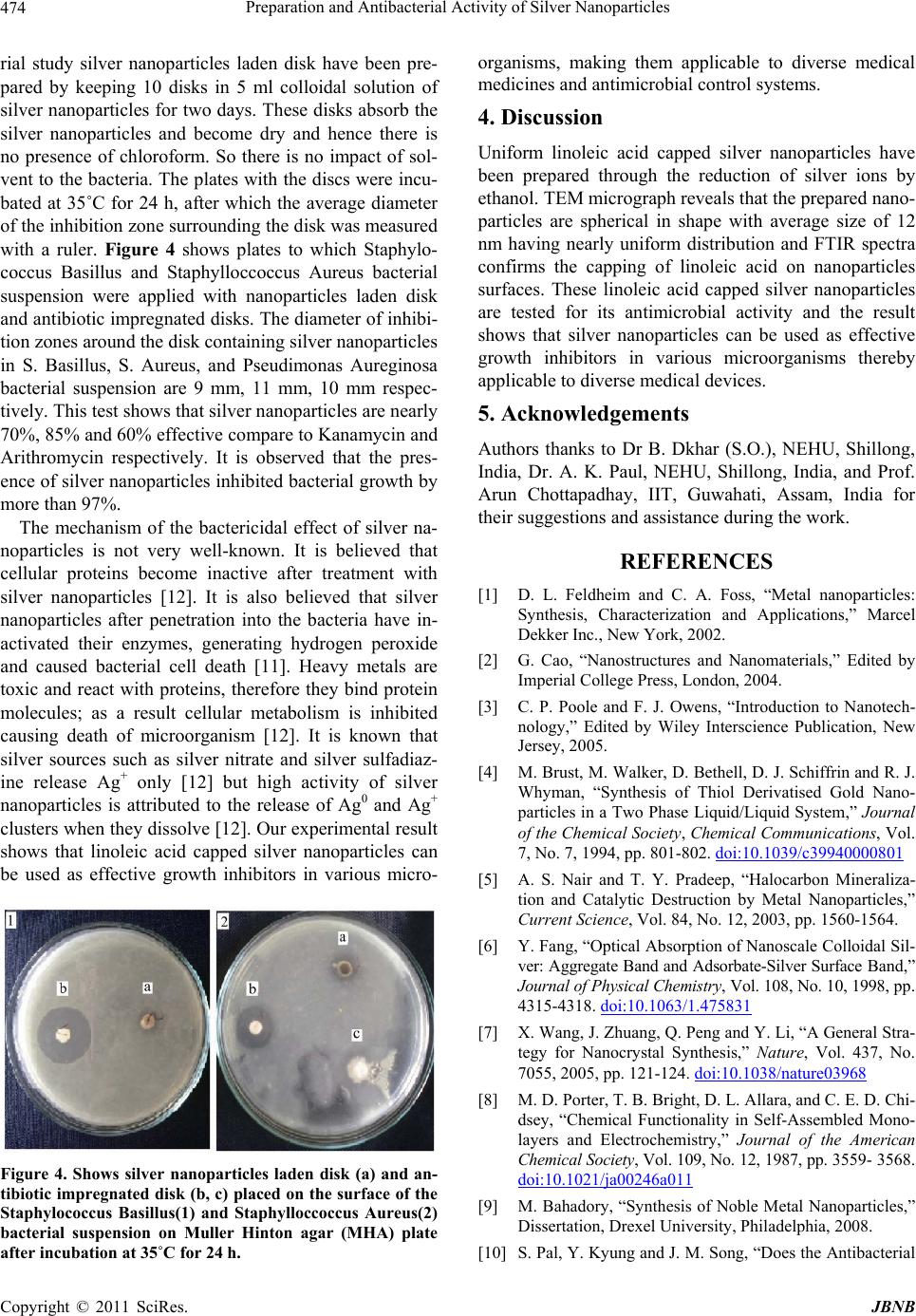
Preparation and Antibacterial Activity of Silver Nanoparticles
474
rial study silver nanoparticles laden disk have been pre-
pared by keeping 10 disks in 5 ml colloidal solution of
silver nanoparticles for two days. These disks absorb the
silver nanoparticles and become dry and hence there is
no presence of chloroform. So there is no impact of sol-
vent to the bacteria. The plates with the discs were incu-
bated at 35˚C for 24 h, after which the average diameter
of the inhibitio n zon e surround ing th e disk was measured
with a ruler. Figure 4 shows plates to which Staphylo-
coccus Basillus and Staphylloccoccus Aureus bacterial
suspension were applied with nanoparticles laden disk
and antibiotic impregnated disks. The diameter of inhibi-
tion zones around the disk containing silver nanoparticles
in S. Basillus, S. Aureus, and Pseudimonas Aureginosa
bacterial suspension are 9 mm, 11 mm, 10 mm respec-
tively. This test shows that silver nanoparticles are nearly
70%, 85% and 60% effective compare to Kanamycin and
Arithromycin respectively. It is observed that the pres-
ence of silver nanoparticles inhibited bacterial growth by
more than 97%.
The mechanism of the bactericidal effect of silver na-
noparticles is not very well-known. It is believed that
cellular proteins become inactive after treatment with
silver nanoparticles [12]. It is also believed that silver
nanoparticles after penetration into the bacteria have in-
activated their enzymes, generating hydrogen peroxide
and caused bacterial cell death [11]. Heavy metals are
toxic and react with proteins, therefore they bind protein
molecules; as a result cellular metabolism is inhibited
causing death of microorganism [12]. It is known that
silver sources such as silver nitrate and silver sulfadiaz-
ine release Ag+ only [12] but high activity of silver
nanoparticles is attributed to the release of Ag0 and Ag+
clusters when they dissolve [12]. Our experimental result
shows that linoleic acid capped silver nanoparticles can
be used as effective growth inhibitors in various micro-
Figure 4. Shows silver nanoparticles laden disk (a) and an-
tibiotic impregnated disk (b, c) placed on the surface of the
Staphylococcus Basillus(1) and Staphylloccoccus Aureus(2)
bacterial suspension on Muller Hinton agar (MHA) plate
after incubation at 35˚C for 24 h.
organisms, making them applicable to diverse medical
medicines and antimicrobial control systems.
4. Discussion
Uniform linoleic acid capped silver nanoparticles have
been prepared through the reduction of silver ions by
ethanol. TEM micrograph reveals that the prepared nano-
particles are spherical in shape with average size of 12
nm having nearly uniform distribution and FTIR spectra
confirms the capping of linoleic acid on nanoparticles
surfaces. These linoleic acid capped silver nanoparticles
are tested for its antimicrobial activity and the result
shows that silver nanoparticles can be used as effective
growth inhibitors in various microorganisms thereby
applicable to diverse medical devices.
5. Acknowledgements
Authors thanks to Dr B. Dkhar (S.O.), NEHU, Shillong,
India, Dr. A. K. Paul, NEHU, Shillong, India, and Prof.
Arun Chottapadhay, IIT, Guwahati, Assam, India for
their suggestions and assistance during the work.
REFERENCES
[1] D. L. Feldheim and C. A. Foss, “Metal nanoparticles:
Synthesis, Characterization and Applications,” Marcel
Dekker Inc., New York, 2002.
[2] G. Cao, “Nanostructures and Nanomaterials,” Edited by
Imperial College Press, London, 2004.
[3] C. P. Poole and F. J. Owens, “Introduction to Nanotech-
nology,” Edited by Wiley Interscience Publication, New
Jersey, 2005.
[4] M. Brust, M. Walker, D. Bethell, D. J. Schiffrin and R. J.
Whyman, “Synthesis of Thiol Derivatised Gold Nano-
particles in a Two Phase Liquid/Liquid System,” Journal
of the Chemical Society, Chemical Communications, Vol.
7, No. 7, 1994, pp. 801-802. doi:10.1039/c39940000801
[5] A. S. Nair and T. Y. Pradeep, “Halocarbon Mineraliza-
tion and Catalytic Destruction by Metal Nanoparticles,”
Current Science, Vol. 84, No. 12, 2003, pp. 1560-1564.
[6] Y. Fang, “Optical Absorption of Nanoscale Colloidal Sil-
ver: Aggregate Band and Adsorbate-Silver Surface Ban d,”
Journal of Physical Chemistry, Vol. 108, No. 10, 1998, pp.
4315-4318. doi:10.1063/1.475831
[7] X. Wang, J. Zhuang, Q. Peng and Y. Li, “A General Stra-
tegy for Nanocrystal Synthesis,” Nature, Vol. 437, No.
7055, 2005, pp. 121-124. doi:10.1038/nature03968
[8] M. D. Porter, T. B. Bright, D. L. Allara, and C. E. D. Chi-
dsey, “Chemical Functionality in Self-Assembled Mono-
layers and Electrochemistry,” Journal of the American
Chemical Society, Vol. 109, No. 12, 1987, pp. 3559- 3568.
doi:10.1021/ja00246a011
[9] M. Bahadory, “Synthesis of Noble Metal Nanoparticles,”
Dissertation, Drexel University, Philadelphia, 2008.
[10] S. Pal, Y. Kyung and J. M. Song, “Does the Antibacterial
C
opyright © 2011 SciRes. JBNB