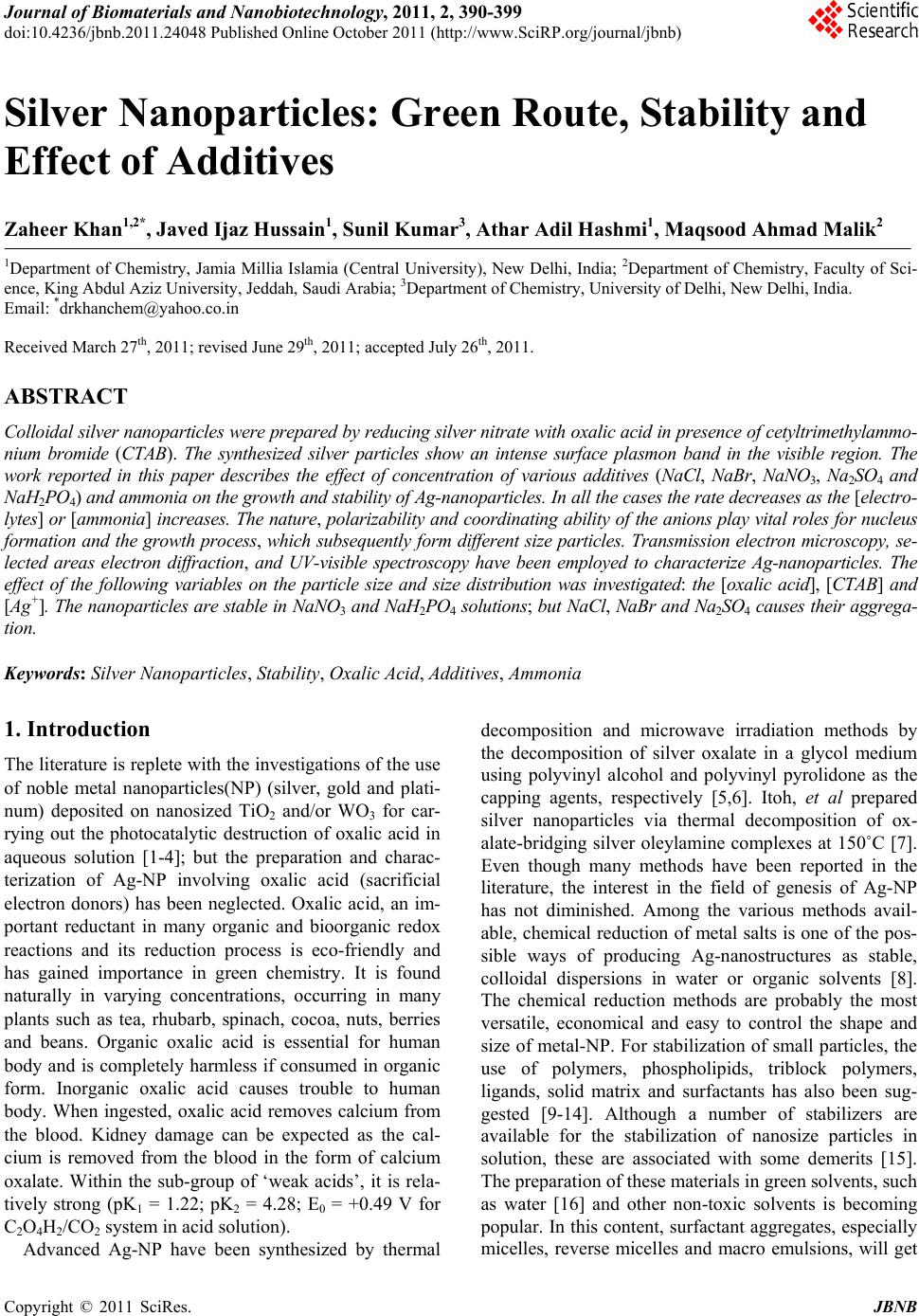 Journal of Biomaterials and Nanobiotechnology, 2011, 2, 390-399 doi:10.4236/jbnb.2011.24048 Published Online October 2011 (http://www.SciRP.org/journal/jbnb) Copyright © 2011 SciRes. JBNB Silver Nanoparticles: Green Route, Stability and Effect of Additives Zaheer Khan1,2*, Javed Ijaz Hussain1, Sunil Kumar3, Athar Adil Hashmi1, Maqsood Ahmad Malik2 1Department of Chemistry, Jamia Millia Islamia (Central University), New Delhi, India; 2Department of Chemistry, Faculty of Sci- ence, King Abdul Aziz University, Jeddah, Saudi Arabia; 3Department of Chemistry, University of Delhi, New Delhi, India. Email: *drkhanchem@yahoo.co.in Received March 27th, 2011; revised June 29th, 2011; accepted July 26th, 2011. ABSTRACT Colloidal silver nanoparticles were prepared by reducing silver nitrate with oxalic acid in presence of cetyltrimethylammo- nium bromide (CTAB). The synthesized silver particles show an intense surface plasmon band in the visible region. The work reported in this paper describes the effect of concentration of various additives (NaCl, NaBr, NaNO3, Na2SO4 and NaH2PO4) and ammonia on the growth and stability of Ag-nanoparticles. In all the cases the rate decreases as the [electro- lytes] or [ammonia] increases. The nature, polarizability and coordinating ability of the anions play vital roles for nucleus formation and the growth process, which subsequently form different size particles. Transmission electron microscopy, se- lected areas electron diffraction, and UV-visible spectroscopy have been employed to characterize Ag-nanoparticles. The effect of the following variables on the particle size and size distribution was investigated: the [oxalic acid], [CTAB] and [Ag+]. The nanoparticles are stable in NaNO3 and NaH2PO4 solutions ; bu t NaCl, NaBr and Na2SO4 causes their aggrega- tion. Keywords: Silver Nanoparticles, Stability, Oxalic Acid, Additives, Ammonia 1. Introduction The literature is replete with the investigations of the use of noble metal nanoparticles(NP) (silver, gold and plati- num) deposited on nanosized TiO2 and/or WO3 for car- rying out the photocatalytic destruction of oxalic acid in aqueous solution [1-4]; but the preparation and charac- terization of Ag-NP involving oxalic acid (sacrificial electron donors) has been neglected. Oxalic acid, an im- portant reductant in many organic and bioorganic redox reactions and its reduction process is eco-friendly and has gained importance in green chemistry. It is found naturally in varying concentrations, occurring in many plants such as tea, rhubarb, spinach, cocoa, nuts, berries and beans. Organic oxalic acid is essential for human body and is completely harmless if consumed in organic form. Inorganic oxalic acid causes trouble to human body. When ingested, oxalic acid removes calcium from the blood. Kidney damage can be expected as the cal- cium is removed from the blood in the form of calcium oxalate. Within the sub-group of ‘weak acids’, it is rela- tively strong (pK1 = 1.22; pK2 = 4.28; E0 = +0.49 V for C2O4H2/CO2 system in acid solution). Advanced Ag-NP have been synthesized by thermal decomposition and microwave irradiation methods by the decomposition of silver oxalate in a glycol medium using polyvinyl alcohol and polyvinyl pyrolidone as the capping agents, respectively [5,6]. Itoh, et al prepared silver nanoparticles via thermal decomposition of ox- alate-bridging silver oleylamine complexes at 150˚C [7]. Even though many methods have been reported in the literature, the interest in the field of genesis of Ag-NP has not diminished. Among the various methods avail- able, chemical reduction of metal salts is one of the pos- sible ways of producing Ag-nanostructures as stable, colloidal dispersions in water or organic solvents [8]. The chemical reduction methods are probably the most versatile, economical and easy to control the shape and size of metal-NP. For stabilization of small particles, the use of polymers, phospholipids, triblock polymers, ligands, solid matrix and surfactants has also been sug- gested [9-14]. Although a number of stabilizers are available for the stabilization of nanosize particles in solution, these are associated with some demerits [15]. The preparation of these materials in green solvents, such as water [16] and other non-toxic solvents is becoming popular. In this content, surfactant aggregates, especially micelles, reverse micelles and macro emulsions, will get
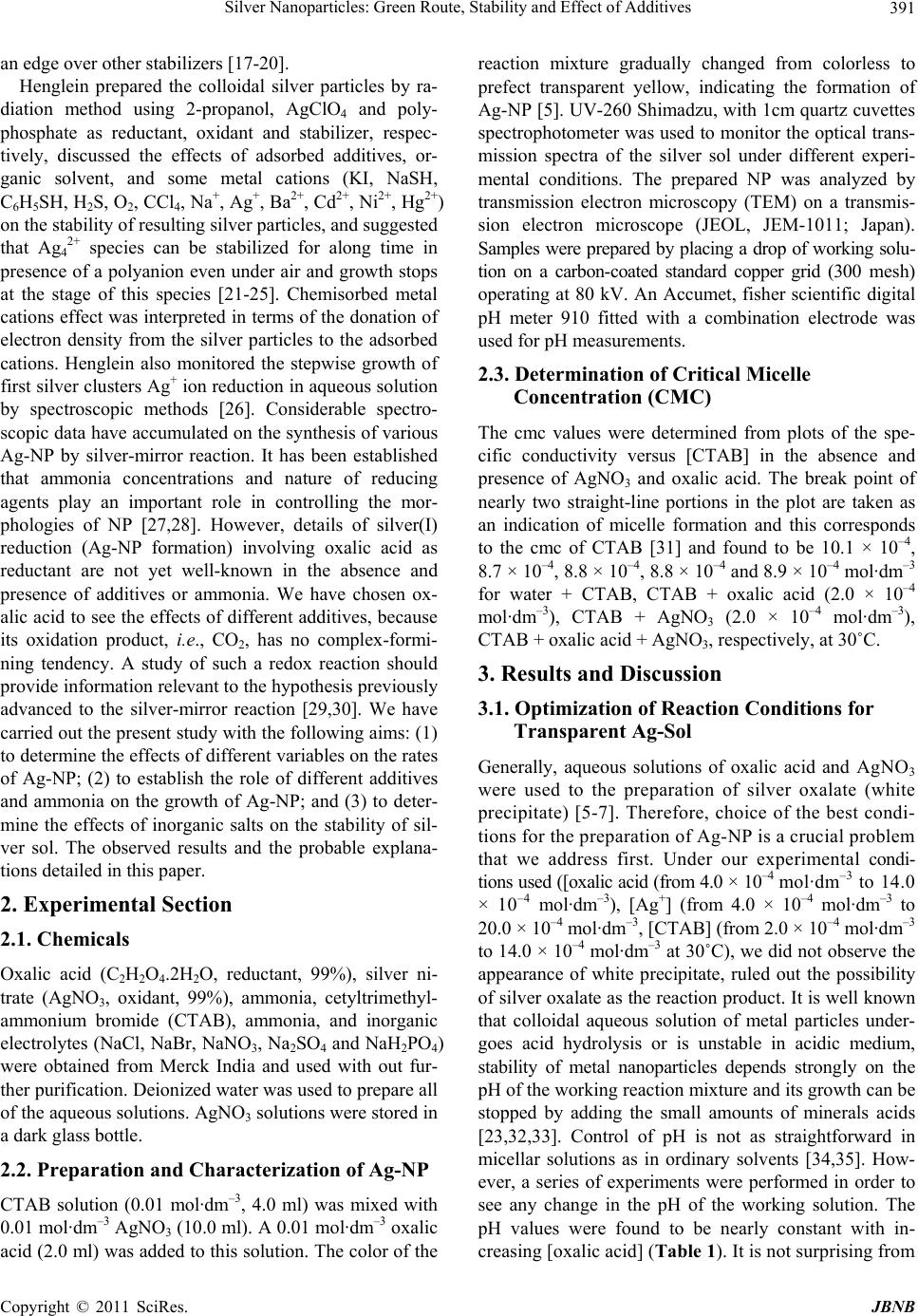 Silver Nanoparticles: Green Route, Stability and Effect of Additives391 an edge over other stabilizers [17-20]. Henglein prepared the colloidal silver particles by ra- diation method using 2-propanol, AgClO4 and poly- phosphate as reductant, oxidant and stabilizer, respec- tively, discussed the effects of adsorbed additives, or- ganic solvent, and some metal cations (KI, NaSH, C6H5SH, H2S, O2, CCl4, Na+, Ag+, Ba2+, Cd2+, Ni2+, Hg2+) on the stability of resulting silver particles, and suggested that Ag4 2+ species can be stabilized for along time in presence of a polyanion even under air and growth stops at the stage of this species [21-25]. Chemisorbed metal cations effect was interpreted in terms of the donation of electron density from the silver particles to the adsorbed cations. Henglein also monitored the stepwise growth of first silver clusters Ag+ ion reduction in aqueous solution by spectroscopic methods [26]. Considerable spectro- scopic data have accumulated on the synthesis of various Ag-NP by silver-mirror reaction. It has been established that ammonia concentrations and nature of reducing agents play an important role in controlling the mor- phologies of NP [27,28]. However, details of silver(I) reduction (Ag-NP formation) involving oxalic acid as reductant are not yet well-known in the absence and presence of additives or ammonia. We have chosen ox- alic acid to see the effects of different additives, because its oxidation product, i.e., CO2, has no complex-formi- ning tendency. A study of such a redox reaction should provide information relevant to the hypothesis previously advanced to the silver-mirror reaction [29,30]. We have carried out the present study with the following aims: (1) to determine the effects of different variables on the rates of Ag-NP; (2) to establish the role of different additives and ammonia on the growth of Ag-NP; and (3) to deter- mine the effects of inorganic salts on the stability of sil- ver sol. The observed results and the probable explana- tions detailed in this paper. 2. Experimental Section 2.1. Chemicals Oxalic acid (C2H2O4.2H2O, reductant, 99%), silver ni- trate (AgNO3, oxidant, 99%), ammonia, cetyltrimethyl- ammonium bromide (CTAB), ammonia, and inorganic electrolytes (NaCl, NaBr, NaNO3, Na2SO4 and NaH2PO4) were obtained from Merck India and used with out fur- ther purification. Deionized water was used to prepare all of the aqueous solutions. AgNO3 solutions were stored in a dark glass bottle. 2.2. Preparation and Characterization of Ag-NP CTAB solution (0.01 mol·dm–3, 4.0 ml) was mixed with 0.01 mol·dm–3 AgNO3 (10.0 ml). A 0.01 mol·dm–3 oxalic acid (2.0 ml) was added to this solution. The color of the reaction mixture gradually changed from colorless to prefect transparent yellow, indicating the formation of Ag-NP [5]. UV-260 Shimadzu, with 1cm quartz cuvettes spectrophotometer was used to monitor the optical trans- mission spectra of the silver sol under different experi- mental conditions. The prepared NP was analyzed by transmission electron microscopy (TEM) on a transmis- sion electron microscope (JEOL, JEM-1011; Japan). Samples were prepared by placing a drop of working solu- tion on a carbon-coated standard copper grid (300 mesh) operating at 80 kV. An Accumet, fisher scientific digital pH meter 910 fitted with a combination electrode was used for pH measurements. 2.3. Determination of Critical Micelle Concentration (CMC) The cmc values were determined from plots of the spe- cific conductivity versus [CTAB] in the absence and presence of AgNO3 and oxalic acid. The break point of nearly two straight-line portions in the plot are taken as an indication of micelle formation and this corresponds to the cmc of CTAB [31] and found to be 10.1 × 10–4, 8.7 × 10–4, 8.8 × 10–4, 8.8 × 10–4 and 8.9 × 10–4 mol·dm–3 for water + CTAB, CTAB + oxalic acid (2.0 × 10–4 mol·dm–3), CTAB + AgNO3 (2.0 × 10–4 mol·dm–3), CTAB + oxalic acid + AgNO3, respectively, at 30˚C. 3. Results and Discussion 3.1. Optimization of Reaction Conditions for Transparent Ag-Sol Generally, aqueous solutions of oxalic acid and AgNO3 were used to the preparation of silver oxalate (white precipitate) [5-7]. Therefore, choice of the best condi- tions for the preparation of Ag-NP is a crucial problem that we address first. Under our experimental condi- tions used ([oxalic acid (from 4.0 × 10–4 mol·dm–3 to 14.0 × 10–4 mol·dm–3), [Ag+] (from 4.0 × 10–4 mol·dm–3 to 20.0 × 10–4 mol·dm–3, [CTAB] (from 2.0 × 10–4 mol·dm–3 to 14.0 × 10–4 mol·dm–3 at 30˚C), we did not observe the appearance of white precipitate, ruled out the possibility of silver oxalate as the reaction product. It is well known that colloidal aqueous solution of metal particles under- goes acid hydrolysis or is unstable in acidic medium, stability of metal nanoparticles depends strongly on the pH of the working reaction mixture and its growth can be stopped by adding the small amounts of minerals acids [23,32,33]. Control of pH is not as straightforward in micellar solutions as in ordinary solvents [34,35]. How- ever, a series of experiments were performed in order to see any change in the pH of the working solution. The pH values were found to be nearly constant with in- creasing [oxalic acid] (Table 1). It is not surprising from Copyright © 2011 SciRes. JBNB
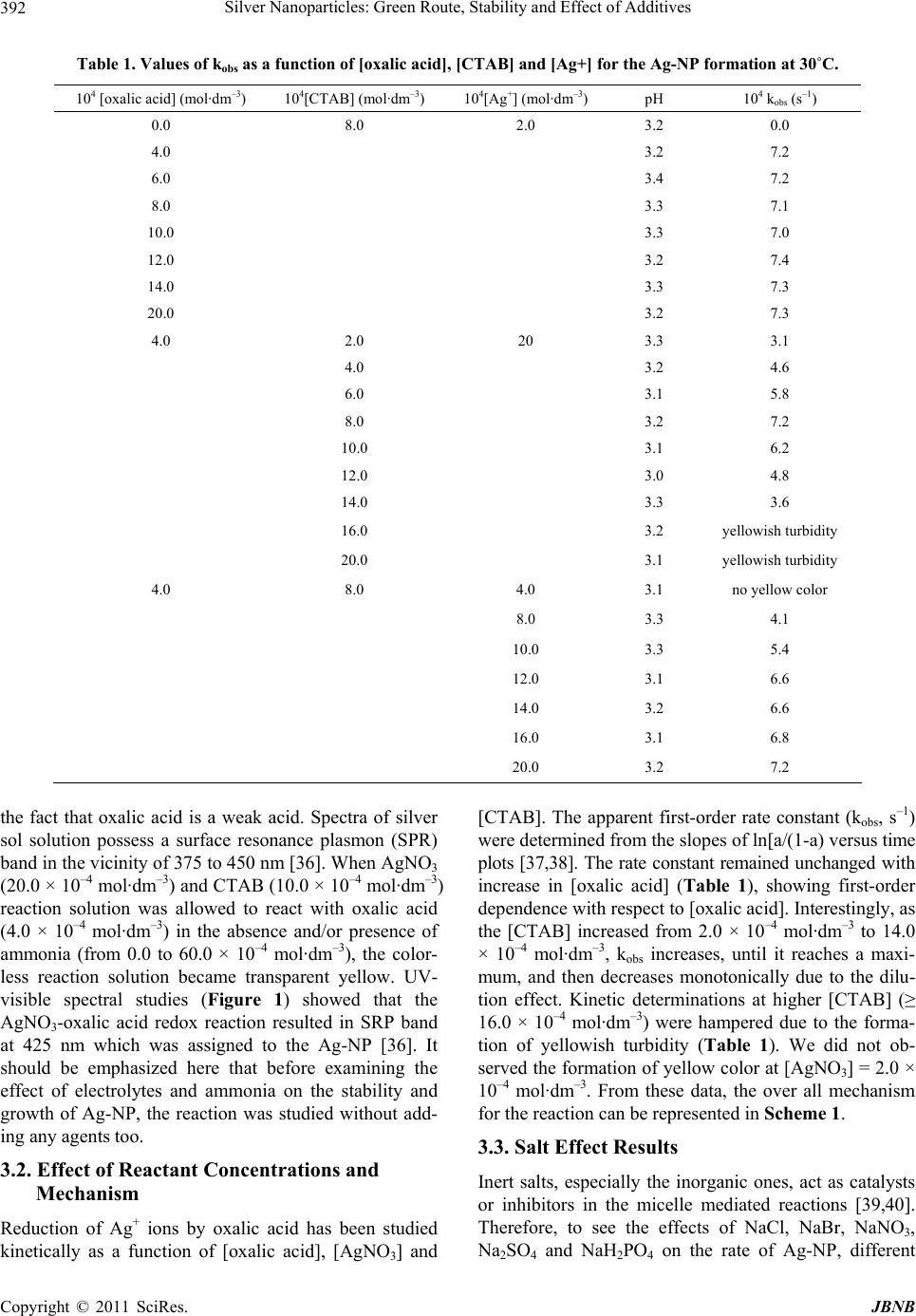 Silver Nanoparticles: Green Route, Stability and Effect of Additives Copyright © 2011 SciRes. JBNB 392 Table 1. Values of kobs as a function of [oxalic acid], [CTAB] and [Ag+] for the Ag-NP formation at 30˚C. 104 [oxalic acid] (mol·dm–3) 104[CTAB] (mol·dm–3) 104[Ag+] (mol·dm–3) pH 104 kobs (s–1) 0.0 8.0 2.0 3.2 0.0 4.0 3.2 7.2 6.0 3.4 7.2 8.0 3.3 7.1 10.0 3.3 7.0 12.0 3.2 7.4 14.0 3.3 7.3 20.0 3.2 7.3 4.0 2.0 20 3.3 3.1 4.0 3.2 4.6 6.0 3.1 5.8 8.0 3.2 7.2 10.0 3.1 6.2 12.0 3.0 4.8 14.0 3.3 3.6 16.0 3.2 yellowish turbidity 20.0 3.1 yellowish turbidity 4.0 8.0 4.0 3.1 no yellow color 8.0 3.3 4.1 10.0 3.3 5.4 12.0 3.1 6.6 14.0 3.2 6.6 16.0 3.1 6.8 20.0 3.2 7.2 the fact that oxalic acid is a weak acid. Spectra of silver sol solution possess a surface resonance plasmon (SPR) band in the vicinity of 375 to 450 nm [36]. When AgNO3 (20.0 × 10–4 mol·dm–3) and CTAB (10.0 × 10–4 mol·dm–3) reaction solution was allowed to react with oxalic acid (4.0 × 10–4 mol·dm–3) in the absence and/or presence of ammonia (from 0.0 to 60.0 × 10–4 mol·dm–3), the color- less reaction solution became transparent yellow. UV- visible spectral studies (Figure 1) showed that the AgNO3-oxalic acid redox reaction resulted in SRP band at 425 nm which was assigned to the Ag-NP [36]. It should be emphasized here that before examining the effect of electrolytes and ammonia on the stability and growth of Ag-NP, the reaction was studied without add- ing any agents too. 3.2. Effect of Reactant Concentrations and Mechanism Reduction of Ag+ ions by oxalic acid has been studied kinetically as a function of [oxalic acid], [AgNO3] and [CTAB]. The apparent first-order rate constant (kobs, s–1) were determined from the slopes of ln[a/(1-a) versus time plots [37,38]. The rate constant remained unchanged with increase in [oxalic acid] (Table 1), showing first-order dependence with respect to [oxalic acid]. Interestingly, as the [CTAB] increased from 2.0 × 10–4 mol·dm–3 to 14.0 × 10–4 mol·dm–3, kobs increases, until it reaches a maxi- mum, and then decreases monotonically due to the dilu- tion effect. Kinetic determinations at higher [CTAB] (≥ 16.0 × 10–4 mol·dm–3) were hampered due to the forma- tion of yellowish turbidity (Table 1). We did not ob- served the formation of yellow color at [AgNO3] = 2.0 × 10–4 mol·dm–3. From these data, the over all mechanism for the reaction can be represented in Scheme 1. 3.3. Salt Effect Results Inert salts, especially the inorganic ones, act as catalysts or inhibitors in the micelle mediated reactions [39,40]. Therefore, to see the effects of NaCl, NaBr, NaNO3, Na2SO4 and NaH2PO 4 on the rate of Ag-NP, different
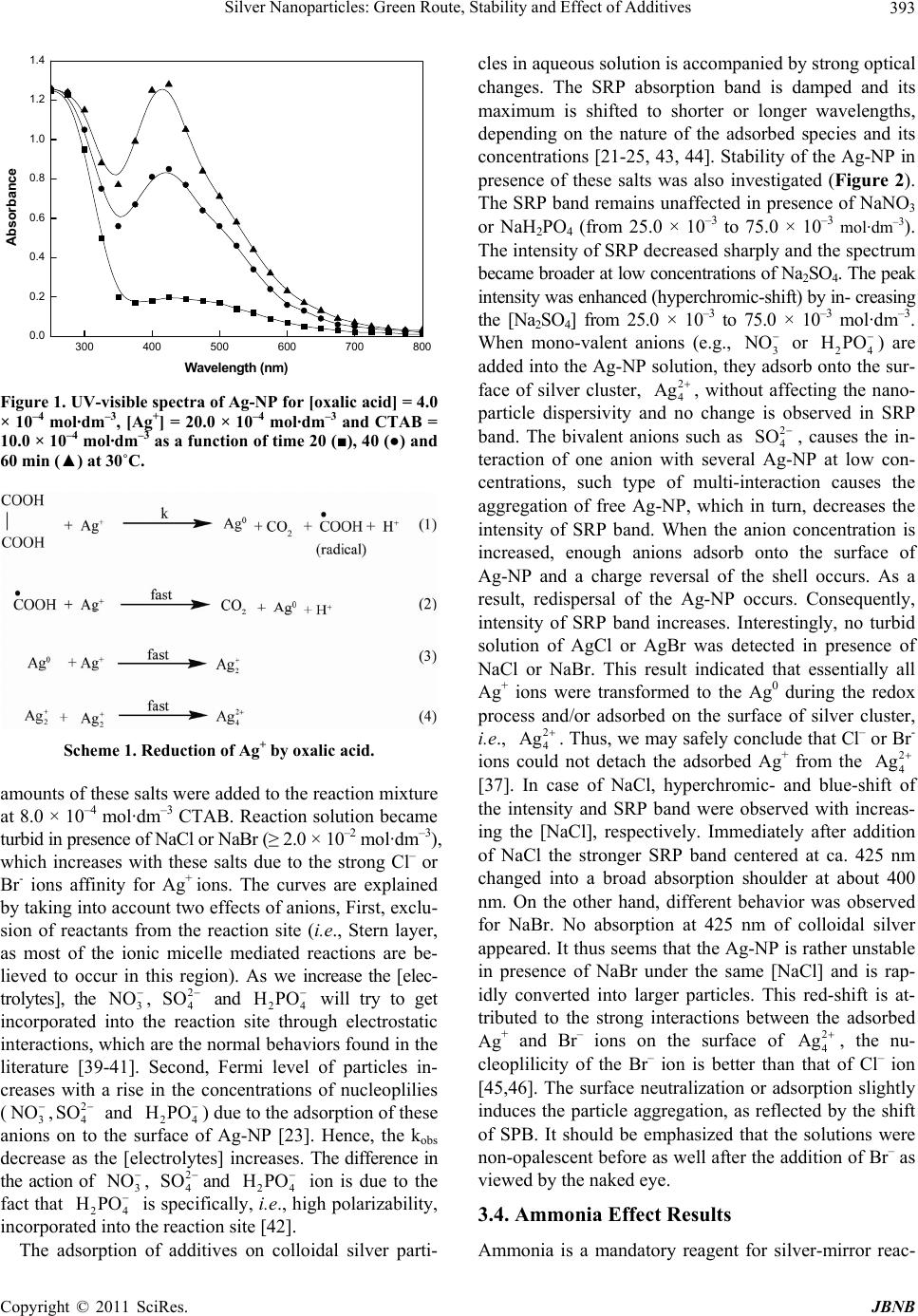 Silver Nanoparticles: Green Route, Stability and Effect of Additives393 300 400 500 600 700 800 0.0 0.2 0.4 0.6 0.8 1.0 1.2 1.4 Absorbance Wav elength (nm) Figure 1. UV-visible spectra of Ag-NP for [oxalic acid] = 4.0 × 10–4 mol·dm–3, [Ag+] = 20.0 × 10–4 mol·dm–3 and CTAB = 10.0 × 10–4 mol·dm–3 as a function of time 20 (■), 40 (●) and 60 min (▲) at 30˚C. Scheme 1. Reduction of Ag+ by oxalic acid. amounts of these salts were added to the reaction mixture at 8.0 × 10–4 mol·dm–3 CTAB. Reaction solution became turbid in presence of NaCl or NaBr (≥ 2.0 × 10–2 mol·dm–3), which increases with these salts due to the strong Cl– or Br- ions affinity for Ag+ ions. The curves are explained by taking into account two effects of anions, First, exclu- sion of reactants from the reaction site (i.e., Stern layer, as most of the ionic micelle mediated reactions are be- lieved to occur in this region). As we increase the [elec- trolytes], the 3, and 24 will try to get incorporated into the reaction site through electrostatic interactions, which are the normal behaviors found in the literature [39-41]. Second, Fermi level of particles in- creases with a rise in the concentrations of nucleoplilies (3, and 24 ) due to the adsorption of these anions on to the surface of Ag-NP [23]. Hence, the kobs decrease as the [electrolytes] increases. The difference in the action of 3, and 24 ion is due to the fact that 24 is specifically, i.e., high polarizability, incorporated into the reaction site [42]. NO NO O 2 4 SO HPO 2 4 SO HPO HPO NO2 4 SO HP The adsorption of additives on colloidal silver parti- cles in aqueous solution is accompanied by strong optical changes. The SRP absorption band is damped and its maximum is shifted to shorter or longer wavelengths, depending on the nature of the adsorbed species and its concentrations [21-25, 43, 44]. Stability of the Ag-NP in presence of these salts was also investigated (Figure 2). The SRP band remains unaffected in presence of NaNO3 or NaH2PO4 (from 25.0 × 10–3 to 75.0 × 10–3 mol· dm–3). The intensity of SRP decreased sharply and the spectrum became broader at low concentrations of Na2SO4. The peak intensity was enhanced (hyperchromic-shift) by in- creasing the [Na2SO4] from 25.0 × 10–3 to 75.0 × 10–3 mol·dm–3. When mono-valent anions (e.g., 3 or 24 NOHPO ) are added into the Ag-NP solution, they adsorb onto the sur- face of silver cluster, 2 4 Ag , without affecting the nano- particle dispersivity and no change is observed in SRP band. The bivalent anions such as , causes the in- teraction of one anion with several Ag-NP at low con- centrations, such type of multi-interaction causes the aggregation of free Ag-NP, which in turn, decreases the intensity of SRP band. When the anion concentration is increased, enough anions adsorb onto the surface of Ag-NP and a charge reversal of the shell occurs. As a result, redispersal of the Ag-NP occurs. Consequently, intensity of SRP band increases. Interestingly, no turbid solution of AgCl or AgBr was detected in presence of NaCl or NaBr. This result indicated that essentially all Ag+ ions were transformed to the Ag0 during the redox process and/or adsorbed on the surface of silver cluster, i.e., 2 4 SO 2 4 Ag . Thus, we may safely conclude that Cl– or Br- ions could not detach the adsorbed Ag+ from the 2 4 Ag [37]. In case of NaCl, hyperchromic- and blue-shift of the intensity and SRP band were observed with increas- ing the [NaCl], respectively. Immediately after addition of NaCl the stronger SRP band centered at ca. 425 nm changed into a broad absorption shoulder at about 400 nm. On the other hand, different behavior was observed for NaBr. No absorption at 425 nm of colloidal silver appeared. It thus seems that the Ag-NP is rather unstable in presence of NaBr under the same [NaCl] and is rap- idly converted into larger particles. This red-shift is at- tributed to the strong interactions between the adsorbed Ag+ and Br– ions on the surface of Ag2 4 , the nu- cleoplilicity of the Br– ion is better than that of Cl– ion [45,46]. The surface neutralization or adsorption slightly induces the particle aggregation, as reflected by the shift of SPB. It should be emphasized that the solutions were non-opalescent before as well after the addition of Br– as viewed by the naked eye. 3.4. Ammonia Effect Results Ammonia is a mandatory reagent for silver-mirror reac- Copyright © 2011 SciRes. JBNB
 Silver Nanoparticles: Green Route, Stability and Effect of Additives Copyright © 2011 SciRes. JBNB 394 300 400 500 600700 0.0 0.1 0.2 0.3 0.4 0.5 Absorbance Wa v e len gth (nm) [NaCl](mol dm-3) 0.0 0.025 0.050 0.075 300 400 500 600 700 0.0 0.1 0.2 0.3 Absorbance W a v e le n g th (n m) [NaNO3] (mol dm-3) 0.0 0.025 0.050 0.075 300 400 500 600 700 0.0 0.1 0.2 0.3 0.4 Absorbance W a vele n g th (n m ) [Na2SO4] (mol dm-3) 0.0 0.025 0.050 0.075 300 400 500 600 700 0.0 0.1 0.2 0.3 Absorbance Wav elength (nm) [NaH2PO4] (mol dm-3) 0.0 0.025 0.050 0.075 300 400 500 600 700 0.0 0.1 0.2 0.3 0.4 0.5 104[NaBr] (mol dm-3) 0.0 0.025 0.050 0.075 Absorbance Wav elength (nm) Figure 2. Effect of [electrolytes] on the surface plasma resonance band of Ag-NP. The concentration of Ag-NP was diluted from the original solution by 4 times. tion and their concentration play a major role in control- ling the Ag-NP size and shape [27,47-49]. In order to gain insight into the role of ammonia, the oxalic acid- silver(I) reaction was also studied in presence of [ammo- nia]. The evolution of the absorption spectra with time is presented in Figure 3 in an ammonia solution containing 4.0 × 10–4 mol·dm–3 oxalic acid, 20.0 × 10–4 mol·dm–3 AgNO3 and 10.0 × 10–4 mol·dm–3 CTAB. As shown in Figure 1 the SRP band centered at about 425 nm de- creased in intensity and shifted to shorter wavelengths with increasing time (blue shift of about 15 nm). It seemed possible that the decrease in the intensity was related to the complexation of ammonia with Ag+ ions during the course of reaction. To conform this hypothesis,
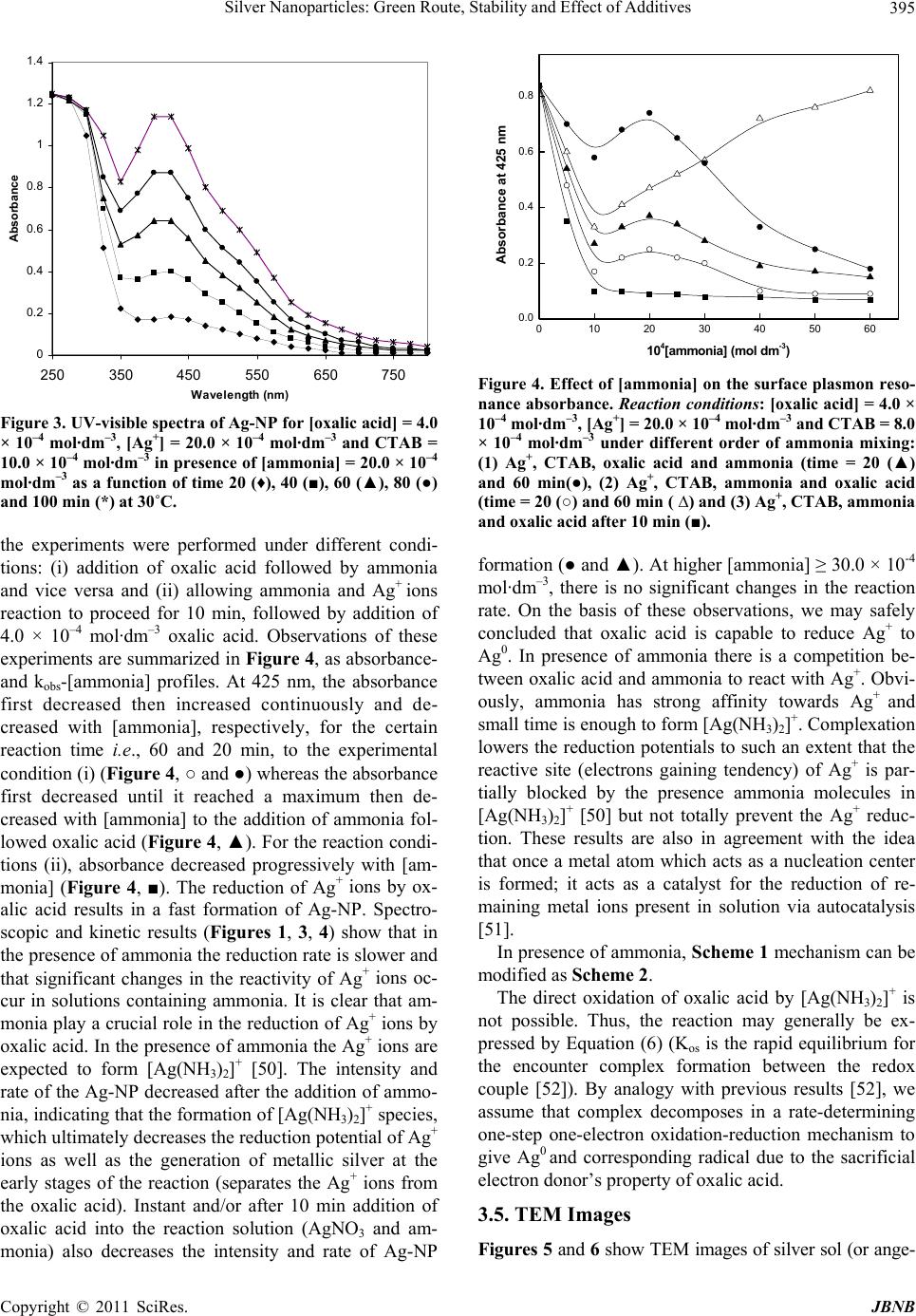 Silver Nanoparticles: Green Route, Stability and Effect of Additives395 0 0.2 0.4 0.6 0.8 1 1.2 1.4 250 350450 550 650 750 Wave length (n m) Absorbance Figure 3. UV-visible spectra of Ag-NP for [oxalic acid] = 4.0 × 10–4 mol·dm–3, [Ag+] = 20.0 × 10–4 mol·dm–3 and CTAB = 10.0 × 10–4 mol·dm–3 in presence of [ammonia] = 20.0 × 10–4 mol·dm–3 as a function of time 20 (♦), 40 (■), 60 (▲), 80 (●) and 100 min (*) at 30˚C. the experiments were performed under different condi- tions: (i) addition of oxalic acid followed by ammonia and vice versa and (ii) allowing ammonia and Ag+ ions reaction to proceed for 10 min, followed by addition of 4.0 × 10–4 mol·dm–3 oxalic acid. Observations of these experiments are summarized in Figure 4, as absorbance- and kobs-[ammonia] profiles. At 425 nm, the absorbance first decreased then increased continuously and de- creased with [ammonia], respectively, for the certain reaction time i.e., 60 and 20 min, to the experimental condition (i) (Figure 4, ○ and ●) whereas the absorbance first decreased until it reached a maximum then de- creased with [ammonia] to the addition of ammonia fol- lowed oxalic acid (Figure 4, ▲). For the reaction condi- tions (ii), absorbance decreased progressively with [am- monia] (Figure 4, ■). The reduction of Ag+ ions by ox- alic acid results in a fast formation of Ag-NP. Spectro- scopic and kinetic results (Figures 1, 3, 4) show that in the presence of ammonia the reduction rate is slower and that significant changes in the reactivity of Ag+ ions oc- cur in solutions containing ammonia. It is clear that am- monia play a crucial role in the reduction of Ag+ ions by oxalic acid. In the presence of ammonia the Ag+ ions are expected to form [Ag(NH3)2]+ [50]. The intensity and rate of the Ag-NP decreased after the addition of ammo- nia, indicating that the formation of [Ag(NH3)2]+ species, which ultimately decreases the reduction potential of Ag+ ions as well as the generation of metallic silver at the early stages of the reaction (separates the Ag+ ions from the oxalic acid). Instant and/or after 10 min addition of oxalic acid into the reaction solution (AgNO3 and am- 0 102030405060 0.0 0.2 0.4 0.6 0.8 Absorbance at 425 nm 104[amm onia] (mol dm-3) Figure 4. Effect of [ammonia] on the surface plasmon res rmation (● and ▲). At higher [ammonia] ≥ 30.0 × 10-4 sence of ammonia, Scheme 1 mechanism can be m oxalic acid by [Ag(NH3)2] is no TEM images of silver sol (or ange- o- nance absorbance. Reaction conditions: [oxalic acid] = 4.0 × 10–4 mol·dm–3, [Ag+] = 20.0 × 10–4 mol·dm–3 and CTAB = 8.0 × 10–4 mol·dm–3 under different order of ammonia mixing: (1) Ag+, CTAB, oxalic acid and ammonia (time = 20 (▲) and 60 min(●), (2) Ag+, CTAB, ammonia and oxalic acid (time = 20 (○) and 60 min ( ∆) and (3) Ag+, CTAB, ammonia and oxalic acid after 10 min (■). fo mol·dm–3, there is no significant changes in the reaction rate. On the basis of these observations, we may safely concluded that oxalic acid is capable to reduce Ag+ to Ag0. In presence of ammonia there is a competition be- tween oxalic acid and ammonia to react with Ag+. Obvi- ously, ammonia has strong affinity towards Ag+ and small time is enough to form [Ag(NH3)2]+. Complexation lowers the reduction potentials to such an extent that the reactive site (electrons gaining tendency) of Ag+ is par- tially blocked by the presence ammonia molecules in [Ag(NH3)2]+ [50] but not totally prevent the Ag+ reduc- tion. These results are also in agreement with the idea that once a metal atom which acts as a nucleation center is formed; it acts as a catalyst for the reduction of re- maining metal ions present in solution via autocatalysis [51]. In pre odified as Scheme 2. The direct oxidation of + t possible. Thus, the reaction may generally be ex- pressed by Equation (6) (Kos is the rapid equilibrium for the encounter complex formation between the redox couple [52]). By analogy with previous results [52], we assume that complex decomposes in a rate-determining one-step one-electron oxidation-reduction mechanism to give Ag0 and corresponding radical due to the sacrificial electron donor’s property of oxalic acid. 3.5. TEM Images Figures 5 and 6 show monia) also decreases the intensity and rate of Ag-NP Copyright © 2011 SciRes. JBNB
 Silver Nanoparticles: Green Route, Stability and Effect of Additives Copyright © 2011 SciRes. JBNB 396 Scheme 2. Oxidation of oxalic acid by Ag-ammonia complex. Figure 5. TEM images of Ag-NP. Reaction conditions: [oxalic acid] = 4.0 × 10–4 mol·dm–3, [Ag+] = 20.0 × 10–4 mol·dm–3 and CTAB = 10.0 × 10–4 mol·dm–3. Figure 6. TEM images of Ag-NP. Reaction conditions: [oxalic acid] = 4.0 × 10–4 mol·dm–3, [Ag+] = 20.0 × 10–4 mol·dm–3 and CTAB = 10.0 × 10–4 mol·dm–3 in presence of [ammonia] = 20.0 × 10-4 mol·dm–3.
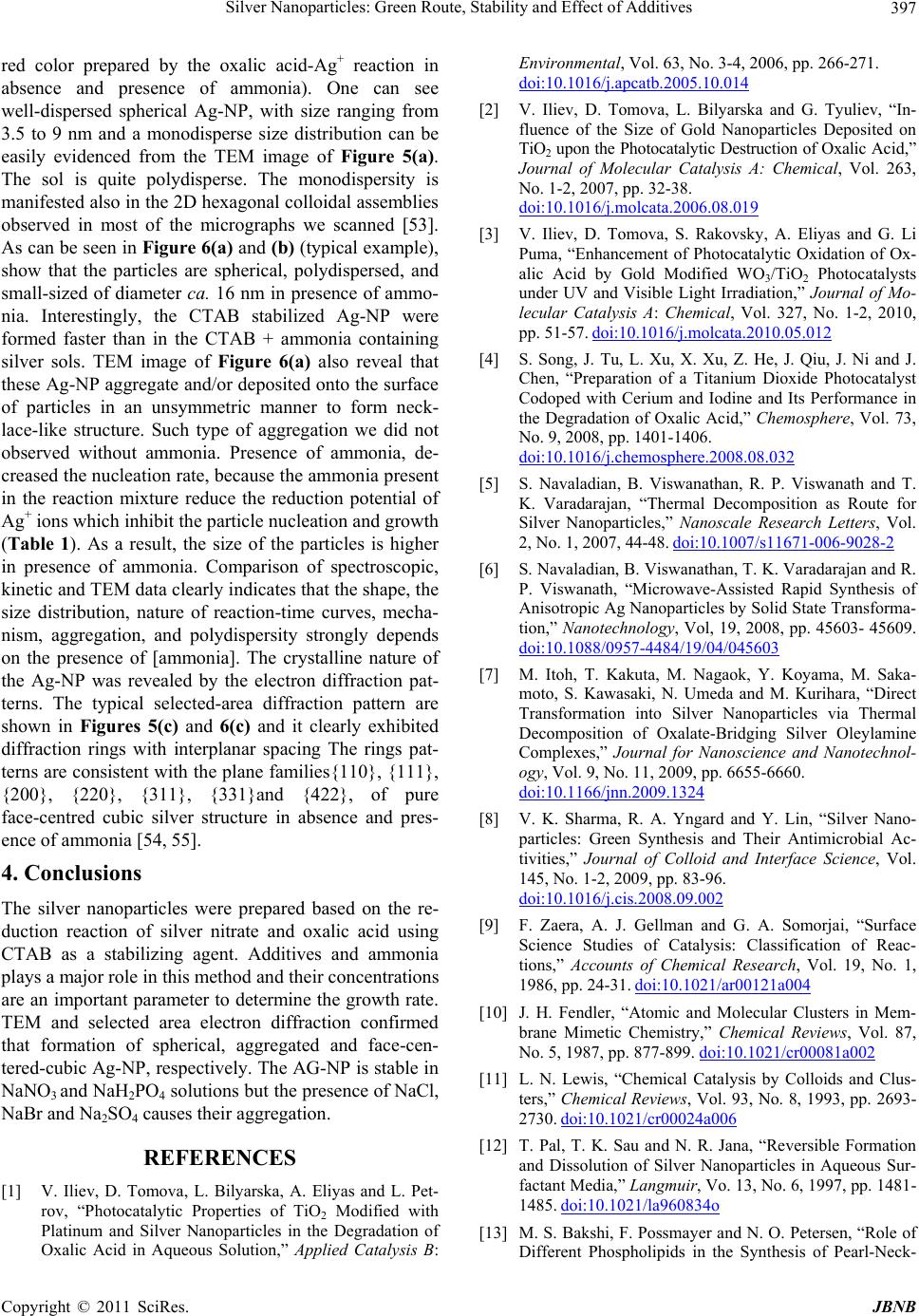 Silver Nanoparticles: Green Route, Stability and Effect of Additives397 icles were prepared based on the re- [1] V. Iliev, D. T Eliyas and L. Pet- ental, Vol. 63, No. 3-4, 2006, pp. 266-271. red color prepared by the oxalic acid-Ag+ reaction in Environm absence and presence of ammonia). One can see well-dispersed spherical Ag-NP, with size ranging from 3.5 to 9 nm and a monodisperse size distribution can be easily evidenced from the TEM image of Figure 5(a). The sol is quite polydisperse. The monodispersity is manifested also in the 2D hexagonal colloidal assemblies observed in most of the micrographs we scanned [53]. As can be seen in Figure 6(a) and (b) (typical example), show that the particles are spherical, polydispersed, and small-sized of diameter ca. 16 nm in presence of ammo- nia. Interestingly, the CTAB stabilized Ag-NP were formed faster than in the CTAB + ammonia containing silver sols. TEM image of Figure 6(a) also reveal that these Ag-NP aggregate and/or deposited onto the surface of particles in an unsymmetric manner to form neck- lace-like structure. Such type of aggregation we did not observed without ammonia. Presence of ammonia, de- creased the nucleation rate, because the ammonia present in the reaction mixture reduce the reduction potential of Ag+ ions which inhibit the particle nucleation and growth (Table 1). As a result, the size of the particles is higher in presence of ammonia. Comparison of spectroscopic, kinetic and TEM data clearly indicates that the shape, the size distribution, nature of reaction-time curves, mecha- nism, aggregation, and polydispersity strongly depends on the presence of [ammonia]. The crystalline nature of the Ag-NP was revealed by the electron diffraction pat- terns. The typical selected-area diffraction pattern are shown in Figures 5(c) and 6(c) and it clearly exhibited diffraction rings with interplanar spacing The rings pat- terns are consistent with the plane families{110}, {111}, {200}, {220}, {311}, {331}and {422}, of pure face-centred cubic silver structure in absence and pres- ence of ammonia [54, 55]. 4. Conclusions The silver nanopart duction reaction of silver nitrate and oxalic acid using CTAB as a stabilizing agent. Additives and ammonia plays a major role in this method and their concentrations are an important parameter to determine the growth rate. TEM and selected area electron diffraction confirmed that formation of spherical, aggregated and face-cen- tered-cubic Ag-NP, respectively. The AG-NP is stable in NaNO3 and NaH2PO4 solutions but the presence of NaCl, NaBr and Na2SO4 causes their aggregation. REFERENCES omova, L. Bilyarska, A. rov, “Photocatalytic Properties of TiO2 Modified with Platinum and Silver Nanoparticles in the Degradation of Oxalic Acid in Aqueous Solution,” Applied Catalysis B: doi:10.1016/j.apcatb.2005.10.014 [2] V. Iliev, D. Tomova, L. Bilyarska and G. Tyuliev, “In- fluence of the Size of Gold Nanoparticles Deposited on TiO2 upon the Photocatalytic Destruction of Oxalic Acid,” Journal of Molecular Catalysis A: Chemical, Vol. 263, No. 1-2, 2007, pp. 32-38. doi:10.1016/j.molcata.2006.08.019 [3] V. Iliev, D. Tomova, S. Rakovsky, A. Eliyas and G. Li Puma, “Enhancement of Photocatalytic Oxidation of Ox- alic Acid by Gold Modified WO3/TiO2 Photocatalysts under UV and Visible Light Irradiation,” Journal of Mo- lecular Catalysis A: Chemical, Vol. 327, No. 1-2, 2010, pp. 51-57. doi:10.1016/j.molcata.2010.05.012 [4] S. Song, J. Tu, L. Xu, X. Xu, Z. He, J. Qiu, J. Ni and J. Chen, “Preparation of a Titanium Dioxide Photocatalyst Codoped with Cerium and Iodine and Its Performance in the Degradation of Oxalic Acid,” Chemosphere, Vol. 73, No. 9, 2008, pp. 1401-1406. doi:10.1016/j.chemosphere.2008.08.032 [5] S. Navaladian, B. Viswanathan, R. P. Viswanath and T. K. Varadarajan, “Thermal Decomposition as Route for Silver Nanoparticles,” Nanoscale Research Letters, Vol. 2, No. 1, 2007, 44-48. doi:10.1007/s11671-006-9028-2 [6] S. Navaladian, B. Viswanathan, T. K. Varadarajan and R. P. Viswanath, “Microwave-Assisted Rapid Synthesis of Anisotropic Ag Nanoparticles by Solid State Transforma- tion,” Nanotechnology, Vol, 19, 2008, pp. 45603- 45609. doi:10.1088/0957-4484/19/04/045603 [7] M. Itoh, T. Kakuta, M. Nagaok, Y. Koyama, M. Saka- moto, S. Kawasaki, N. Umeda and M. Kurihara, “Direct Transformation into Silver Nanoparticles via Thermal Decomposition of Oxalate-Bridging Silver Oleylamine Complexes,” Journal for Nanoscience and Nanotechnol- ogy, Vol. 9, No. 11, 2009, pp. 6655-6660. doi:10.1166/jnn.2009.1324 [8] V. K. Sharma, R. A. Yngard and Y. Lin, “Silver Nano- particles: Green Synthesis and Their Antimicrobial Ac- tivities,” Journal of Colloid and Interface Science, Vol. 145, No. 1-2, 2009, pp. 83-96. doi:10.1016/j.cis.2008.09.002 [9] F. Zaera, A. J. Gellman and G. A. Somorjai, “Surface Science Studies of Catalysis: tions,” Accounts of Chemical Classification of Reac- Research, Vol. 19, No. 1, 1986, pp. 24-31. doi:10.1021/ar00121a004 [10] J. H. Fendler, “Atomic and Molecular Clusters in Mem- brane Mimetic Chemistry,” Chemical Reviews, Vol. 87, No. 5, 1987, pp. 877-899. doi:10.1021/cr00081a002 [11] L. N. Lewis, “Chemical Catalysis by Colloids and Clus- ters,” Chemical Reviews, Vol. 93, No. 8, 1993, pp. 2693- 2730. doi:10.1021/cr00024a006 [12] T. Pal, T. K. Sau and N. R. Jana, “Reversible Formation and Dissolution of Silver Nanoparticles in Aqueous Sur- factant Media,” Langmuir, Vo. 13, No. 6, 1997, pp. 1481- 1485. doi:10.1021/la960834o [13] M. S. Bakshi, F. Possmayer and N. O. Petersen, “Role of Different Phospholipids in the Synthesis of Pearl-Neck- Copyright © 2011 SciRes. JBNB
 Silver Nanoparticles: Green Route, Stability and Effect of Additives 398 lace-Type Gold-Silver Bimetallic Nanoparticles as Bio- conjugate Materials,” Journal of Physical Chemistry C, Vol. 111, No. 38, 2007, pp. 14113-14124. doi:10.1021/jp072862t [14] P. Khullar, A. Mahal, V. Singh, T. S. Banipal, G. Kaur and M. S. Bakshi, “How PEO-PPO-PEO Triblock Poly- mer Micelles Control the Synthesis of Gold Temperature and Hydro Nanoparticles: phobic Effects,” Langmuir, Vol, 26, No. 13, 2010, pp. 11363-11371. doi:10.1021/la100734p [15] P. Ball, “When Size Does Matter,” Nature, Vol. 349, No. 6305, 1991, p. 101. doi:10.1038/349101a0 [16] X. Tian, W. Wang and G. Cao, “A F Route for the Synthesis acile Aqueous-Phase of Silver Nanoplates,” Materials Letters, Vol. 61, No. 1, 2007, pp. 130-133. doi:10.1016/j.matlet.2006.04.021 [17] M. P. Pileni, “Reverse Micelles as Microreactors,” Jour- nal of Physical Chemistry, Vol. 97, No. 27, 1993, pp. 6961-6973. doi:10.1021/j100129a008 [18] D. E. Tembe and M. M. Sharma, “Factors Controlling the -253. Stability of Colloid-Stabilized Emulsions: I. An Experi- mental Investigation,” Journal of Colloid and Interface Science, Vol. 157, No. 1, 1993, pp. 244 doi:10.1006/jcis.1993.1182 [19] X. Zhai and S. Efrima, “Chemical and Physical Aspects of Macroemulsions Stabilized by Interfacial Colloids,” Journal of Physical Chemistry, Vol. 100, No pp. 11019-11028. . 26, 1996, 1/jp953171cdoi:10.102 hotobiology [20] S. De, A. Pal, N. R. Jana and T. Pal, “Anion Effect in Linear Silver Nanoparticle Aggregation as Evidenced by Efficient Fluorescence Quenching and SERS Enhance- ment,” Journal of Photochemistry and PA: Chemistry, Vol. 131, No. 1, 2000, pp. 111-123. doi:10.1016/S1010-6030(99)00231-2 [21] P. Mulvaney and A. Henglein, “Long-Lived Nonmetallic Silver Clusters in Aqueous Solution: A Pulse Radiolysis Study of Their Formation,” Journal of Physical try, Vol. 94, No. 10, 1990, pp. 4182-41 Chemis 88. - doi:10.1021/j100373a056 [22] T. Linnert, P. Mulvaney and A. Henglein, “Surface Chemistry of Colloidal Silver: Surface Plasmon Damping by Chemisorbed Iodide, Hydrosulfide (SH- thiolate,” Journal of Physi), and Phen cal Chemistry, Vol. 97, No. 3, yl- 1993, pp. 679-682. doi:10.1021/j100105a024 [23] A. Henglein, “Physicochemical Properties of Small Metal Particles in Solution: “Microelectrode” Reactions, Chemi- sorption, Composite Metal Particles, and the Atom-to- Metal Transition,” Journal of Physical Chemistry, Vol. 97, No. 21, 1993, pp. 5457-5471. doi:10.1021/j100123a004 [24] F. Strelow and A. Henglein, “Time Resolved Chemisorp- tion of I- and SH- on Colloidal Silver Particles (A Stopped Flow Study),” Journal of Physical C 31, 1995, pp. 11834-11838 hemistry, Vol. 99, No. . doi:10.1021/j100031a008 [25] A. Hanglein, “Colloidal Silver Nanoparticles: Photo- chemical Preparation and Interaction with O2, CCl4, and Some Metal Ions,” Chemistry of Materials, Vol. 10, No. 1, 1998, pp. 444-450. doi:10.1021/cm970613j [26] E. Janata, A. Henglein and B. G. Ershov, “First Clusters of Ag+ Ion Reduction in Aqueous Solution,” Journal of Physical Chemistry, Vol. 98, No. 42, 1994, pp. 10888- 10890. doi:10.1021/j100093a033 [27] D. Yu and V. W. W. Yam, “Hydrothermal-Induced As- sembly of Colloidal Silver Spheres into Various Nano- particles on the Basis of HTAB-Modified Silver Mirror Reaction,” Journal of Physical Chemistry B, Vol. 109, No. 12, 2005, pp. 5497-5503. doi:10.1021/jp0448346 [28] A. T. Le, P. T. Huy, P. D. Tam, T. Q. Huy, P. D. Cam, A. A. Kudrinskiy and Y. A. Krutyakov, “Green Synthesis of Finely-Dispersed Highly Bactericidal Silver Nanoparti- cles via Modified Tollens Technique,” Current Applied Physics, Vol. 10, No. 3, 2010, pp. 910-916. doi:10.1016/j.cap.2009.10.021 [29] A. G. Ingalls, “Amateur Telescope Making (Book one),” Scientific American Ind., New York, 1981, p. 101. [30] S. Huang and A. W. H. Mau, “Selective G gned Carbon Nanotubes on a rowth of Ali- Silver-Patterned Substrate . by the Silver Mirror Reaction,” Journal of Physical Chemistry B, Vol. 107, No. 15, 2003, pp. 3455-3458 doi:10.1021/jp034282b [31] P. Mukherjee and K. J. Mysels, “Critical Micelle Con- centrations of Aqueous Surfactants, NSRDS-NBS # 36,” Superintendent of Documents, Washington, DC, 1971. [32] Z. Zhong, S. Patskovsky y, P. Bouvrette, J. H. T. Luong 3, and A. Gedanken, “The Surface Chemistry of Au Col- loids and Their Interactions with Functional Amino Ac- ids,” Journal of Physical Chemistry B, Vol. 108, No. 1 2004, pp. 4046-4052. doi:10.1021/jp037056a [33] Z. Khan, P. Kumar, Kabir-ud-Din, “Kinetics and Mecha- nism of the Reduction of Colloidal Manganese Dioxide by D-Fructose,” Colloids and Surfaces A: Physicochemi- cal and Engineering Aspects, Vol. 248, No. 1-3, 2004, pp. 25-31. doi:10.1016/j.colsurfa.2004.08.020 [34] R. Bacaloglu, C. A. Bunton and F. Ortega, “Micellar Enhancements of Rates of SN2 Reactions of Halide Ions: The Effect of Head Group Size,” Journal of Physical Chemistry, Vol. 93, No. 4, 1989, pp. 1497-1502. doi:10.1021/j100341a061 [35] C. Tondre and M. Hebrant, “Micellar and Microemulsion Systems to Perform Heterogeneous Reactions, Biphasic Extraction and Solute Transport,” Journal of M Liquids, Vol. 72, No. 1-3, 1 olecular 997, pp. 279-294. doi:10.1016/S0167-7322(97)00042-1 [36] A. Henglein, “Colloidal Silver Nanoparticles: Photo- chemical Preparation and Interaction with O2, CCl4, and Some Metal Ions,” Chemistry of Materials, V 1, 1998, pp. 444-450. ol. 10, No. 70613jdoi:10.1021/cm9 [37] Z. Y. Huang, G. Mills and B. “Hajek, Spontaneous For- mation of Silver Particles in Basic 2-Propanol,” Journal of Physical Chemistry, Vol. 97, No. 44, 1993, pp. 11542- 11550. doi:10.1021/j100146a031 [38] A. Rafey, K. B. L. Shrivastav, S. A. Iqbal and Z. Khan, “Growth of Ag-nanoparticles Using Aspartic Acid in Aqueous Solutions,” Journal of Colloid and Interface C opyright © 2011 SciRes. JBNB
 Silver Nanoparticles: Green Route, Stability and Effect of Additives Copyright © 2011 SciRes. JBNB 399 . 190- 195. Science, Vol. 534, No. 3, 2011, pp doi:10.1016/j.jcis.2010.10.046 [39] S. Tascioglu, “Micellar Solutions as Reaction Media,” Tetrahedron, Vol. 52, No. 34, 1996, pp. 11113-11152. doi:10.1016/0040-4020(96)00669-2 [40] Kabir-ud-Din, J. K. J. Salem, S. Kumar and Z. khan, e, “The Micelle-Induced Interaction between Ninhydrin and Tryptophan,” Journal of Colloid and Interface Scienc Vol. 215, No. 1, 1999, pp. 9-15. doi:10.1006/jcis.1999.6211 [41] H. A. Al-Lohedan, C. A. Bunton and L. S. Romsted, “Mi- cellar Effects upon the Reaction of Betaine Esters with Hydroxide Ion,” Journal of Phys No. 14, 1981, pp. 2123-2129 ical Chemistry, Vol. 85, . doi:10.1021/j150614a034 [42] C. A. Bunton and J. R. Moffatt, “Micellar Effects upon Substitutions by Nucleophilic Anions,” Journal of Phy- sical Chemistry, Vol. 92, No. 10, 1988, pp. 2896-2902. doi:10.1021/j100321a038 [43] D. Longa, G. Wua and S. Chen, “Preparation of Oli- gochitosan Stabilized Silver Nanoparticles by Gamma Ir- radiation,” Radiation Physics and Chemistry, Vol. 76, N 7, 2007, pp. 1126-1131. o. doi:10.1016/j.radphyschem.2006.11.001 [44] R. A. Alvarez-Puebla, E. Arceo, P. J .G. Goulet, J. J. Garrido and R. F. Aroca, “Role of Nanoparticle Surface Charge in Surface-Enhanc of Physical Chemistry B, Vol. 109, N ed Raman Scattering,” Journal o. 9, 2005, pp. 3787-3792. doi:10.1021/jp045015o [45] H. A. Al-Lohedan, C. A. Bunton and J. R. Moffatt, “Quantitative Treatment of Micellar Effects upon the Nu- cleophilicity of Halide Ions,” Journal of Physical Chem- istry, Vol. 87, No. 2, 1983, pp. 332-335. doi:10.1021/j100225a033 [46] H. A. Al-Lohedan, “Nucleophilicity of Halide Ions in the Micellar Pseudophase,” Tetrahedron, Vol. 45, No. 6, 1987, pp. 1747-1754. doi:10.1016/S0040-4020(01)80038-7 . Batchelder and D. A. Sm 57- [47] Y. Sato, J. J. Wang, D. Nith, “Simple Chemical Method for Forming Silver Surfaces with Controlled Grain Sizes for Surface Plasmon Ex- periments,” Langmuir, Vol. 19, No. 17, 2003, pp. 68 6861. doi:10.1021/la0301240 [48] L. Kvitek, A. Panacek, J. Soukupova, M. Kolar, R. Ve- cerova, R. Prucek, M. Holecova and R. Zboril, “Effect of Surfactants and Polymers on Stability and Antibacterial Activity of Silver Nanoparticles (NPs),” Journal of Physical Chemistry C, Vol. 112, No. 15, 2008, pp. 5825-5834. doi:10.1021/jp711616v [49] J. Soukupova, L. Kvitek, A. Panacek, T. Nevecna and R. Zboril, “Comprehensive study on Surfactant Role on Sil- ver Nanoparticles (NPs) Prepared via Modified Tollens Process,” Materials Chemistry and Physics, Vol. 111, No. 1, 2008, pp. 77-81. doi:10.1016/j.matchemphys.2008.03.018 [50] D. V. Goia and E. Matijevic, “Preparation of Monodis- persed Metal Partia 22, No. 11, 1998, pp. 1203-1215. ls,” New Journal of Chemistry, Vol. 039/a709236idoi:10.1 , [51] A. Henglein, “Small-Particle Research: Physicochemical Properties of Extremely Small Colloidal Metal and Semi- conductor Particles,” Chemical Reviews, Vol. 89, No. 8 1989, pp. 1861-1873. doi:10.1021/cr00098a010 [52] M. Nishizawa, Y. Sasaki and K. Saito, “Kinetics and Mechanisms of the Outer-Sphere Oxidation of Cis- Aquaoxovanadium(IV) Complexes Containing Quadrin- dentate Amino Polycarboxylates. Interpretation of the Difference in Activation Parameters with the Charge Type of Reactants,” Inorganic Chemistry, Vol. 24, No. 5, 1985, pp. 767-772. doi:10.1021/ic00199a028 [53] W. Wang, X. Chen and S. Efrima, “Silver Nanoparticles Capped by Long-Chain Unsaturated Carboxylates,” Jour- nal of Physical Chemistry B, Vol. 103, No. 34, 1999, pp. 7238-7246. doi:10.1021/jp991101q [54] M. G. Guzman, J. Dille and S. Godet, “Synthesis of Sil- ver Nanoparticles by Chemical Reduction Method and Their Antibacterial Activity,” World Academy of Science, Engineering and Technology, Vol. 43, 2008, pp. 357-364. [55] A. Serra, E. Filippo, M. Re, M. Palmisano, M. Vittori- Antisari, A. Buccolieri and D. Manno, “Non-function- alized Silver Nanoparticles for a Localized Surface Plas- mon Resonance-Based Glucose Sensor,” Nanotechnology, Vol. 20, No. 16, 2009, pp. 165501-165508. doi:10.1088/0957-4484/20/16/165501
|