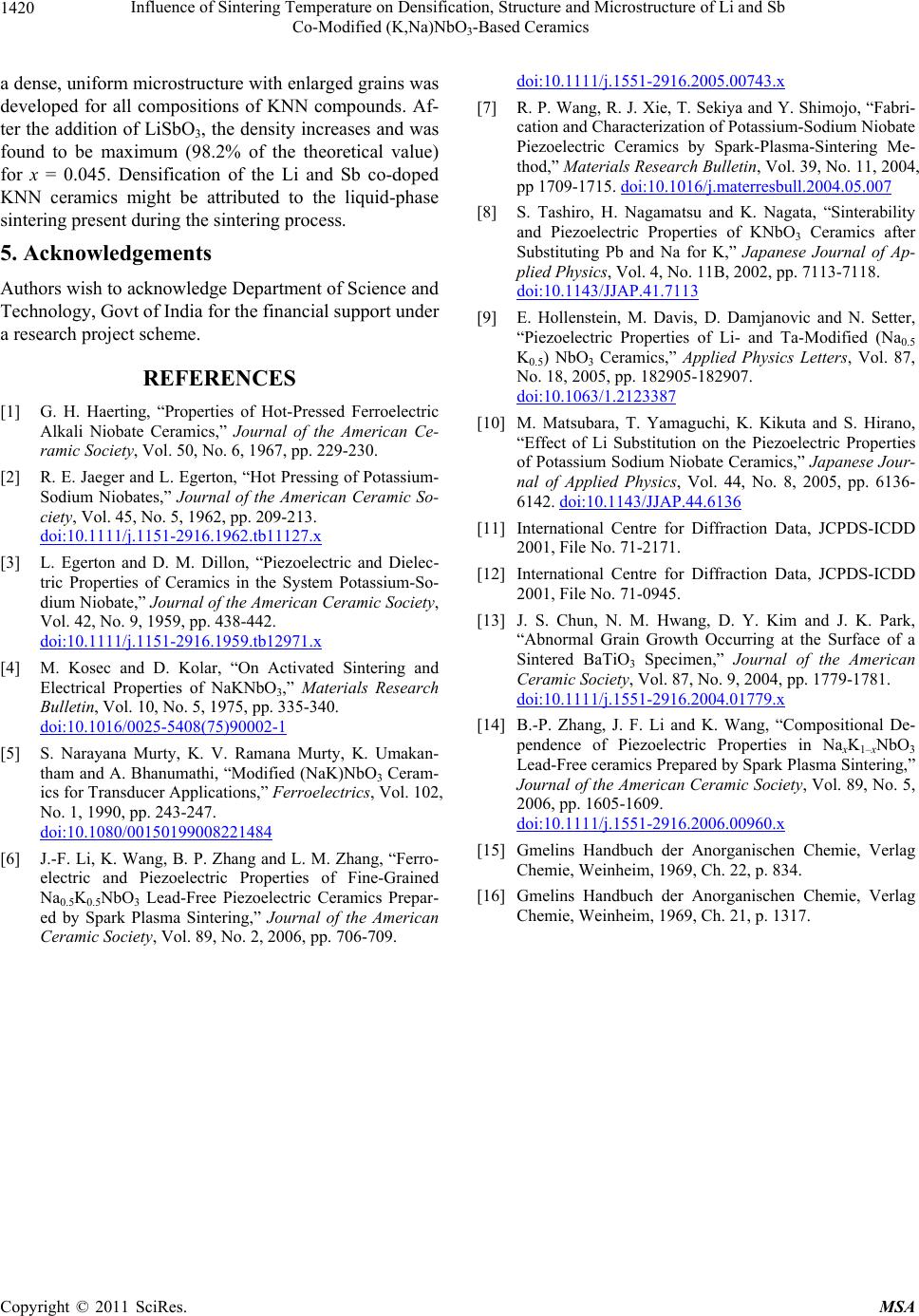
Influence of Sintering Temperature on Densification, Structure and Microstructure of Li and Sb
1420
Co-Modified (K,Na)NbO3-Based Ceramics
a dense, uniform microstructure with enlarged grains was
developed for all compositions of KNN compounds. Af-
ter the addition of LiSbO3, the density increases and was
found to be maximum (98.2% of the theoretical value)
for x = 0.045. Densification of the Li and Sb co-doped
KNN ceramics might be attributed to the liquid-phase
sintering present during the sintering process.
5. Acknowledgements
Authors wish to acknowledge Department of Science and
Technology, Govt of India for the financial support under
a research project scheme.
REFERENCES
[1] G. H. Haerting, “Properties of Hot-Pressed Ferroelectric
Alkali Niobate Ceramics,” Journal of the American Ce-
ramic Society, Vol. 50, No. 6, 1967, pp. 229-230.
[2] R. E. Jaeger and L. Egerton, “Hot Pressing of Potassium-
Sodium Niobates,” Journal of the American Ceramic So-
ciety, Vol. 45, No. 5, 1962, pp. 209-213.
doi:10.1111/j.1151-2916.1962.tb11127.x
[3] L. Egerton and D. M. Dillon, “Piezoelectric and Dielec-
tric Properties of Ceramics in the System Potassium-So-
dium Niobate,” Journal of the American Ceramic Society,
Vol. 42, No. 9, 1959, pp. 438-442.
doi:10.1111/j.1151-2916.1959.tb12971.x
[4] M. Kosec and D. Kolar, “On Activated Sintering and
Electrical Properties of NaKNbO3,” Materials Research
Bulletin, Vol. 10, No. 5, 1975, pp. 335-340.
doi:10.1016/0025-5408(75)90002-1
[5] S. Narayana Murty, K. V. Ramana Murty, K. Umakan-
tham and A. Bhanumathi, “Modified (NaK)NbO3 Ceram-
ics for Transducer Applications,” Ferroelectrics, Vol. 102,
No. 1, 1990, pp. 243-247.
doi:10.1080/00150199008221484
[6] J.-F. Li, K. Wang, B. P. Zhang and L. M. Zhang, “Ferro-
electric and Piezoelectric Properties of Fine-Grained
Na0.5K0.5NbO3 Lead-Free Piezoelectric Ceramics Prepar-
ed by Spark Plasma Sintering,” Journal of the American
Ceramic Society, Vol. 89, No. 2, 2006, pp. 706-709.
doi:10.1111/j.1551-2916.2005.00743.x
[7] R. P. Wang, R. J. Xie, T. Sekiya and Y. Shimojo, “Fabri-
cation and Characterization of Potassium-Sodium Niobate
Piezoelectric Ceramics by Spark-Plasma-Sintering Me-
thod,” Materials Research Bulletin, Vol. 39, No. 11, 2004,
pp 1709-1715. doi:10.1016/j.materresbull.2004.05.007
[8] S. Tashiro, H. Nagamatsu and K. Nagata, “Sinterability
and Piezoelectric Properties of KNbO3 Ceramics after
Substituting Pb and Na for K,” Japanese Journal of Ap-
plied Physics, Vol. 4, No. 11B, 2002, pp. 7113-7118.
doi:10.1143/JJAP.41.7113
[9] E. Hollenstein, M. Davis, D. Damjanovic and N. Setter,
“Piezoelectric Properties of Li- and Ta-Modified (Na0.5
K0.5) NbO3 Ceramics,” Applied Physics Letters, Vol. 87,
No. 18, 2005, pp. 182905-182907.
doi:10.1063/1.2123387
[10] M. Matsubara, T. Yamaguchi, K. Kikuta and S. Hirano,
“Effect of Li Substitution on the Piezoelectric Properties
of Potassium Sodium Niobate Ceramics,” Japanese Jour-
nal of Applied Physics, Vol. 44, No. 8, 2005, pp. 6136-
6142. doi:10.1143/JJAP.44.6136
[11] International Centre for Diffraction Data, JCPDS-ICDD
2001, File No. 71-2171.
[12] International Centre for Diffraction Data, JCPDS-ICDD
2001, File No. 71-0945.
[13] J. S. Chun, N. M. Hwang, D. Y. Kim and J. K. Park,
“Abnormal Grain Growth Occurring at the Surface of a
Sintered BaTiO3 Specimen,” Journal of the American
Ceramic Society, Vol. 87, No. 9, 2004, pp. 1779-1781.
doi:10.1111/j.1551-2916.2004.01779.x
[14] B.-P. Zhang, J. F. Li and K. Wang, “Compositional De-
pendence of Piezoelectric Properties in NaxK1–xNbO3
Lead-Free ceramics Prepared by Spark Plasma Sintering,”
Journal of the American Ceramic Society, Vol. 89, No. 5,
2006, pp. 1605-1609.
doi:10.1111/j.1551-2916.2006.00960.x
[15] Gmelins Handbuch der Anorganischen Chemie, Verlag
Chemie, Weinheim, 1969, Ch. 22, p. 834.
[16] Gmelins Handbuch der Anorganischen Chemie, Verlag
Chemie, Weinheim, 1969, Ch. 21, p. 1317.
Copyright © 2011 SciRes. MSA