Term Climate and Hydrol-
ogy Change―A Numerical Experiment,” Monthly
Weather Review, Vol. 112, No. 3, 1984, pp. 474-490.
doi:10.1175/1520-0493(1984)112<0474:TEOSMO>2.0.
CO;2
[4] Y. Q. Liu, “Spatial Patterns of Soil Moisture Connected
to Monthly-Seasonal Precipitation Variability in a Mon-
soon Region,” Journal of Geophysical Research, Vol.
108, No. D22, 2003, p. 8856.
doi:10.1029/2002JD003124
[5] E. A. B. Eltahir, “A Soil Moisture-Rainfall Feedback
Mechanism, 1: Theory and Observations,” Water Re-
source Research, Vol. 34, No. 4, 1998, pp. 765-776.
doi:10.1029/97WR03499
[6] R. D. Koster, P. A. Dirmeyer, Z. C. Guo and Coauthors,
“Regions of Strong Coupling between Soil Moisture and
Precipitation,” Science, Vol. 305, No. 5687, 2004, pp.
1138-1140. doi:10.1126/science.1100217
[7] J. J. Xu, W. J. Shuttleworth and X. Gao, “Soil Mois-
ture-Precipitation Feedback on the North American
Monsoon System in the MM5-OSU Model,” Quarterly
Journal of Royal Meteorology Society, Vol. 130, No. 603,
2004, pp. 2873-2890. doi:10.1256/qj.03.192
[8] H. Douville, F. Chauvin and H. Broqua, “Influence of
Soil Moisture on the Asian and African Monsoons Part I:
Mean Monsoon and Daily Precipitation,” Journal of Cli-
mate, Vol. 14, No. 11, 2001, pp. 2381-2403.
doi:10.1175/1520-0442(2001)014<2381:IOSMOT>2.0.C
O;2
[9] Z. G. Ma, H. L. Wei and C. B. Fu, “Relationship between
Regional Soil Moisture Variation and Climatic Variabil-
ity over East China,” ACTA Meteorological Sinica, Vol.
58, 2000, pp. 278-287. (in Chinese)
[10] C. H. Sun, W. J. Li and Z. Q. Zhang, et al., “Impact of