 Journal of Biomaterials and Nanobiotechnology, 2011, 2, 400-408 doi:10.4236/jbnb.2011.24049 Published Online October 2011 (http://www.SciRP.org/journal/jbnb) Copyright © 2011 SciRes. JBNB Enzymatic Synthesis of Butyl Ferulate by Silica-Immobilized Lipase in a Non-Aqueous Medium Chandresh Chandel, Ashok Kumar, Shamsher S. Kanwar Department of Biotechnology, Himachal Pradesh University, Shimla, India. Email: kanwarss2000@yahoo.com Received March 18th, 2011; revised June 20th, 2011; accepted August 15th, 2011. ABSTRACT Butyl ferulate was synthesized using a silica-immobilized commercial lipase (Steapsin) in dimethylsulfoxide (DMSO). Lipase-immobilized by surface adsorption onto silica pretreated with 1% glutaraldehyde showed 89% binding of pro- tein. The esterification of butanol (100 mM) and ferulic acid (50 mM) by silica-bound biocatalyst was carried out at 45˚C for 6 h under shaking (120 rpm). The optimization of various reaction conditions like molar concentration of re- actants, biocatalyst concentration, reaction time, temperature, addition of molecular sieves, salt ions, and repetitive bio-catalysis in DMSO were studied, consecutively. The bound lipase (15 mg/ml) catalyzed the esterification of ferulic acid and butanol with a yield of 64 mM under optimized reaction conditions. Among the salt ions Cu2+, Zn2+ and Al3+ ions moderately promoted the ester yield (66 mM) while Mg2+, NH4+, Fe2+ and Ca2+ were found to decrease the ester yield. The by-product (H2O) produced in the reaction was scavenged by the molecular sieves (10 mg/ml) added to the reaction mixture, which enhanced the formation of ester up to 74 mM. During the repetitive reactions, the bound lipase produced 32 mM ester after 4th cycle of esterification. On scaling-up the reaction volume to 30 ml, 32.5 mM butyl feru- late was synthesized under optimized conditions. Keywords: Silica, Glutaraldehyde, Butyl Ferulate Synthesis, Molecular Sieves 1. Introduction The lipases (EC 3.1.1.3.) are becoming increasingly at- tractive in the pharmaceutical, cosmetic and oil industry due to their stability, especially selectivity and successful biotransformation. Currently, scientists are making ef- forts to evolve newer derivatives of natural plant pro- ducts that are more amenable as emoluments, constitu- ents of cosmetics, toiletries and fragrances etc. Such pro- ducts of plant origin are likely to be better acceptable for human use with least side effects or cytotoxicity. Esters of cinnamic acid, ellagic acid, ferulic acid etc. are orga- nic compounds of biotechnological relevance that could be suitable modified as flavor/fragrance compounds, pre- cursors of pharmaceuticals and as additives in foods, cos- metics and sun-screens. Ferulic acid (4-hydroxy-3-me- thoxy cinnamate) is an effective antioxidative agent among various hydroxycinnamic acids as it potentially prevents the autoxidation of linoleic acid in the ethanol- buffer system [1]. Moreover, esters of ferulic acid are easy to apply on skin and show an increased antioxidant activity instead of use of ferulic acid that has an acidic nature and as such cannot be spread on the skin. The activity of alkyl ferulate is somewhat influenced by the chain length of alcohol moiety [2]. Comparing the effects of ferulic acid and its esters, hexyl, octyl and 2-ethyl-1- hexyl ferulates were significantly more active than feru- lic acid itself, and higher or lower carbon chain homo- logues than hexyl and octyl ferulates were less active as anti-oxidant mo lecules [1,3]. Ethyl ferulate that is widely distributed in plants possesses a UV absorbance maxi- mum at ~320 nm [4]. Ferulic acid is believed to suppress melanin generation by antagonizing tyrosine because its chemical structure is similar to tyrosine; and it effec- tively absorbs harmful long wave ultraviolet radiations emerging from the Sun. Ferulic acid and its esters are well known “oxidation inhibitors”. Butyl ferulate exhibits free radical scavenging activity against DPPH radicals [1]. Also the same compound was reported to play a chemopreventive role in cancer by in- ducing tumor cells apoptosis. A significant cytochromec
 Enzymatic Synthesis of Butyl Ferulate by Silica-Immobilized Lipase in a Non-Aqueous Medium401 release has been found when treating TM-3 cells with butyl ferulate [5]. In the light of the afor esaid an attempt was made to synthesize butyl ferulate using a silica- bound commercial lipase “Steapsin”. Most common cin- namic acids and short-chain esters are water soluble, limiting their usefulness as waterproof sunscreens but ferulic acid is insoluble in water and its derivatives there- fore have been designed with long-chain hydrocarbons (e.g., octyl-p-methoxy cinnamate), which renders them water-insoluble and suitable for waterproof sunscreens [6]. The -OCH3 group of octyl-p-methoxy cinnamate acts as an electron-releasing group to improve the electron excitation process [7]. When triolin ferulate was com- bined with ferulyl-substituted oleins, it elevated the UV absorptivity of a cinnamate ester with the water- insolu- ble properties of a lipid [8]. The lipase-catalyzed trans- esterification of ethyl ferulate with ethanol could poten- tially lead to new sunscreen products, synthesized from natural products, while providing a value-added use for vegetable oils [9-11]. Ferulic acid that has little solubility in most alkanes and aqueous media could be solublized in DMSO or di- ethyl phthalate to achieve ester synthesis or trans-esteri- fication [12,13]. The immobilized enzymes are preferred for biocatalysts because of obvious easy recovery, in- creased stability at raised temperature and or pH as well as repetitive use in reaction system(s). The current work focuses on the synthesis of butyl ferulate from butyl al- cohol and ferulic acid by employing immobilized-lipase under optimized reaction conditions such as catalyst loa- ding, mole ratio, temperature, pH, effect of salt ions, mo- lecular sieves and repetitiv e use of silica-bound lipase. 2. Material and Methods Ferulic acid and butanol were procured from Merck Schuchardt, Germany. DMSO from Sigma Aldrich, USA; and molecular sieves 3 Å × 1.5 mm were from E. Merck (India) Ltd., Mumbai. Commercial lipase: Steapsin was obtained from Sisco Research Laboratory, Mumbai, In- dia; and silica 100 - 200 mesh was from s-d fin e Chemi- cals, Mumbai, India. All chemicals were of analytical grade and were used as received. 2.1. Determination of Lipase Activity The activity of free and immobilized Steapsin was mea- sured by lipase assay [14] with minor modifications. The reaction mixture contained 80 µl of p-nitrophenol palmi- tate (p-NPP) stock solution (20 mM p-NPP prepared in isopropyl alcohol), 80 µl of the test sample (lipase) and Tris buffer (0.05 M, pH 8.5) to make final volume to 3 ml . The reaction mixture was incubated at 45˚C for 10 min in a water-bath. The reaction was stopped by keeping the reaction mixture at –20˚C for 10 minutes. An appropriate control with a heat-inactivated enzyme (5 min in a boil- ing-water bath) was included with each assay. The ab- sorbance of p-nitrophenol released in the reaction mix- ture was measured at A410. Each of the assays was per- formed in triplicate, and mean values ± standard devia- tions were presented. One unit (1 IU) of lipase activity was defined as the micromoles of p-nitrophenol released/ minute by the hydrolysis of p-NPP by 1 ml of soluble enzyme or 1 g of silica-bound enzyme (weight of matrix included) at 45˚C under assay conditions. All the addi- tives including buffer were pre-incubated at 45˚C for short period (3 min) before the enzyme was added to start the reaction. 2.2. Determination of Protein Content Protein concentration in the free or matrix-bound lipase was determined as described previously [15] using bo- vine serum albumin as a standard. 2.3. Immobilization of Enzyme onto Silica The matrix was washed three times with Tris buffer 0.05 M pH 8.5 to remove soluble impurities. The silica (3.5 g), pre-equilibrated in an excess volume of Tris buffer (0.05M, pH 8.5), was incubated with commercial lipase (Steapsin 3.60 IU/ml and 18.2 mg/ml protein) at 8˚C overnight. The volume of the supernatant, amount of unbound protein, and the lipase activity were estimated. The bound-lipase activity was assayed in silica-bound lipase 20 mg/reaction volume of 2 ml). The bound pro- tein in the matrix was determined by subtraction of the unbound protein in the sup ernatant from the total protein used for immob ilization. The silica was pretreated with a glutaraldehyde (12 ml; 1%, v/v in 0.05 M Tris pH 8.5) before immobilization of the lipase by adsorption [16,17]. 2.4. Esterification of Butanol and Ferulic Acid Butyl ferulate synthesis was performed by using 100 mM ethanol, 50 mM ferulic acid and silica-bound lipase (~2.95 IU/mg) taken in 2.0 ml of DMSO in Tefloncap- ped glass-vial (5 ml capacity). The reaction was per- formed at 45˚C for 6 h along w ith buffer-treated silica as a control under shaking (120 rpm). Each of the esterifi- cation reactions was performed in triplicate, unless other- wise stated, and the mean values and standard deviation (SD) were determined. Samples of the reaction cocktail (10 l) were withdrawn at intervals and analyzed by gas liquid chromatography (GLC) for presence of butyl fe- rulate. 2.5. Analysis of Butyl Ferulate by GLC The butyl ferulate produced in the reaction cocktail was Copyright © 2011 SciRes. JBNB
 Enzymatic Synthesis of Butyl Ferulate by Silica-Immobilized Lipase in a Non-Aqueous Medium Copyright © 2011 SciRes. JBNB 402 O O O OH CH CH3 OH H 3O O O O CH CH H 3 3 Ferulic acidn-Butanol Butyl ferulate Immobilized lipase 45oC, DMSO Figure 1. Lipase catalyzed esterification of ferulic acid and n-butanol to produce butyl fer ulate. 2.6.4. Effect of Temperature on Ester Synthesis analyzed by GLC equipped with a flame ionization de- tector and a packed type column (10% SE-30 Chrom WHP, 2 meter length, mesh size 80 - 100, internal dia me- ter 1/8 inches, maximum temperature limit 300˚C; Netel Chromatograph, Thane, India). N2 was used as a carrier gas (30 cm3/min). The injector was set at 260˚C; detector at 270˚C and the column/ oven temperature was kept at 250˚C. The sample size for the GLC analysis was 2 l. The effect of reaction temperature (25, 35, 45, 55, and 65˚C) on the synthesis of butyl ferulate was studied in Teflon-capped glass-vials (5 ml). The reaction mixture containing butanol, ferulic acid (100 mM:50 mM) in DMSO and silica-immobilized lipase (30 mg/reaction volume of 2 ml) were incubated at each of the selected temperatures for 6 h under shaking. The amount of ester synthesized was determined by GLC. 2.6. Optimization of Parameters for Synthesis of Butyl Ferulate 2.6.5. Effect of Salt Ions on the Esterification of Ferulic Acid and Butanol. The effects of various parameters such as reaction time, relative molar concentration of reactants, reaction tempe- rature, pH, and effect of detergents, chelating agen ts, salt ions and repetitive use of immobilized lipase on yield of butyl ferulate were separately evaluated. All esterifica- tion reactions were performed using 20 mg of silica- bound lipase/reaction volume (2 ml) at 45˚C under sha- king condition (120 rpm) unless stated otherwise. The effect of salt ions on the synthesis of butyl ferulate was evaluated by pre-incubating the immobilized en- zyme separately with each of the salt ions NH4+, Mg2+, Ca2+, Mn2+, Zn2+ and Al+3 (1 mM in Tris buffer) for 30 min at 45˚C. The silica-bound lipase was spun down by centrifugation (10,000 rpm, 5 min) and the buffer con- taining salt ion(s) was completely decanted by inversion. The sedimented bound-enzyme was washed in DMSO and spun down again to perform the esterification as above. The synthesis of butyl ferulate was recorded, the- reafter. 2.6.1. Effect of Molar C oncentration of React an ts The concentration of feru lic acid and bu tanol w ere varied one at a time (25, 50, 75 and 100 mM). The reaction was carried out for 18 h at 45˚C under shaking. The subse- quent esterification reactions were carried out at opti- mized molar concentrations of ferulic acid and butanol. 2.6.6. Effect of Molecular Sieves on the Synthesis of Butyl Ferulate The reaction mixture containing 50 mM ferulic acid and 100 mM butanol when incubated at 45˚C with varying concentration of molecular sieves (10 - 200 mg) and to observe the effect of varying concentration of molecular sieves on the amount of ester synthesized was recorded in comparison to the control without molecular sieves. 2.6.2. Effect of Reaction Time on Synthesis of Butyl Ferulate The reaction mixture containing silica-bound lipase, and 100 mM of butanol and 50 mM ferulic acid in DMSO was incubated at 45˚C in a water-bath-incubator shaker for 18 h. At intervals of 2 h, the solvent phase was sam- pled and analyzed by GLC for the presence of butyl fe- rulate. The optimized reaction time was considered in the subsequent experiments. 2.6.7. Reusability of Silica Immobilized Lipase in Continuous Cycles of Esterification. The immobilized lipase was used for the synthesis of butyl ferulate in DMSO, repetitively up to 4th cycle of 6 h each at 45˚C under shaking. After first cycle of esteri- fication, the biocatalyst was recovered (by centrifuging and decanting the reaction mixture), and this biocatalyst was used to catalyze fresh esterification reaction. The reaction system contained 100 mM of butanol and 50 mM of ferulic acid treated with molecular sieves (10 mg/ml reaction volume) and the reaction was carried out under optimized conditions. 2.6.3. Effect of the Biocatalyst Concentration The synthesis of butyl ferulate was performed by placing varying amounts of the immobilized lipase (5 - 30 mg/ml in triplicates) in 2 ml of a reaction mixture containing 100 mM each of ferulic acid and butanol in DMSO at 45˚C for 6 h under shaking. GLC analysis was done for the quantification of the butyl ferulate produced in the reaction mixture.
 Enzymatic Synthesis of Butyl Ferulate by Silica-Immobilized Lipase in a Non-Aqueous Medium403 2.6.8. Effect of Volumetric Scale up of Reaction System on the Synthesis of Butyl Ferulate The volume of reaction mixture containing 50 mM:100 mM ferulic acid and butanol, respectively was scaled up from initial 2 ml to 5, 10, 15 and 30 ml with a corres- ponding increase in the amount of biocatalyst and the molecular sieves in the reaction system. 3. Results 3.1. Immobilization of the Commercial Lipase A commercial lipase “Steapsin” was optimally immo- bilized on to silica that retained 95.3% of total protein used for immobilization. The activity of free lipase was 3.6 IU/ml. After immobilization onto silica, the bound- lipase exhibited 2.95 IU activity and retained approxi- mately 98% of its orig in al hydro lytic activit y. To ach iev e the biocatalysis it is essential to determine the optimum values of temperature, pH, time of reaction, and molar concentration of reactants for higher yield of products [18-20]. 3.2. Optimization of Various Reaction Parameters for Esterification Reaction 3.2.1. Effec t of Mol ar Concentration of Re act ants The maximum ester synthesis (64 mM) was observed when concentration of ferulic acid and butanol was kept 50 mM: 100 mM (Figure 2). The reactant concentration above or below this ratio resulted in a decline in the syn- thesis of butyl ferulate. In contrast a little esterification could be achieved by immobilized lipase when concen- tration of ferulic acid was kept constant (100 mM) and butanol concentration was varied from 25 to 100 mM. Thus further esterification reactions were carried out at optimized molar concentrations of ferulic acid and bu- tanol (50 mM:100 mM, respectively). 3.2.2. Effect of Incubation Time on Synthesis of Butyl Ferulate Kinetics of immobilized-lipase catalyzed synthesis of butyl ferulate was studied for 18 h at 45˚C in DMSO under continuous shak ing. The butyl ferulate was formed with a maximum conversion rate of approximately 64 mM within a short period of 6 h (Figure 3). Thus in the subsequent experiments reaction time of 6 h at 45˚C for immobilized-lipase was considered optimum for the syn- thesis of butyl ferulate. 3.2.3. Effect of the Biocatalyst Concentration The synthesis of butyl ferulate was performed by placing varying amounts of the immobilized lipase (5-30 mg/ml in triplicates) in 2 ml of a reaction mixture containing 100 mM butanol and 50 mM ferulic acid in DMSO at 45˚C for 6 h under shaking (Figure 4). GLC analysis Figure 2. Effect of molar concentrations of reactants on the synthesis of butyl ferulate. The reaction mixture containing varying ratio of ethanol and ferulic acid in DMSO was in- cubated at 45˚C under shaking for 6 h. Figure 3. Effect of reaction time on the synthesis of butyl ferulate. The reaction mixture containing 100 mM of bu- tanol and 50 mM ferulic acid and, silica-bound biocatalyst in DMSO was incubated at 45˚C under shaking. Figure 4. Effect of biocatalyst concentration on synthesis of butyl ferulate. The reaction mixture containing 100 mM butanol and 50 mM ferulic acid and, silica-bound biocata- lyst in DMSO was incubated at 45˚C under shaking for 6 h. Copyright © 2011 SciRes. JBNB
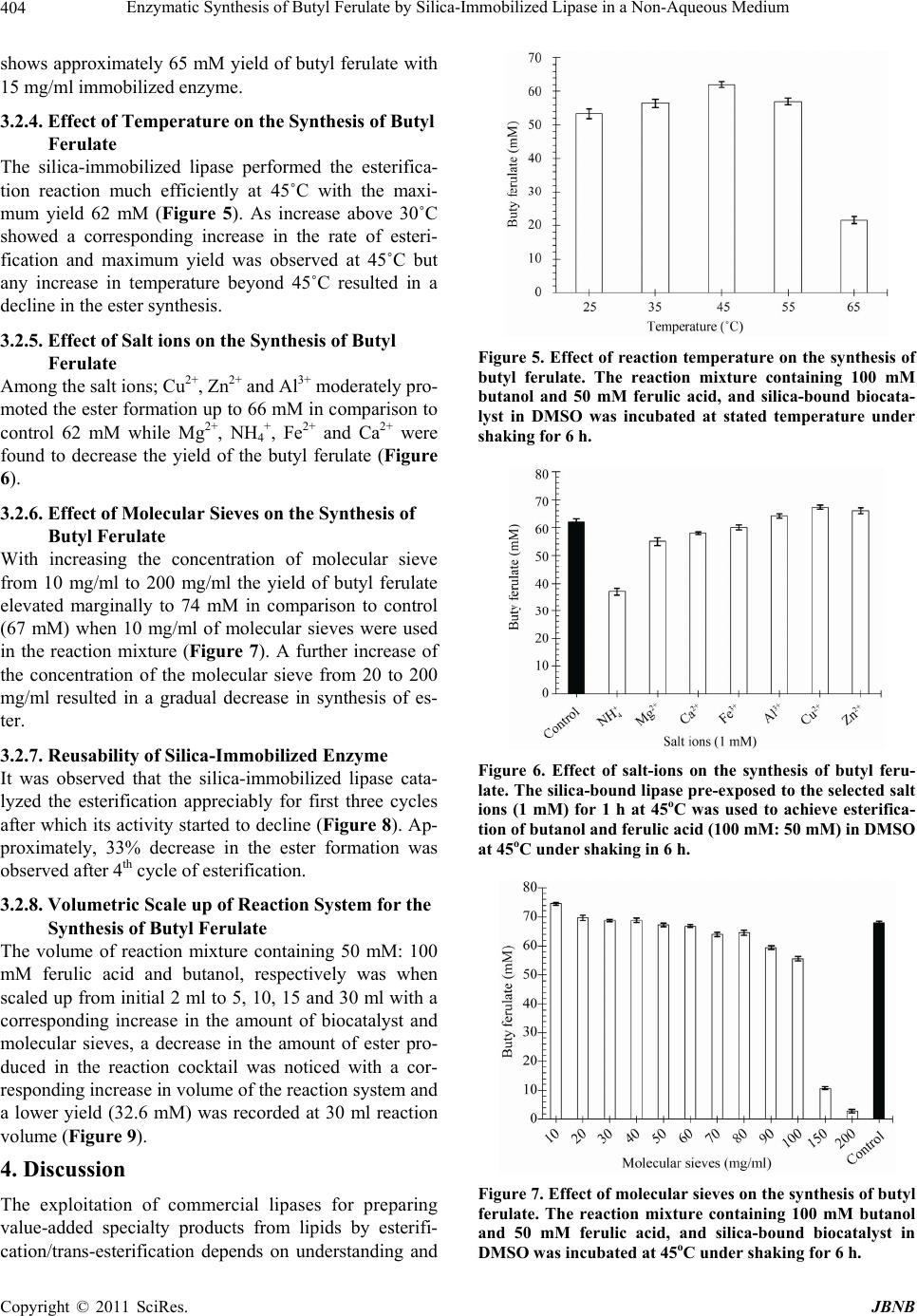 Enzymatic Synthesis of Butyl Ferulate by Silica-Immobilized Lipase in a Non-Aqueous Medium 404 shows approx imately 65 mM yield of butyl feru late with 15 mg/ml immobilized enzyme. 3.2.4. Effect of Temperature on the Synthesis of Butyl Ferulate The silica-immobilized lipase performed the esterifica- tion reaction much efficiently at 45˚C with the maxi- mum yield 62 mM (Figure 5). As increase above 30˚C showed a corresponding increase in the rate of esteri- fication and maximum yield was observed at 45˚C but any increase in temperature beyond 45˚C resulted in a decline in the ester synthesis. 3.2.5. Effect of Salt ions on the Synthesis of Butyl Ferulate Among the salt ions; Cu2+, Zn2+ and Al3+ moderately pro- moted the ester formation up to 66 mM in comparison to control 62 mM while Mg2+, NH4+, Fe2+ and Ca2+ were found to decrease the yield of the butyl ferulate (Figure 6). 3.2.6. Effect of Molecular Sieves on the Synthesis of Butyl Ferulate With increasing the concentration of molecular sieve from 10 mg/ml to 200 mg/ml the yield of butyl ferulate elevated marginally to 74 mM in comparison to control (67 mM) when 10 mg/ml of molecular sieves were used in the reaction mixture (Figure 7). A further increase of the concentration of the molecular sieve from 20 to 200 mg/ml resulted in a gradual decrease in synthesis of es- ter. 3.2.7. Reusability of Silica-Immobilized Enzyme It was observed that the silica-immobilized lipase cata- lyzed the esterification appreciably for first three cycles after which its activity started to decline (Figure 8). Ap- proximately, 33% decrease in the ester formation was observed after 4th cycle of esterification. 3.2.8. Volumetric Scale up of Reaction System for the Synthesis of Butyl Ferulate The volume of reaction mixture containing 50 mM: 100 mM ferulic acid and butanol, respectively was when scaled up from initial 2 ml to 5, 10, 15 and 30 ml with a corresponding increase in the amount of biocatalyst and molecular sieves, a decrease in the amount of ester pro- duced in the reaction cocktail was noticed with a cor- responding increase in volume of the reaction system and a lower yield (32.6 mM) was recorded at 30 ml reaction volume (Figure 9). 4. Discussion The exploitation of commercial lipases for preparing value-added specialty products from lipids by esterifi- cation/trans-esterification depends on understanding and Figure 5. Effect of reaction temperature on the synthesis of butyl ferulate. The reaction mixture containing 100 mM butanol and 50 mM ferulic acid, and silica-bound biocata- lyst in DMSO was incubated at stated temperature under shaking for 6 h. Figure 6. Effect of salt-ions on the synthesis of butyl feru- late. The silica-bound lipase pre-exposed to the selected salt ions (1 mM) for 1 h at 45oC was used to achieve esterifica- tion of butanol and ferulic acid (100 mM: 50 mM) in DMSO at 45oC under shaking in 6 h. Figure 7. Effect of molecular sieves on the synthesis of butyl ferulate. The reaction mixture containing 100 mM butanol and 50 mM ferulic acid, and silica-bound biocatalyst in DMSO was incubated at 45oC under shaking for 6 h. C opyright © 2011 SciRes. JBNB
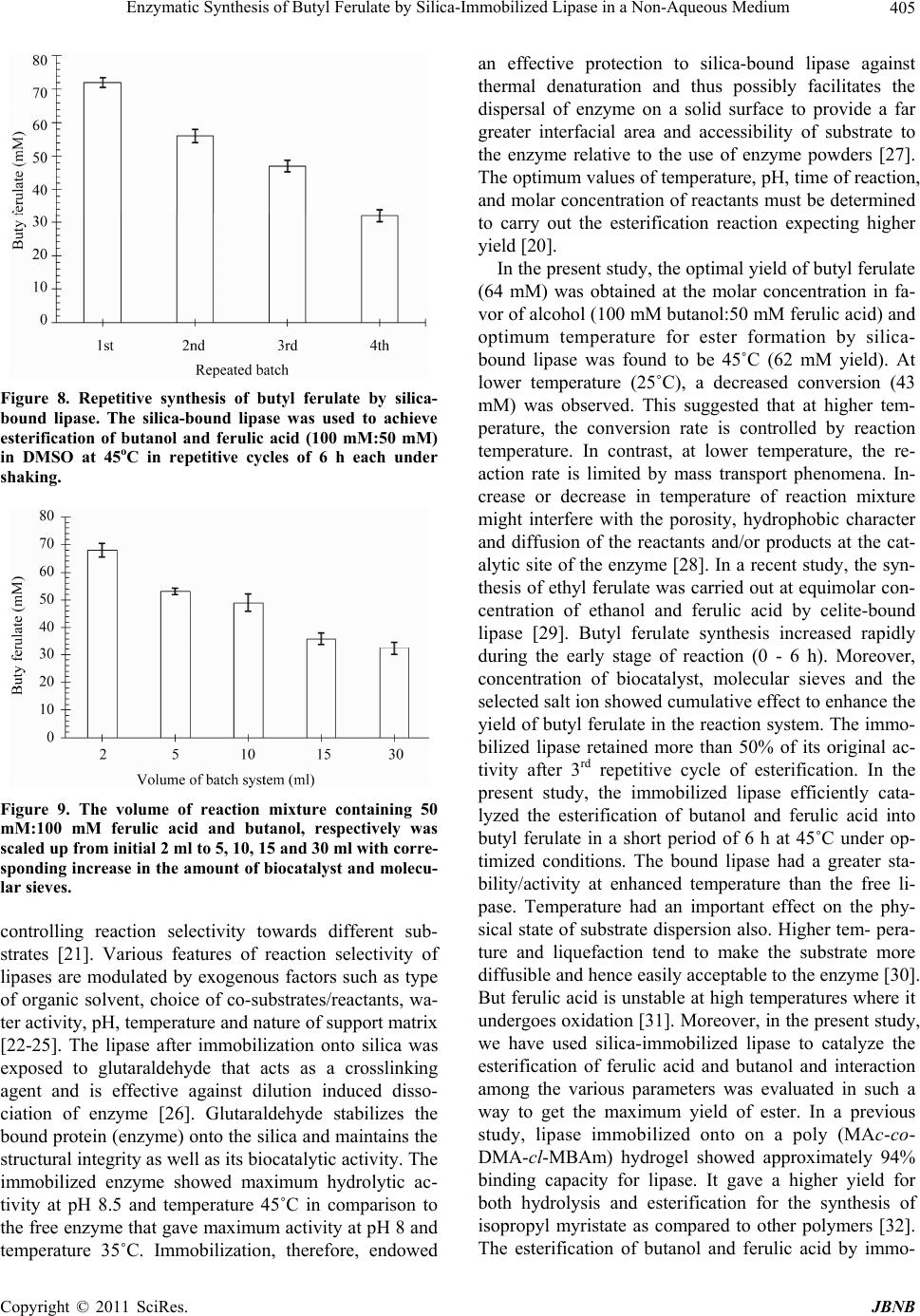 Enzymatic Synthesis of Butyl Ferulate by Silica-Immobilized Lipase in a Non-Aqueous Medium405 Figure 8. Repetitive synthesis of butyl ferulate by silica- bound lipase. The silica-bound lipase was used to achieve esterification of butanol and ferulic acid (100 mM:50 mM) in DMSO at 45 oC in repetitive cycles of 6 h each under shaking. Figure 9. The volume of reaction mixture containing 50 mM:100 mM ferulic acid and butanol, respectively was scaled up from initial 2 ml to 5, 10, 15 and 30 ml with corre- sponding increase in the amount of biocatalyst and molecu- lar sieves. controlling reaction selectivity towards different sub- strates [21]. Various features of reaction selectivity of lipases are modulated by exogenous factors such as type of organic solvent, choice of co-substrates/reactants, wa- ter activity, pH, temperature and nature of support matrix [22-25]. The lipase after immobilization onto silica was exposed to glutaraldehyde that acts as a crosslinking agent and is effective against dilution induced disso- ciation of enzyme [26]. Glutaraldehyde stabilizes the bound protein (enzyme) onto the silica and maintains the structural integrity as well as its biocatalytic activity. Th e immobilized enzyme showed maximum hydrolytic ac- tivity at pH 8.5 and temperature 45˚C in comparison to the free enzyme that gave maximum activity at pH 8 and temperature 35˚C. Immobilization, therefore, endowed an effective protection to silica-bound lipase against thermal denaturation and thus possibly facilitates the dispersal of enzyme on a solid surface to provide a far greater interfacial area and accessibility of substrate to the enzyme relative to the use of enzyme powders [27]. The optimum values of temperature, pH, time of reaction, and molar concentration of reactants must be determined to carry out the esterification reaction expecting higher yield [20]. In the present study, the op timal yield of butyl feru late (64 mM) was obtained at the molar concentration in fa- vor of alcohol (100 mM butanol:50 mM ferulic acid) and optimum temperature for ester formation by silica- bound lipase was found to be 45˚C (62 mM yield). At lower temperature (25˚C), a decreased conversion (43 mM) was observed. This suggested that at higher tem- perature, the conversion rate is controlled by reaction temperature. In contrast, at lower temperature, the re- action rate is limited by mass transport phenomena. In- crease or decrease in temperature of reaction mixture might interfere with the porosity, hydrophobic character and diffusion of the reactants and/or products at the cat- alytic site of the enzyme [28]. In a recent study, the syn- thesis of ethyl ferulate was carried out at equimolar con- centration of ethanol and ferulic acid by celite-bound lipase [29]. Butyl ferulate synthesis increased rapidly during the early stage of reaction (0 - 6 h). Moreover, concentration of biocatalyst, molecular sieves and the selected salt ion showed cu mu lative effect to enhance the yield of butyl ferulate in the reactio n system. The immo- bilized lipase retained more than 50% of its original ac- tivity after 3rd repetitive cycle of esterification. In the present study, the immobilized lipase efficiently cata- lyzed the esterification of butanol and ferulic acid into butyl ferulate in a short period of 6 h at 45˚C under op- timized conditions. The bound lipase had a greater sta- bility/activity at enhanced temperature than the free li- pase. Temperature had an important effect on the phy- sical state of substrate dispersion also. Higher tem- pera- ture and liquefaction tend to make the substrate more diffusible and hence easily acceptable to the enzyme [30]. But ferulic acid is unstab le at high temperatures where it undergoes oxidation [31]. Moreover, in the present study, we have used silica-immobilized lipase to catalyze the esterification of ferulic acid and butanol and interaction among the various parameters was evaluated in such a way to get the maximum yield of ester. In a previous study, lipase immobilized onto on a poly (MAc-co- DMA-cl-MBAm) hydrogel showed approximately 94% binding capacity for lipase. It gave a higher yield for both hydrolysis and esterification for the synthesis of isopropyl myristate as compared to other polymers [32]. The esterification of butanol and ferulic acid by immo- Copyright © 2011 SciRes. JBNB
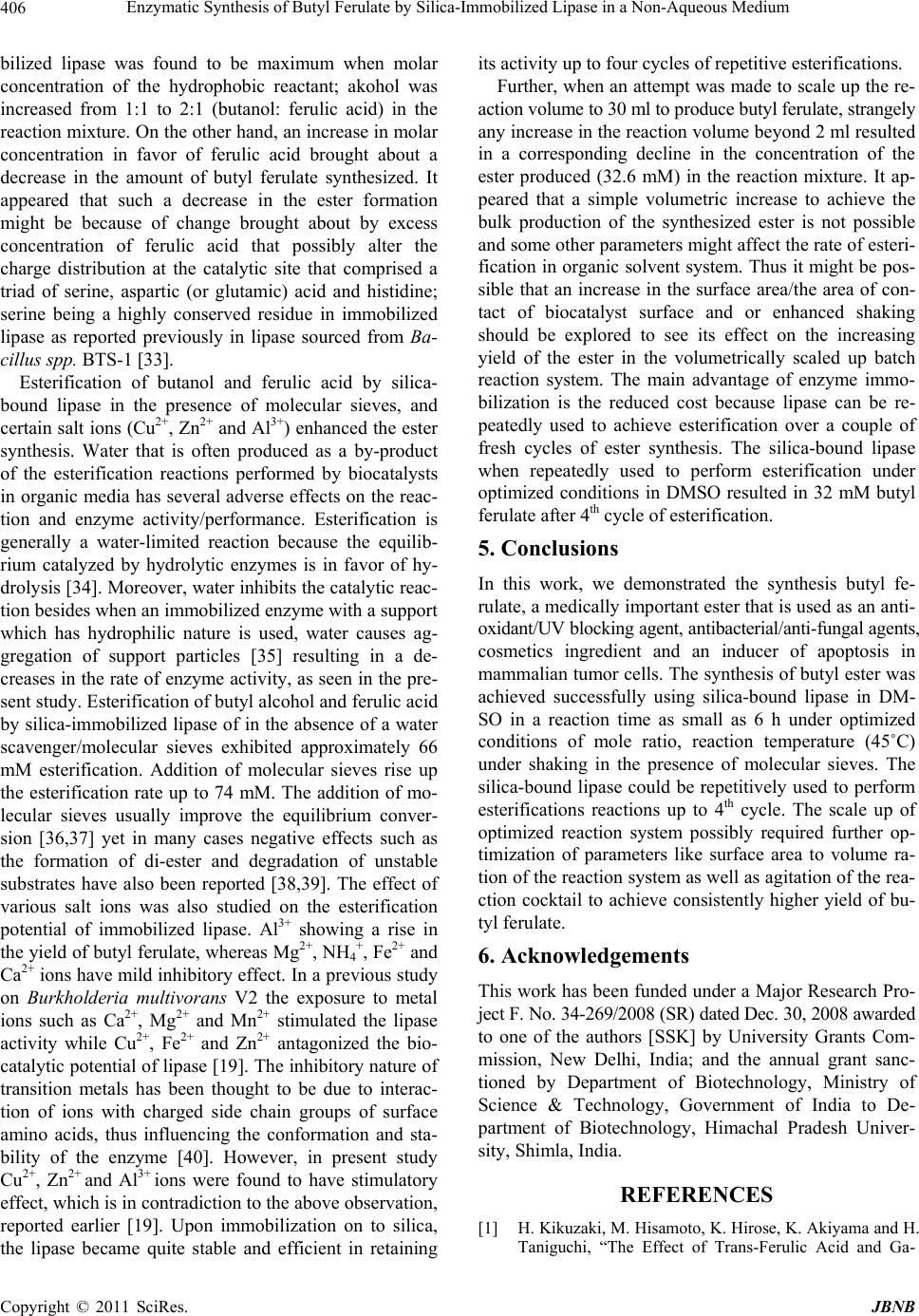 Enzymatic Synthesis of Butyl Ferulate by Silica-Immobilized Lipase in a Non-Aqueous Medium 406 bilized lipase was found to be maximum when molar concentration of the hydrophobic reactant; akohol was increased from 1:1 to 2:1 (butanol: ferulic acid) in the reaction mixture. On the other hand, an increase in molar concentration in favor of ferulic acid brought about a decrease in the amount of butyl ferulate synthesized. It appeared that such a decrease in the ester formation might be because of change brought about by excess concentration of ferulic acid that possibly alter the charge distribution at the catalytic site that comprised a triad of serine, aspartic (or glutamic) acid and histidine; serine being a highly conserved residue in immobilized lipase as reported previously in lipase sourced from Ba- cillus spp. BTS-1 [33]. Esterification of butanol and ferulic acid by silica- bound lipase in the presence of molecular sieves, and certain salt ions (Cu2+, Zn2+ and Al3+) enhanced the ester synthesis. Water that is often produced as a by-product of the esterification reactions performed by biocatalysts in organic media has several adverse effects on the reac- tion and enzyme activity/performance. Esterification is generally a water-limited reaction because the equilib- rium catalyzed by hydrolytic enzymes is in favor of hy- drolysis [34]. Moreover, water inhibits the catalytic reac- tion besides when an immobilized en zyme with a suppo rt which has hydrophilic nature is used, water causes ag- gregation of support particles [35] resulting in a de- creases in the rate of enzyme activity, as seen in the pre- sent study. Esterification of butyl alcohol and ferulic acid by silica-immobilized lipase of in the absence of a water scavenger/molecular sieves exhibited approximately 66 mM esterification. Addition of molecular sieves rise up the esterification rate up to 74 mM. The addition of mo- lecular sieves usually improve the equilibrium conver- sion [36,37] yet in many cases negative effects such as the formation of di-ester and degradation of unstable substrates have also been reported [38,39]. The effect of various salt ions was also studied on the esterification potential of immobilized lipase. Al3+ showing a rise in the yield of butyl ferulate, whereas Mg2+, NH 4+, Fe2+ and Ca2+ ions have mild inhibitory effect. In a previous study on Burkholderia multivorans V2 the exposure to metal ions such as Ca2+, Mg2+ and Mn2+ stimulated the lipase activity while Cu2+, Fe2+ and Zn2+ antagonized the bio- catalytic potential of lipase [19 ]. The inhibitory nature of transition metals has been thought to be due to interac- tion of ions with charged side chain groups of surface amino acids, thus influencing the conformation and sta- bility of the enzyme [40]. However, in present study Cu2+, Zn2+ and Al3+ ions were found to have stimulatory effect, which is in contradictio n to the abo ve observ ation , reported earlier [19]. Upon immobilization on to silica, the lipase became quite stable and efficient in retaining its activity up to four cycles of repetitive esterifications. Further, when an attempt was made to scale up the re- action volume to 30 ml to produce but yl ferulate, strangely any increase in the reaction volume beyond 2 ml resulted in a corresponding decline in the concentration of the ester produced (32.6 mM) in the reaction mixture. It ap- peared that a simple volumetric increase to achieve the bulk production of the synthesized ester is not possible and some other parameters might affect the rate of esteri- fication in organic solvent system. Thus it might be pos- sible that an increase in the surface area/the area of con- tact of biocatalyst surface and or enhanced shaking should be explored to see its effect on the increasing yield of the ester in the volumetrically scaled up batch reaction system. The main advantage of enzyme immo- bilization is the reduced cost because lipase can be re- peatedly used to achieve esterification over a couple of fresh cycles of ester synthesis. The silica-bound lipase when repeatedly used to perform esterification under optimized conditions in DMSO resulted in 32 mM butyl ferulate after 4th cycle of esterification. 5. Conclusions In this work, we demonstrated the synthesis butyl fe- rulate, a medically important ester that is used as an anti- oxidant/UV blocking agent, antibacterial/anti-fungal agents, cosmetics ingredient and an inducer of apoptosis in mammalian tumor cells. The synthesis of butyl ester was achieved successfully using silica-bound lipase in DM- SO in a reaction time as small as 6 h under optimized conditions of mole ratio, reaction temperature (45˚C) under shaking in the presence of molecular sieves. The silica-bound lipase could be repetitively used to perform esterifications reactions up to 4th cycle. The scale up of optimized reaction system possibly required further op- timization of parameters like surface area to volume ra- tion of the reaction system as well as agitation of the rea- ction cocktail to achieve consistently higher yield of bu- tyl ferulate. 6. Acknowledgements This work has been funded under a Major Research Pro- ject F. No. 34-269/2008 (SR) dated Dec. 30, 2008 awarded to one of the authors [SSK] by University Grants Com- mission, New Delhi, India; and the annual grant sanc- tioned by Department of Biotechnology, Ministry of Science & Technology, Government of India to De- partment of Biotechnology, Himachal Pradesh Univer- sity, Shimla, India. REFERENCES [1] H. Kikuzaki, M. Hisamoto, K. Hirose, K. Akiyama and H. Taniguchi, “The Effect of Trans-Ferulic Acid and Ga- C opyright © 2011 SciRes. JBNB
 Enzymatic Synthesis of Butyl Ferulate by Silica-Immobilized Lipase in a Non-Aqueous Medium407 mmaoryzanol on Ethanol-Induced Liver Injury in C57BL Mouse,” Journal of Agricultural and Food Chemistry, Vol. 50, No. 7, 2002, pp. 2161-2168. doi:10.1021/jf011348w [2] J. Chalas, C. Claise, M. Edeas, C. Messaoudi, L. Vergnes, A. Abella and A. Lindenbaum, “Effect of Ethyl Esterifi- cation of Phenolic Acids on Low-Density Lipoprotein O- xidation,” Biomedicine Pharmacotherapy Vol. 55, No. 1, 2001, pp. 54-60. doi:10.1016/S0753-3322(00)00011-1 [3] N. Nakatani, Y. Tachibana and H. Kikuzaki, “Establish- ment of Model Substrate Oil for Antioxidant Activity Assessment by Oil Stability Index Method,” Journal of the American Oil Chemists’ Society, Vol. 78, No. 1, 2001, pp. 19-23. [4] L. D. Compton, A. Joseph, A. J. Laszlo and A. M. Ber- how, “Lipase-Catalyzed Synthesis of Ferulate Esters,” Journal of the American Oil Chemists’ Society, Vol. 77, No. 5, 2000, pp. 513-519. [5] E. Cione, P. Tucci, V. Senatore, M. Perri, S. Trombino, F. Iemma, N. Picci and G. Genchi, “Synthesized Esters of Ferulic Acid Induce Release of Cytochrome C From Rat Testes Mitochondria,” Journal of Bioenergetics and Biomembranes, Vol. 40, No. 1, 2008, pp. 19-26. doi:10.1007/s10863-007-9097-7 [6] D.L. Compton, “Sunscreens Based on Vegetable Oil,” Lipid Technology, Vol. 17, No. 3, 2005, pp. 276-279. [7] A. Saija, A. Tomaino, R. L. Cascio, D. Trombetta, A. Proteggente, A. D. Pasquale, N. Uccella and F. Bonina, “Ferulic and Caffeic Acids as Potential Protective Agents against Photooxidative Skin Damage,” Journal of the Sci- ence of Food and Agriculture, Vol. 79, No. 3, 1999, pp. 476-480. doi:10.1002/(SICI)1097-0010(19990301)79:3<476::AID- JSFA270>3.0.CO;2-L [8] T. Akihisa, K. Tasukawa, M. Yamaura, M. Ukiya, Y. Ki- mura, N. Shimizu and K. Arai, “Triterpene Alcohol and Sterol Ferulates from Rice Bran and Their Antioxidant Properties,” Journal of Agricultural and Food Chemistry, Vol. 48, No. 6, 2000, pp. 2313-2319. doi:10.1021/jf000135o [9] J. A. Laszlo, D. L. Compton, F. J. Eller, S. L. Taylor and T. A. Isbell, “Packed-Bed Bioreactor Synthesis of Feru- loylated Monoacyl and Diacylglycerols: Clean Produc- tion of a “Green” Sunscreen,” Green Chemistry, Vol. 5, No. 4, 2003, pp. 382-386. doi:10.1039/b302384b [10] A. Murakami, Y. Nakamura, K. Koshimizu, D. Takahashi, K. Matsumoto, K. Hagihara, H. Taniguchi, E. Nomura, A. Hosoda, T. Tsuno, Y. Maruta, H. W. Kim, K. Kawabata and H. Ohigashi, “FA15, a Hydrophobic Derivative of Ferulic Acid, Suppresses Inflammatory Responses and Skin Tumor Promotion: Comparison with Ferulic Acid,” Cancer Letters, Vol. 180, No. 2, 2002, pp. 121-129. doi:10.1016/S0304-3835(01)00858-8 [11] K. Warner and J. A. Laszlo, “Addition of Ferulic Acid, Ethyl Ferulate, and Feruloylated Monoacyl and Diacyl- glycerols to Salad Oils and Frying Oils,” Journal of the American Oil Chemists’ Society, Vol. 82, No. 9, 2005, pp. 647-652. [12] A. Zaks and A. M. Klibanov. “Enzymatic Catalysis in Nonaqueous Solvents,” The Journal of Biological Chem- istry, Vol. 263, No. 5, 1988, pp. 3194-3201. [13] T. Nishio, T. Chicano and M. Kamimura, “Purification and Some Properties of Lipase Produced by Pseudomo- nas fragi,” Agricultural and Biological Chemistry, Vol. 52, No. 5, 1988, pp. 1203-1208. doi:10.1271/bbb1961.52.1203 [14] U. K. Winkler and M. Stuckmann, “Glucogen, Hyaluro- nate and Some Other Polysaccharides Greatly Enhance the Formation of Exolipase by Serratia marcescens,” Journal of Bacteriology, Vol. 138, No. 3, 1979, pp. 663- 670. [15] O. H. Lowry, N. J. Rosenbrough, A. L. Farr and R. J. Randall, “Protein Measurement with the Folin Phenol Reagent,” Journal of Biological Chemistry, Vol. 193, No. 1, 1951, pp. 265–275. [16] H. J. Chae, M. J. In and E. Y. Kim, “Optimization of Protease Immobilization by Covalent Binding Using Glu- taraldehyde,” Applied Biochemistry and Biotechnology, Vol. 69, No. 1, 1998, pp. 53-67. [17] J. M. Palomo, R. L. Segura, G. Fernandez-Lorente, R. Fernandez-Lafuente and J. M. Guisa´n, “Glutaraldehyde Modification of Lipases Adsorbed on Aminated Supports: A Simple Way to Improve Their Behaviour as Enanti- oselective Biocatalyst,” Enzyme and Microbial Technol- ogy, Vol. 40, No. 4-5, 2007, pp. 704-707. doi:10.1016/j.enzmictec.2006.05.026 [18] S. Sun, L. Shan, Q. Jin, Y. Liu and X. Wang, “Solvent Free Synthesis of Glyceryl Ferulate Using a Commercial Microbial Lipase,” Biotechnology Letters, Vol. 29, No. 1, 2007, pp. 45-949. doi:10.1007/s10529-007-9338-1 [19] V. Dandavate, J. Jinjala, H. Kehria and D. Madamwar, “Production Partial Purification and Characterization of Organic Solvent Tolerant Lipases from Burkholderia multivorans V2 and Its Applications for Ester Synthesis,” Bioresource Technology, Vol. 100, No. 13, 2009, pp. 3374-3381. doi:10.1016/j.biortech.2009.02.011 [20] M. L. Verma and S. S. Kanwar, “Lipases, Wiley Ency- clopedia of Industrial Biotechnology,” Bioprocess Bio- separation and Cell Technology, In: Michael C. Flick- inger, Ed., Vol. 5, 2010, pp. 3550-3565. [21] R. G. Jensen, D. R. Galluzzo and V. J. Bush, “Selectivity is an Important Characteristic of Lipases (Acylglycerol Hydrolases),” Biocatalysis, Vol. 3. No. 2, 1990, pp. 307- 317. doi:10.3109/10242429008992074 [22] U. T. Bornscheur, C. Bessler, R. Sriniwas and S. H. Krishna, “Optimizing Lipase and Related Enzymes for Efficient Applications,” Trends in Biotechnology, Vol. 20, No. 10, 2002, pp. 433-437. doi:10.1016/S0167-7799(02)02046-2 [23] R. R. Klein, G. King, R. A. Moreau, G. P. McNeill, P. Villeneuve and M. J., Hass, “Additive Effects of Acyl- binding Site Mutations on the Fatty Acid Selectivity of Rhizopus delemar Lipase,” Journal of the American Oil Chemists’ Society, Vol. 74, No. 11, 1997, pp. 1401- 1407. Copyright © 2011 SciRes. JBNB
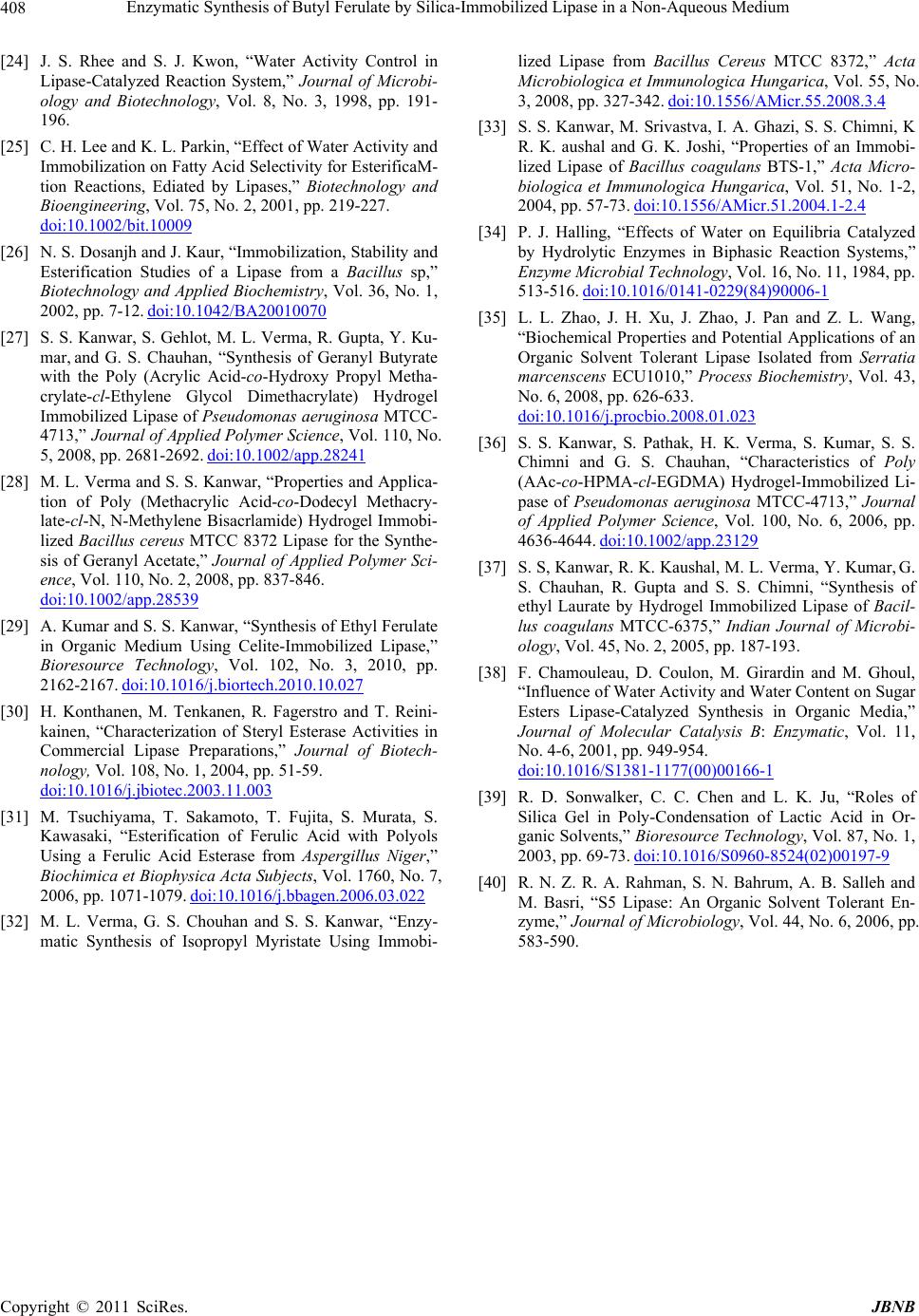 Enzymatic Synthesis of Butyl Ferulate by Silica-Immobilized Lipase in a Non-Aqueous Medium Copyright © 2011 SciRes. JBNB 408 [24] J. S. Rhee and S. J. Kwon, “Water Activity Control in Lipase-Catalyzed Reaction System,” Journal of Microbi- ology and Biotechnology, Vol. 8, No. 3, 1998, pp. 191- 196. [25] C. H. Lee and K. L. Parkin, “Effect of Water Activity and Immobilization on Fatty Acid Selectivity for EsterificaM- tion Reactions, Ediated by Lipases,” Biotechnology and Bioengineering, Vol. 75, No. 2, 2001, pp. 219-227. doi:10.1002/bit.10009 [26] N. S. Dosanjh and J. Kaur, “Immobilization, Stability and Esterification Studies of a Lipase from a Bacillus sp,” Biotechnology and Applied Biochemistry, Vol. 36, No. 1, 2002, pp. 7-12. doi:10.1042/BA20010070 [27] S. S. Kanwar, S. Gehlot, M. L. Verma, R. Gupta, Y. Ku- mar, and G. S. Chauhan, “Synthesis of Geranyl Butyrate with the Poly (Acrylic Acid-co-Hydroxy Propyl Metha- crylate-cl-Ethylene Glycol Dimethacrylate) Hydrogel Immobilized Lipase of Pseudomonas aeruginosa MTCC- 4713,” Journal of Applied Polymer Science, Vol. 110, No. 5, 2008, pp. 2681-2692. doi:10.1002/app.28241 [28] M. L. Verma and S. S. Kanwar, “Properties and Applica- tion of Poly (Methacrylic Acid-co-Dodecyl Methacry- late-cl-N, N-Methylene Bisacrlamide) Hydrogel Immobi- lized Bacillus cereus MTCC 8372 Lipase for the Synthe- sis of Geranyl Acetate,” Journal of Applied Polymer Sci- ence, Vol. 110, No. 2, 2008, pp. 837-846. doi:10.1002/app.28539 [29] A. Kumar and S. S. Kanwar, “Synthesis of Ethyl Ferulate in Organic Medium Using Celite-Immobilized Lipase,” Bioresource Technology, Vol. 102, No. 3, 2010, pp. 2162-2167. doi:10.1016/j.biortech.2010.10.027 [30] H. Konthanen, M. Tenkanen, R. Fagerstro and T. Reini- kainen, “Characterization of Steryl Esterase Activities in Commercial Lipase Preparations,” Journal of Biotech- nology, Vol. 108, No. 1, 2004, pp. 51-59. doi:10.1016/j.jbiotec.2003.11.003 [31] M. Tsuchiyama, T. Sakamoto, T. Fujita, S. Murata, S. Kawasaki, “Esterification of Ferulic Acid with Polyols Using a Ferulic Acid Esterase from Aspergillus Niger,” Biochimica et Biophysica Acta Subjects, Vol. 1760, No. 7, 2006, pp. 1071-1079. doi:10.1016/j.bbagen.2006.03.022 [32] M. L. Verma, G. S. Chouhan and S. S. Kanwar, “Enzy- matic Synthesis of Isopropyl Myristate Using Immobi- lized Lipase from Bacillus Cereus MTCC 8372,” Acta Microbiologica et Immunologica Hungarica, Vol. 55, No. 3, 2008, pp. 327-342. doi:10.1556/AMicr.55.2008.3.4 [33] S. S. Kanwar, M. Srivastva, I. A. Ghazi, S. S. Chimni, K R. K. aushal and G. K. Joshi, “Properties of an Immobi- lized Lipase of Bacillus coagulans BTS-1,” Acta Micro- biologica et Immunologica Hungarica, Vol. 51, No. 1-2, 2004, pp. 57-73. doi:10.1556/AMicr.51.2004.1-2.4 [34] P. J. Halling, “Effects of Water on Equilibria Catalyzed by Hydrolytic Enzymes in Biphasic Reaction Systems,” Enzyme Microbial Technology, Vol. 16, No. 11, 1984, pp. 513-516. doi:10.1016/0141-0229(84)90006-1 [35] L. L. Zhao, J. H. Xu, J. Zhao, J. Pan and Z. L. Wang, “Biochemical Properties and Potential Applications of an Organic Solvent Tolerant Lipase Isolated from Serratia marcenscens ECU1010,” Process Biochemistry, Vol. 43, No. 6, 2008, pp. 626-633. doi:10.1016/j.procbio.2008.01.023 [36] S. S. Kanwar, S. Pathak, H. K. Verma, S. Kumar, S. S. Chimni and G. S. Chauhan, “Characteristics of Poly (AAc-co-HPMA-cl-EGDMA) Hydrogel-Immobilized Li- pase of Pseudomonas aeruginosa MTCC-4713,” Journal of Applied Polymer Science, Vol. 100, No. 6, 2006, pp. 4636-4644. doi:10.1002/app.23129 [37] S. S, Kanwar, R. K. Kaushal, M. L. Verma, Y. Kumar, G. S. Chauhan, R. Gupta and S. S. Chimni, “Synthesis of ethyl Laurate by Hydrogel Immobilized Lipase of Bacil- lus coagulans MTCC-6375,” Indian Journal of Microbi- ology, Vol. 45, No. 2, 2005, pp. 187-193. [38] F. Chamouleau, D. Coulon, M. Girardin and M. Ghoul, “Influence of Water Activity and Water Content on Sugar Esters Lipase-Catalyzed Synthesis in Organic Media,” Journal of Molecular Catalysis B: Enzymatic, Vol. 11, No. 4-6, 2001, pp. 949-954. doi:10.1016/S1381-1177(00)00166-1 [39] R. D. Sonwalker, C. C. Chen and L. K. Ju, “Roles of Silica Gel in Poly-Condensation of Lactic Acid in Or- ganic Solvents,” Bioresource Technology, Vol. 87, No. 1, 2003, pp. 69-73. doi:10.1016/S0960-8524(02)00197-9 [40] R. N. Z. R. A. Rahman, S. N. Bahrum, A. B. Salleh and M. Basri, “S5 Lipase: An Organic Solvent Tolerant En- zyme,” Journal of Microbiology, Vol. 44, No. 6, 2006, pp. 583-590.
|