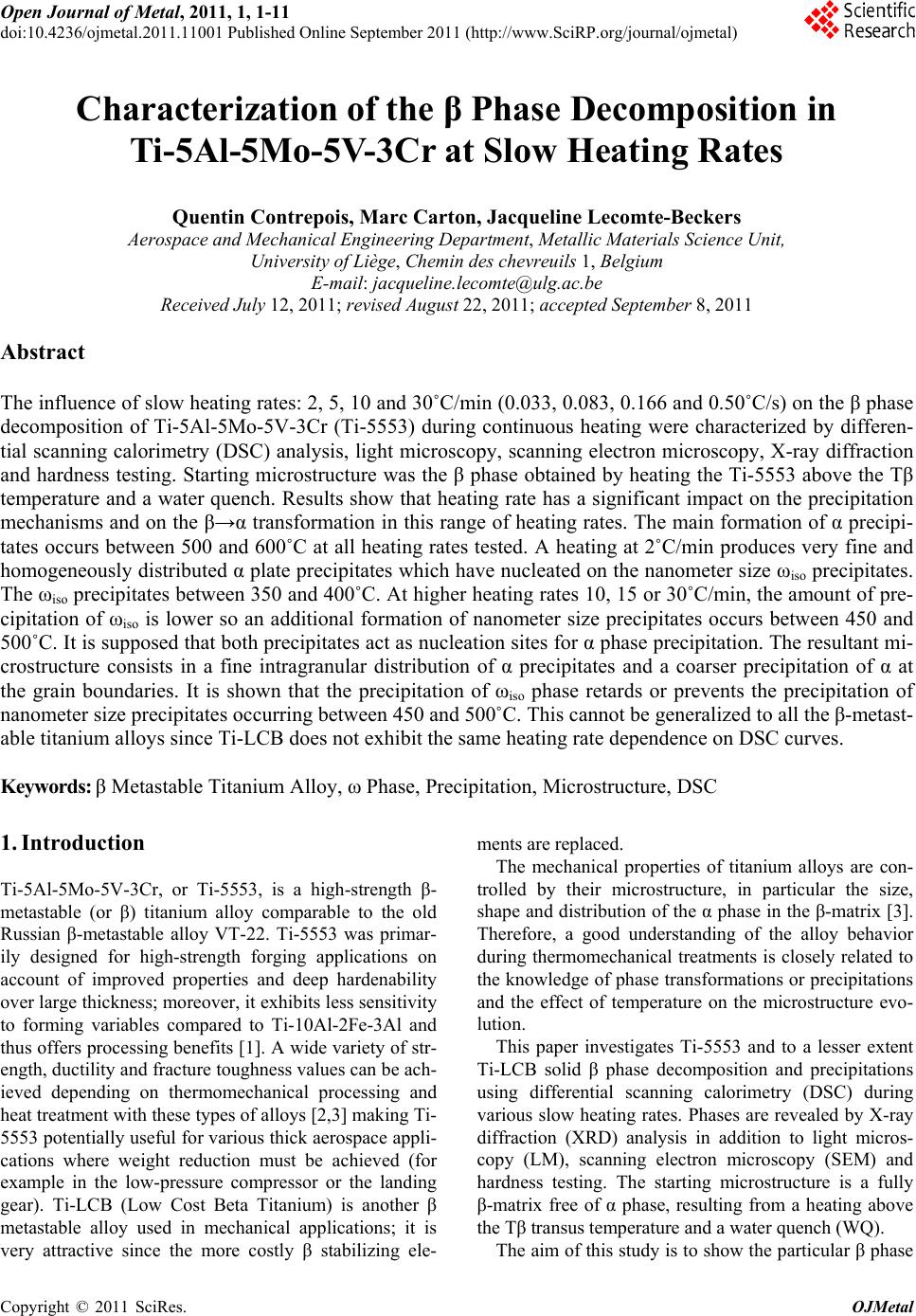 Open Journal of Metal, 2011, 1, 1-11 doi:10.4236/ojmetal.2011.11001 Published Online September 2011 (http://www.SciRP.org/journal/ojmetal) Copyright © 2011 SciRes. OJMetal Characterization of the β Phase Decomposition in Ti-5Al-5Mo-5V-3Cr at Slow Heating Rates Quentin Contrepois, Marc Carton, Jacqueline Lecomte-Beckers Aerospace and Mechanical Engineering Department, Metallic Materials Science Unit, University of Liège, Chemin des chevreuils 1, Belgium E-mail: jacqueline.lecomte@ulg.ac.be Received July 12, 2011; revised August 22, 2011; accepted September 8, 2011 Abstract The influence of slow heating rates: 2, 5, 10 and 30˚C/min (0.033, 0.083, 0.166 and 0.50˚C/s) on the β phase decomposition of Ti-5Al-5Mo-5V-3Cr (Ti-5553) during continuous heating were characterized by differen- tial scanning calorimetry (DSC) analysis, light microscopy, scanning electron microscopy, X-ray diffraction and hardness testing. Starting microstructure was the β phase obtained by heating the Ti-5553 above the Tβ temperature and a water quench. Results show that heating rate has a significant impact on the precipitation mechanisms and on the β→α transformation in this range of heating rates. The main formation of α precipi- tates occurs between 500 and 600˚C at all heating rates tested. A heating at 2˚C/min produces very fine and homogeneously distributed α plate precipitates which have nucleated on the nanometer size ωiso precipitates. The ωiso precipitates between 350 and 400˚C. At higher heating rates 10, 15 or 30˚C/min, the amount of pre- cipitation of ωiso is lower so an additional formation of nanometer size precipitates occurs between 450 and 500˚C. It is supposed that both precipitates act as nucleation sites for α phase precipitation. The resultant mi- crostructure consists in a fine intragranular distribution of α precipitates and a coarser precipitation of α at the grain boundaries. It is shown that the precipitation of ωiso phase retards or prevents the precipitation of nanometer size precipitates occurring between 450 and 500˚C. This cannot be generalized to all the β-metast- able titanium alloys since Ti-LCB does not exhibit the same heating rate dependence on DSC curves. Keywords: β Metastable Titanium Alloy, ω Phase, Precipitation, Microstructure, DSC 1. Introduction Ti-5Al-5Mo-5V-3Cr, or Ti-5553, is a high-strength β- metastable (or β) titanium alloy comparable to the old Russian β-metastable alloy VT-22. Ti-5553 was primar- ily designed for high-strength forging applications on account of improved properties and deep hardenability over large thickness; moreover, it exhibits less sensitivity to forming variables compared to Ti-10Al-2Fe-3Al and thus offers processing benefits [1]. A wide variety of str- ength, ductility and fracture toughness values can be ach- ieved depending on thermomechanical processing and heat treatment with these types of alloys [2,3] making Ti- 5553 potentially useful for various thick aerospace appli- cations where weight reduction must be achieved (for example in the low-pressure compressor or the landing gear). Ti-LCB (Low Cost Beta Titanium) is another β metastable alloy used in mechanical applications; it is very attractive since the more costly β stabilizing ele- ments are replaced. The mechanical properties of titanium alloys are con- trolled by their microstructure, in particular the size, shape and distribution of the α phase in the β-matrix [3]. Therefore, a good understanding of the alloy behavior during thermomechanical treatments is closely related to the knowledge of phase transformations or precipitations and the effect of temperature on the microstructure evo- lution. This paper investigates Ti-5553 and to a lesser extent Ti-LCB solid β phase decomposition and precipitations using differential scanning calorimetry (DSC) during various slow heating rates. Phases are revealed by X-ray diffraction (XRD) analysis in addition to light micros- copy (LM), scanning electron microscopy (SEM) and hardness testing. The starting microstructure is a fully β-matrix free of α phase, resulting from a heating above the Tβ transus temperature and a water quench (WQ). The aim of this study is to show the particular β phase
 2 Q. CONTREPOIS ET AL. decomposition of the Ti-5553 alloy during slow heating rates in the range of 2–30˚C/min and to put in relief the importance of heating rate when studying these types of alloys. Ivasishin et al. [4,5] have shown the importance of the heating rate on various Ti alloys including the predecessor of Ti-5553, VT-22, and showed that differ- ent precipitation mechanisms occurred by varying the heating rate until the ageing temperature. At slow heating rates (15˚C/min or 0.25˚C/s), they observed a fine, pla- te-like α precipitation and at very high heating rates (1200˚C/min or 20˚C/s) a coarse plate-like α microstruc- ture. They attributed this difference to the precipitation of the ω isothermal phase (ωiso) which acts as nucleation sites for the α precipitates in addition to the classical mi- crostructural items such as grain boundaries, dislocations or precipitates. The main difference with our study is the difference in heating rates analysed: 15 and 1200˚C/min in the Ivasishin studies and 2, 5, 15 and 30˚C/min for ours, plus the studied alloy Ti-5553 which is slightly di- fferent from VT-22. In addition, in a recent study, Wain et al. [6] characterized a refinement of the α precipitation in Ti-5553 by using either a low-temperature pre-ageing at 250˚C or a sufficiently slow heating rate up to the final ageing temperature when starting from a β phase micro- structure and according to these authors, the mechanism responsible for such a refinement is also an enhanced α nucleation on fine ωiso precipitates. Two forms of ω phase are differentiated: athermal ω (ωath) which appears without diffusion during cooling from the high tempera- ture β phase field and isothermal ω (ωiso) which precipi- tates during low temperature ageing. Influence of heating rate on β phase decomposition was also studied in the β-metastable titanium alloys Ti-B19 (Ti-3Al-5Mo-5V-4Cr-2Zr) [7] by electrical resis- tivity measurements and Ti17 (Ti-5Al-4Mo-4Cr-2Zr-2Sn) [8] by in situ high energy XRD. It was shown in Ti-B19 [7] that ωiso precipitates between 280 and 350˚C during a 6˚C/min heating but not during 60 and 600˚C/min heat- ing. Moreover the precipitation of the orthorhombic α” phase was evidence between 350 and 450˚C at 6˚C/min. Similarly, two different β phase decompositions are evi- dence in Ti17 [8]: at low heating rate 30˚C/min: β (250˚C) → ω + β (393˚C) → α” + β (490˚C) → α” + α + β (590˚C) → β + α (633˚C) and at high heating rate 300˚C/min: β (300˚C) → α” + β (528˚C) → α” + α + β (604˚C) → α + β (632˚C) (Temperatures in brackets correspond to the temperatures of analyses). It is then shown that the precipitation of ωiso is sup- pressed at 300˚C/min heating rate. Presence of ω precipitates in Ti-5553 has already been reported in detail in previous papers [9,10,11,12,13]. Jo- nes et al. [13] for example clearly showed, using in situ high energy XRD in a synchrotron that the ωath phase appears during rapid cooling from above the β transus temperature in Ti-5553 as much as 20% in volume. The role played by the ω phase in the α precipitation in this type of alloy is of interest and the possible mechanisms have been discussed previously [9,10,11,12]. Ohmori et al. [9] have discussed the role of ω precipi- tates on the nucleation of the HCP α and orthorhombic α” phases in a β-metastable titanium alloy (Ti-9.87V- 1.78Fe-3.20Al). Thanks to transmission electron micros- copy (TEM) analysis they showed that α laths nucleate at the ω / β interface and grow into both the β matrix and the ω particles at temperatures above 350˚C. Nag et al. [12] investigated the role of ω precipitates on the intra- granular nucleation of α precipitates in Ti-5553, it is thought to arise from two possible causes: 1- nucleation near the ω / β interface by local rejection of Al which is an ω destabilizer and a strong α stabilizer [14]; 2-dis- placive transformation within the core of the ω precipi- tates, leading to the formation of α platelets [10]. The importance of ω / β misfit in the α nucleation mecha- nisms is reminded, when there is a large misfit between the ω and β phases, α precipitates nucleate on ledges and misfit dislocations at ω / β interfaces. But Ti-5553 is thought to exhibit a low ω / β misfit so the role of ω on the nucleation of α precipitates is not clearly understood. Their detailed study leads to the conclusion that the β to α transformation and a compositional partitioning of al- loying elements occur in sequential steps, resulting in a mixed-mode displacive-diffusional transformation. In- deed, their experimental results indicate that a thermally activated diffusive process leads to the initial partitioning of alloying elements during coarsening of the ω precipi- tates, leading to the creation of favorable sites, margin- ally enriched in Al, for the nucleation of the α precipi- tates. Subsequently, these precipitates nucleate by a pri- marily displacive process, with a composition marginally enriched in Al as compared with the parent β matrix. These precipitates then grow via a coupled displacive- diffusional process with the diffusional partitioning of the alloying elements being rate-controlling. It is very in- teresting to note that: - after an annealing at 350˚C (2h) they detect a coarsening of ω precipitates and nucleation of α precipitates, - after an annealing at 400˚C (2h) there is less ω phase than at 350˚C but more α precipitates, and finally after an annealing at 600˚C they observe a growth and coarsening of α precipitates. They unfortunately do not mention any heating rate, which leads us to think that the samples were introduced into the furnace preheated to the desired temperature. However, we will see in our study that the thermal route have a high impact on the precipitation mechanisms, and especially the heating rate. Copyright © 2011 SciRes. OJMetal
 3 Q. CONTREPOIS ET AL. This overview shows that the presence of ω precipi- tates is of great importance for α nucleation and that the heating rate can have a strong impact on the ω phase for- mation so that we propose to analyze the role played by heating rate during the β phase decomposition of a β-metastable alloy. 2. Materials and Experimental Procedures Ti-5553 was provided by Timet Ugine (France), it was produced in the form of a forged billet of 200mm in di- ameter, the chemical composition of Ti-5553 is presen- ted in Table 1. The conventional microstructure delive- red by the producer is an α+β bimodal microstructure (see Figure 1-(a)), consisting of large globular primary α precipitates and smaller secondary α platelets in a β- ma- trix. This microstructure is achieved by a two-step ageing heat treatment at 820˚C for 2 h (i.e. below the Tβ transus) and at 630˚C for 8 h. This state is called “bimodal”. Ti- 5553 has been solution heat treated at a super-transus temperature of 865˚C (i.e. above the Tβ transus) over 30 min and water quenched in order to get a fully β-meta- stable matrix free of α phase (but the presence of ωath is expected). This state is termed the “β-solutionized” state (see Figure 1-(b)). Similar solution heat treatment was conducted with one other β-metastable titanium alloy: the Ti-LCB at 810˚C for 30min and water quenched, in order to compare the chemical composition effect. The Ti-5553 β-solutionized samples were heated at two dif- ferent rates: 2˚C and 30˚C/min using a resistance heating furnace at four different temperatures: 300, 400, 500 and 600˚C and then water quenched. Differential scanning calorimetry (DSC) is a thermo- analytical technique in which the difference in the amo- unt of heat required to increase the temperature of a sam- ple and a reference is measured as a function of tem- perature. When the sample undergoes an endothermic or an exothermic physical transformation, heat flux is mea- sured and compared to the reference which must have a well-defined heat capacity over the range of temperatures scanned. The difference of heat flux enables the detec- tion of transformations such as solid phase transforma- tions. Usually, the temperature program for a DSC ana- lysis is designed in such a way that the sample tempera- ture increases linearly as a function of time. DSC analy- ses were performed on a Netzsch DSC 404C device at four different heating rates: 2, 5, 10 and 15˚C/min, on Ti-5553 and Ti-LCB samples in the initial β-solutionized states (respectively 865˚C and 810˚C for 30min then wa- ter-quenched). The heating rates 15˚C/min should have been chosen instead of 30˚C/min for the heat treatment of samples in the resistance heating furnace, but this fur- nace wasn’t able to correctly heat at a constant rate of Table 1. Chemical composition of Ti-5553 and Ti-LCB alloys. (wt%) Al Mo V Cr Fe Ti-55535 5 5 3 0.3 Ti-LCB1.5 6.8 4.5 (a) (b) Figure 1. Microstructures of Ti-5553: (a). SEM micrograph in as-received bimodal state (820˚C - 2 h/630˚C - 8 h) con- sisting of globular primary alpha in fine lamellar α+β ma- trix; (b). LM micrograph of β-solutionized Ti-5553 (865˚C - 30 min/WQ). 15˚C/min. We assume that 15˚C/min and 30˚C/min lead to comparable microstructures in this paper. XRD analyses were performed on a Siemens D-5000 X-ray diffractometer using the Cu-Kα ray of wavelength 1.5406 Å. Samples were mechanically polished using the following steps: SiC papers (320 to 1200), diamond paste (9 and 3 µm), colloidal silica suspension (OPS with H2O2). The microstructures were revealed by chemical etching with 5% HNO3-5% HF in a water solution (kno- wn as Kroll etchant). Electronic SEM micrographs were carried out with an XL30 ESEM-FEG. Hardness was measured as Vickers hardness with a 10 kg load on an EMCO-TEST device. 3. Results 3.1. DSC Curves of Ti-5553 in β-Solutionized State (865˚C - 30 min/WQ) Samples of β-solutionized Ti-5553 were analyzed by DSC. The thermal cycles consisted of heating at constant rates from room temperature to 930˚C to achieve a final temperature above the Tβ transus. In order to character- ize a possible kinetic effect on the phase transformations, four different heating rates were tested: 2, 5, 10 and 15˚C/min. The DSC curves obtained during heating are shown in Figure 2. These curves are complex and the exothermic or endothermic nature of the transformation peaks is not easy to identify. The particular difficulty in DSC analysis is to correctly define a baseline which en- ables detection of the heat flux characteristic of phase we can determine three different “steps” or “areas”, called A, Copyright © 2011 SciRes. OJMetal
 Q. CONTREPOIS ET AL. Copyright © 2011 SciRes. OJMetal 4 Figure 2. DSC curves of β-solutionized Ti-5553 (865˚C - 30 min/WQ) at: (a). the four heating rates; (b). 15˚C/min, (c). 10˚C/min; (d). 5˚C/min; (e). 2˚C /min. B and C in Figure 2, for all the heating rates. transfor- mations. If we consider a baseline approximately hori- zontal at a DSC value close to the start of the curves, The first area A is not clear; it can either be an exother- mic reaction zone located at about 200˚C or an endo- thermic reaction ranging from about 220˚C to 350˚C, we think this is an endothermic peak. The second area B is a series of exothermic peaks ranging from about 350˚C to 575˚C (labeled 1 to 3 on the curves) and the third area C is an endothermic peak ranging from about 600˚C to 860˚C. As the Tβ transus is known to be near 865˚C, we assume that the endothermic reaction (area C) between about 650˚C to 860˚C is the α/β transformation (or the α/β phase field). The effect of heating rate is demonstrated by a shift in the exothermic peak positions towards higher tempera- tures for increasing heating rates, as shown in Figure 2-(a). The higher the heating rate is, the higher the tem- peratures of the different peaks are. This is an effect ob- served in thermally activated reactions. Another more interesting effect of the heating rate is the amplitude variation of the different exothermic peaks between 350 and 600˚C (area B). At heating rates of 10 and 15˚C/min, we clearly observe three different exothermic peaks
 5 Q. CONTREPOIS ET AL. whereas at the heating rate of 2˚C/min, the peak 2 does not exist. This peak 2 is slightly visible at 5˚C/min but it is clearly less important in comparison to the two higher heating rates. The peak 1 is the main exothermic peak at the two lower heating rates but it seems to decrease at the expense of the peak 2 as the heating rate increases. This variation indicates a change in the exothermic mecha- nisms occurring in this temperature range which is linked to the heating rate. However, the relative amplitude of the peak 3 is not affected by the variations in heating rate. The large endothermic area labeled C on all the DSC curves corresponds to the α/β phase field. The Tβ transus temperature is confirmed to be at about 860˚C. Addi- tional DSC results on Ti-5553 and Ti-LCB have previ- ously been reported by Carton et al. [16] with different starting microstructures, and they obtained different DSC curves. 3.2. DSC Curves of Ti-LCB in the β-Solutionized State (810˚C /30 min/WQ) DSC analyses carried out on β-solutionized Ti-LCB are shown on Figure 3. Two exothermic peaks are clearly illustrated at the four heating rates, the first between Figure 3. DSC curves of β-solutionized Ti-LCB (810˚C – 30 min/WQ) at: (a) the four heating rates; (b) 15˚C /min; (c) 10˚C /min; (d) 5˚C /min; (e) 2˚C /min. Copyright © 2011 SciRes. OJMetal
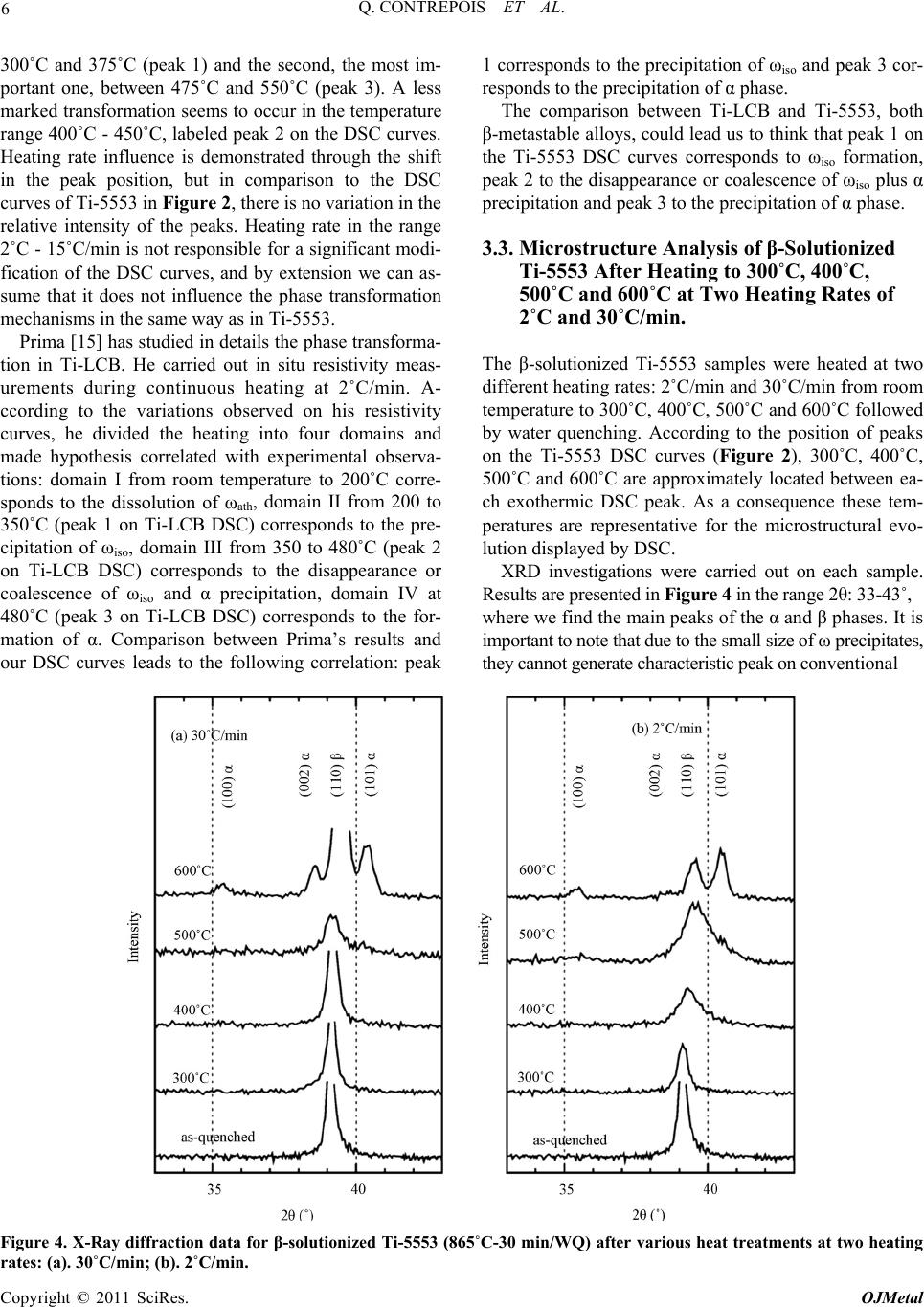 6 Q. CONTREPOIS ET AL. 300˚C and 375˚C (peak 1) and the second, the most im- portant one, between 475˚C and 550˚C (peak 3). A less marked transformation seems to occur in the temperature range 400˚C - 450˚C, labeled peak 2 on the DSC curves. Heating rate influence is demonstrated through the shift in the peak position, but in comparison to the DSC curves of Ti-5553 in Figure 2, there is no variation in the relative intensity of the peaks. Heating rate in the range 2˚C - 15˚C/min is not responsible for a significant modi- fication of the DSC curves, and by extension we can as- sume that it does not influence the phase transformation mechanisms in the same way as in Ti-5553. Prima [15] has studied in details the phase transforma- tion in Ti-LCB. He carried out in situ resistivity meas- urements during continuous heating at 2˚C/min. A- ccording to the variations observed on his resistivity curves, he divided the heating into four domains and made hypothesis correlated with experimental observa- tions: domain I from room temperature to 200˚C corre- sponds to the dissolution of ωath, domain II from 200 to 350˚C (peak 1 on Ti-LCB DSC) corresponds to the pre- cipitation of ωiso, domain III from 350 to 480˚C (peak 2 on Ti-LCB DSC) corresponds to the disappearance or coalescence of ωiso and α precipitation, domain IV at 480˚C (peak 3 on Ti-LCB DSC) corresponds to the for- mation of α. Comparison between Prima’s results and our DSC curves leads to the following correlation: peak 1 corresponds to the precipitation of ωiso and peak 3 cor- responds to the precipitation of α phase. The comparison between Ti-LCB and Ti-5553, both β-metastable alloys, could lead us to think that peak 1 on the Ti-5553 DSC curves corresponds to ωiso formation, peak 2 to the disappearance or coalescence of ωiso plus α precipitation and peak 3 to the precipitation of α phase. 3.3. Microstructure Analysis of β-Solutionized Ti-5553 After Heating to 300˚C, 400˚C, 500˚C and 600˚C at Two Heating Rates of 2˚C and 30˚C/min. The β-solutionized Ti-5553 samples were heated at two different heating rates: 2˚C/min and 30˚C/min from room temperature to 300˚C, 400˚C, 500˚C and 600˚C followed by water quenching. According to the position of peaks on the Ti-5553 DSC curves (Figure 2), 300˚C, 400˚C, 500˚C and 600˚C are approximately located between ea- ch exothermic DSC peak. As a consequence these tem- peratures are representative for the microstructural evo- lution displayed by DSC. XRD investigations were carried out on each sample. Results are presented in Figure 4 in the range 2θ: 33-43˚, where we find the main peaks of the α and β phases. It is important to note that due to the small size of ω precipitates, they cannot generate characteristic peak on conventional Figure 4. X-Ray diffraction data for β-solutionized Ti-5553 (865˚C-30 min/WQ) after various heat treatments at two heating rates: (a). 30˚C/min; (b). 2˚C/min. Copyright © 2011 SciRes. OJMetal
 7 Q. CONTREPOIS ET AL. XRD pattern as confirmed by Jones et al. [13]. In the β-solutionized state and after heating up to 300˚C, only the (110)β peak is visible. After heating up to 400˚C and 500˚C, we observe at the heating rate of 2˚C/min a wid- ening and a shift of this peak to higher values of 2θ. At the heating rate of 30˚C/min, the widening of (110)β is not observed at 400˚C but it is observed at 500˚C. At both heating rates, after heating to 600˚C, characteristic peaks of the α phase appear whilst the β peak is still visible. Between the β-solutionized state and the 600˚C heating, the position of the (110)β peak shift from 2θ = 39.1˚ to 2θ = 39.6˚. At 2˚C/min, XRD data show an evolution of the micro- structure between 300 and 500˚C. In this temperature range, no α phase is clearly detected as it is at 600˚C, so the widening of the (110)β peak could correspond either to the precipitation of ωiso or to the nucleation of very small α precipitates on the ω / β interface. Between 500 and 600˚C, XRD demonstrated the formation of the α phase. The micrographs in Figure 5 evidence that heating Ti-5553 from a β-solutionized state up to 600˚C at two different heating rates of 2 and 30˚C/min leads to differ- ent microstructures. At the 2˚C/min heating rate, the response of the alloy heated up to 600˚C to chemical etching consists of a Figure 5. LM micrographs of β-solutionized Ti-5553 (865˚C - 30 min/WQ) after various heat treatments at two heating rates: (a, b, c, d). 30˚C /min and (e, f, g, h). 2˚C /min. Intragranular precipitation is observed on microstructures a) and b) whereas only microstructures a) exhibit grain boundaries precipitation. Chemical etching leads to a darkening of surface of sample e) due to α precipitation. Copyright © 2011 SciRes. OJMetal
 8 Q. CONTREPOIS ET AL. complete darkening of the surface (Figure 5-(e)) making its observation by LM difficult. Between 300 and 500˚C, no change is observed by LM (Figure 5-(f) to (h)). At the 30˚C/min heating rate, precipitation is not uni- form, first precipitates appear inside the grains between 400 and 500˚C (Figure 5-(b)) whereas larger precipitates appear at the grain boundaries between 500 and 600˚C (Figure 5-(a)). The surface is darkened inside the grains whereas it is brighter at the grain boundaries. The preci- pitation occurring between 400 and 500˚C inside the grains at 30˚C/min (Figure5-(b)) is not observed at 2˚C/min (Figure 5-(f)). SEM micrographs (Figure 6) confirm LM observa- tions and reveal additional information. At 500˚C, at a heating rate of 30˚C/min (Figure 6-(c) and (d)), nano- meter size intragranular precipitates can be observed at high magnifications whereas nothing appears at the grain boundaries. Between 500 and 600˚C, the α precipitates appear both inside the grains and at the grain boundaries (Figure 6-(a) and (b)) but with different size. These pre- cipitates are plate shaped and form “triangle” patterns that are fine inside the grain and coarser at the grain boundaries (Figure 6-(a)). These α precipitates observed at 600˚C are termed “micron size α precipitates” in this paper and are defined as αM inside the matrix and αGB at grain boundaries. This difference in size of α precipitates between grain boundaries and rest the grain arises from the previous precipitation which takes place between 400 and 500˚C inside grains and not at the grain boundaries. We do not know the exact chemical and crystallographic nature of these small precipitates because XRD data do not prove the presence of α phase. However the micro- graphies evidence that they are the precursors of the pre- cipitation of αM precipitates. Thus we choose to call these - precipitates αMprec. At a heating rate of 2˚C/min, no precipitates are visi- ble at the quench temperature of 500˚C (Figure 6-(h)). The α phase precipitates homogeneously in the matrix Figure 6. SEM micrographs of β-solutionized Ti-5553 (865˚C - 30 min/WQ) after heat treatments up to 500˚C and 600˚C at two heating rates: (a-d). 30˚C /min and (e–h). 2˚C /min. (GB = grain boundaries). Copyright © 2011 SciRes. OJMetal
 9 Q. CONTREPOIS ET AL. and at the grain boundaries between 500 and 600˚C, and resultant α plates are fine and homogeneously distributed (Figure 6-(e) and (f)). We did not observe any difference between the grain boundaries and the centre of grains. The arrangements of the precipitates are different from those observed at 30˚C/min; they do not form triangle patterns but small plates. Hardness variations with temperature are illustrated in Figure 7. Hardness indentations were sufficiently small to differentiate between intragranular and grain boundary hardness when differences were highlighted on the mi- crographs at 30˚C/min. The comparison with XRD re- sults (Figure 4) shows that increase of hardness is con- comitant with the widening of the β XRD peak. At a heating rate of 2˚C/min, hardness increases between 300 Figure 7. Vickers hardness of β-solutionized Ti-5553 (865˚C -30 min/WQ) after heating at two heating rates: 30°C/min and 2˚C/min. and 400˚C, maximum is reached at 500˚C and slightly decreases with the appearance of micron size α precipi- tates at 600˚C. At a heating rate of 30˚C/min, when no precipitation is observed, i.e. 300˚C, 400˚C and at grain boundaries at 500˚C, the hardness remains low; when precipitation is observed, the hardness increases, i.e. in- side grains at 500˚C and everywhere at 600˚C. The pre- cipitation responsible for the increase in hardness at 30˚C/min is visible in LM after chemical etching wh- ereas this is not the case at 2˚C/min. 4. Discussion A summary of the results is given in Table 2. At 2˚C/min the DSC exothermic peak 1 is responsible- for a widening of the β XRD peak and an increase in hardness. According to experimental results from other authors [9,11], this peak could correspond to the trans- formation of ω to nanometer size α, this is not clear and it also could simply correspond to the formation of nanometer size ωiso. We assume the second hypothesis. Peak 3 corresponds unambiguously to the formation of micron size α precipitates. The homogeneous distribution of the micron size α indicates that ωiso precipitates formed during heating are responsible for the increase in hardness and act as nucleation sites for the micron size α formation (DSC peak 3). The following β phase decom- position is proposed: β+ωath → β → β+ωiso → β+α At 30˚C/min, DSC peak 1 is present but the difference with 2˚C/min arises from the appearance of DSC peak 2. Peak 2 corresponds to the formation of intragranular Table 2. Overview of all the experimental results at the two heating rates of 2˚C/min and 30˚C/min. Heat treatment Microstructure at micron scale (Figure 5 - 6) DSC (Figure2) XRD (Figure 4) Vickers Hardness (Figure 7) Supposed nanometer and micron scale microstructure evolution 600˚C fine micron size αMatrix and coarser micron size αGB exothermic peak 3 α + β peaks 409 (GB 378) fine precipitation of micron size αM inside grains nucleated on ωiso and αMprec and coarser micron size precipitation of αGB at GB 500˚C fine intragranular precipitation and PFZ at GB exothermic peaks 1 and 2 widening of β peak 406 (GB 281) ωiso formation (peak 1) then for- mation of αMprec inside grains (peak 2) but nothing at GB 400˚C β grains (area A) β peak 258 dissolution of ωath 30˚C /min 300˚C β grains (area A) β peak 258 dissolution of ωath 600˚C fine micron size α precipitates exothermic peak 3 α + β peaks 412 fine homogeneous precipitation of α nucleated on ωiso 500˚C β grains exothermic peak 3* widening of β peak 440 * 400˚C β grains exothermic peak 1 widening of β peak 398 ωiso formation 2˚C/m in 300˚C β grains (area A) β peak 264 dissolution of ωath PFZ = Precipitate-free zone, GB = grain boundaries, IntraG = Intragranular *According to the position of the 3rd exothermic peak, micron size α should have been observed. Copyright © 2011 SciRes. OJMetal
 Q. CONTREPOIS ET AL. 10 nanometer size precipitates which are visible after che- iso Mprec iso MGB n osr pr ce of heating rate in the range of 2) Heating 600˚C at 30˚C/min results in a fine intragranular distribution of m vents the e Walloon Region for fun- . J. Dashwood, D. Dye and M. Jackson, anical Processing of Ti-5Al-5Mo-5V-3Cr,” mical etching. Their exact nature is not known so we call them αMprec. Peak 3 corresponds to the formation of mi- cron size α precipitates, which are fine inside the grain (αM) and larger at the grain boundaries (αGB). Thus, two possible precursors exist at this heating rate: ωiso and αMprec, this lead to various mechanisms possible for the α phase precipitation and the following β phase decompo- sition is proposed: β+ωath → β → β+ω → β+α+ω → β+α+α It is likely that the precipitatiof ωiso phase retard o events the precipitation of αMprec. The ωiso precipitation is sufficiently high at 2˚C/min to inhibit the formation of αMprec corresponding to DSC peak 2. At faster heating rates (e.g. 10, 15 and 30˚C/min) the exothermic peak 2 is present between 450 and 500˚C so that the formation of the finely distributed intragranular precipitation of αMprec is not inhibited. Our results clearly show that α nucleation in Ti- 5553 has different possible mechanisms depending on the heating rate. Clément [18], who studied Ti-5553, was confronted to the same difficulties in interpreting the responses of the different techniques he used to study phase transformations in Ti-5553. He found that differ- ences in the peaks position may be observed with this alloy between various techniques so care should be taken with their interpretation. Due to the nanometer size of the particles, precise re- sponses could only be obtained with extensive and sys- tematic TEM analyses or/and with in situ high energy XRD. This will be the subject of future studies, espe- cially to verify if the αMprec precipitates could be an or- thorhombic α” phase as proposed by Bruneseaux et al. for the β phase decomposition of Ti17 [8]. . Conclusions 5 The strong influen 2–30˚C/min (= 0.033–0.5˚C/s) on the β phase decompo- sition of β-solutionized Ti-5553 was established using DSC analysis. Phase transformations were characterized by LM and SEM micrographs, XRD and hardness test. The results obtained are as follows. 1) Heating β-solutionized Ti-5553 up to 600˚C at 2˚C/min of results in a fine and homogeneous distribu- tion of α precipitates in the matrix. Formation of micron size α precipitates occurs between 500 and 600˚C. Be- fore 300˚C, ωath disappear and ωiso form between 300 and 400˚C. The ωiso precipitates act as nucleation sites for α plates precipitation. Following sequence is proposed: β+ωath → β → β+ωiso → β+α β-solutionized Ti-5553 up to icron size αM precipitates and a coarser precipitation of micron size αGB at the grain boundaries. The formation of ωiso is present between 350 and 450˚C but not suffi- ciently to inhibit the formation of nanometer precipitates called αMprec which takes place between 450 and 500˚C. Both ωiso and αMprec precipitates act as nucleation sites for α precipitation. Following sequence is proposed: β+ωath → β → β+ωiso →β+αMprec+ωiso →β+αM+αGB 3) The precipitation of ωiso phase retards or pre precipitation of αMprec. 4) Influence of slow heating rates on precipitation mechanisms cannot be generalized to all the β-metastable titanium alloys since Ti-LCB does not exhibit the same heating rate dependence on DSC curves. With these experimental observations, it is shown that the ω precipitates have a strong influence in the β to α phase transformation in Ti-5553 during a slow heating. Slow enough heating produces a fine and uniformly dis- tributed α precipitation. In this precipitation sequence, the main factor is the time necessary to form a high enough volume of ωiso precipitates. In an industrial proc- ess, the slow heating rate can reasonably be replaced by an isothermal treatment at 350˚C followed by a heating to 600˚C in order obtain a microstructure with a fine and homogeneous distribution of α plates. This technique co- uld be used to design new microstructures in the β-me- tastable alloys. 6. Acknowledgements The authors wish to thank th ding and support. 7. References [1] N. G. Jones, R “Thermomech Material Sciences and Engineering: A, Vol. 490, 2008, pp. 369-377. doi:10.1016/j.msea.2008.01.055 [2] I. Weiss and S. L. Semiatin, “Thermomechanical Proc- essing of Beta Titanium Alloys: An Overview,” Material Sciences and Engineering: A, Vol. 243, 1998, pp. 46-65. doi:10.1016/S0921-5093(97)00783-1 [3] G. Lütjering, “Influence of Processing on Microstructure and Mechanical Properties of (α+β) Titanium Alloys,” Material Sciences and Engineering: A, Vol. 243, 1998, pp. 32-45. doi:10.1016/S0921-5093(97)00778-8 [4] O. M. Ivasishin, P. E. Markovsky, S. L. Semiatin and C. H. Ward, “Aging Response of Coarse- and Fine-Grained β Titanium Alloys,” Material Sciences and Engineering: A, Vol. 405, 2005, pp. 196-305. doi:10.1016/j.msea.2005.06.027 Copyright © 2011 SciRes. OJMetal
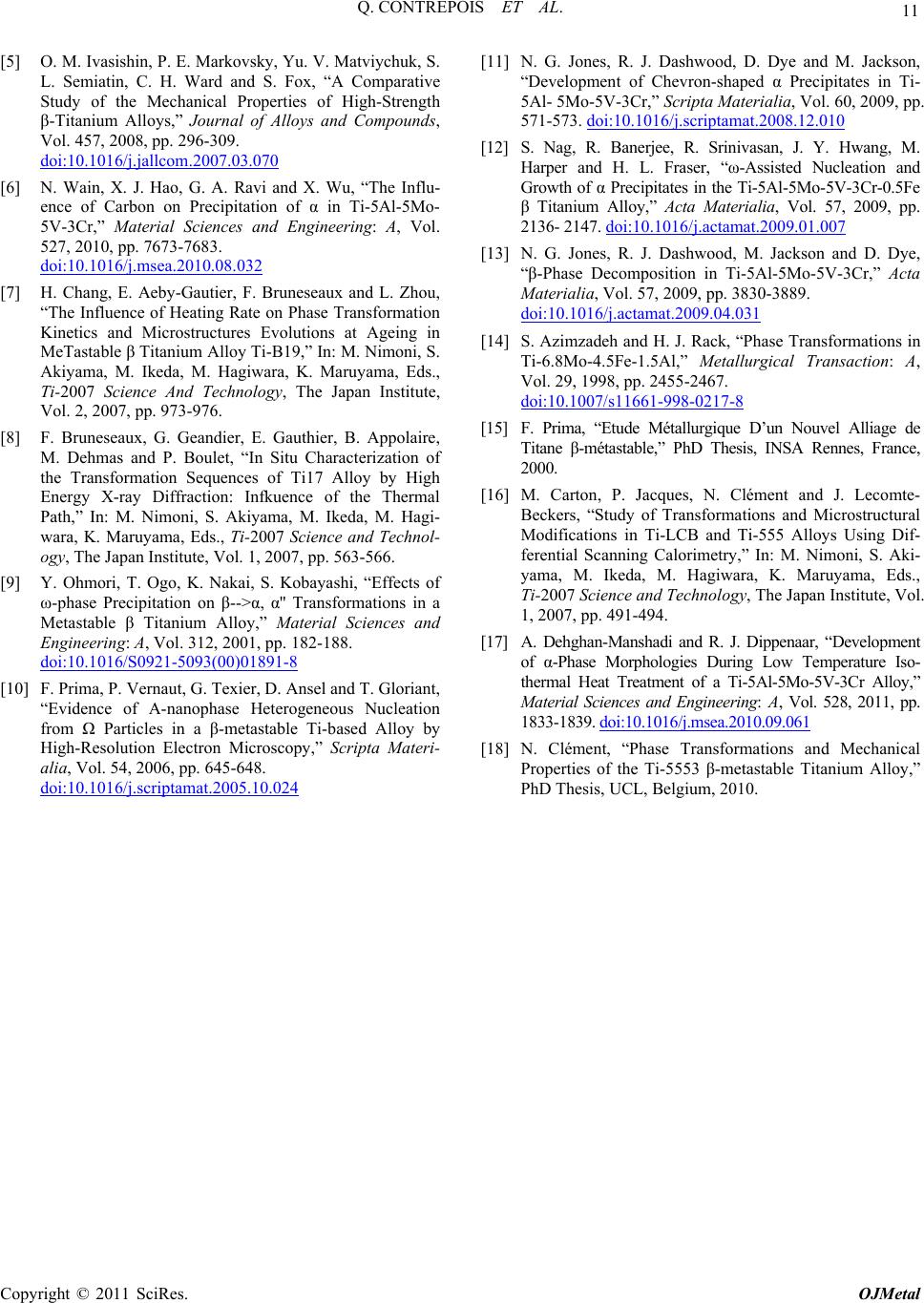 Q. CONTREPOIS ET AL. 11 es of High-Strength [5] O. M. Ivasishin, P. E. Markovsky, Yu. V. Matviychuk, S. L. Semiatin, C. H. Ward and S. Fox, “A Comparative Study of the Mechanical Properti β-Titanium Alloys,” Journal of Alloys and Compounds, Vol. 457, 2008, pp. 296-309. doi:10.1016/j.jallcom.2007.03.070 [6] N. Wain, X. J. Hao, G. A. Ravi and X. Wu, “The Influ- ence of Carbon on Precipita 5V-3Cr,” Material Sciences and tion of α in Ti-5Al-5Mo- Engineering: A, Vol. 527, 2010, pp. 7673-7683. doi:10.1016/j.msea.2010.08.032 [7] H. Chang, E. Aeby-Gautier, F. Bruneseaux and L. Zhou, “The Influence of Heating R Kinetics and Microstructures E ate on Phase Transformation volutions at Ageing in ces of Ti17 Alloy by High and MeTastable β Titanium Alloy Ti-B19,” In: M. Nimoni, S. Akiyama, M. Ikeda, M. Hagiwara, K. Maruyama, Eds., Ti-2007 Science And Technology, The Japan Institute, Vol. 2, 2007, pp. 973-976. [8] F. Bruneseaux, G. Geandier, E. Gauthier, B. Appolaire, M. Dehmas and P. Boulet, “In Situ Characterization of the Transformation Sequen Energy X-ray Diffraction: Infkuence of the Thermal Path,” In: M. Nimoni, S. Akiyama, M. Ikeda, M. Hagi- wara, K. Maruyama, Eds., Ti-2007 Science and Technol- ogy, The Japan Institute, Vol. 1, 2007, pp. 563-566. [9] Y. Ohmori, T. Ogo, K. Nakai, S. Kobayashi, “Effects of ω-phase Precipitation on β-->α, α'' Transformations in a Metastable β Titanium Alloy,” Material Sciences Engineering: A, Vol. 312, 2001, pp. 182-188. doi:10.1016/S0921-5093(00)01891-8 [10] F. Prima, P. Vernaut, G. Texier, D. Ansel and T. Gloriant, “Evidence of Α-nanophase Heterogeneous from Ω Particles in a β-metastable Nucleation Ti-based Alloy by High-Resolution Electron Microscopy,” Scripta Materi- alia, Vol. 54, 2006, pp. 645-648. doi:10.1016/j.scriptamat.2005.10.024 [11] N. G. Jones, R. J. Dashwood, D. Dye and M. Jackson, “Development of Chevron-shaped α Precipitates in Ti- 5Al- 5Mo-5V-3Cr,” Scripta Materialia, Vol. 60, 2009, pp. 571-573. doi:10.1016/j.scriptamat.2008.12.010 [12] S. Nag, R. Banerjee, R. Srinivasan, J. Y. Hwang, M. Harper and H. L. Fraser, “ω-Assisted Nucleation and Growth of α Precipitates in the Ti-5Al-5Mo-5V-3Cr-0.5Fe β Titanium Alloy,” Acta Materialia, Vol. 57, 2009, pp. 2136- 2147. doi:10.1016/j.actamat.2009.01.007 [13] N. G. Jones, R. J. Dashwood, M. Jackson and D. Dye, “β-Phase Decomposition in Ti-5Al-5Mo-5V-3Cr,” Acta Materialia, Vol. 57, 2009, pp. 3830-3889. doi:10.1016/j.actamat.2009.04.031 [14] S. Azimzadeh and H. J. Rack, “Phase Transformations in Ti-6.8Mo-4.5Fe-1.5Al,” Metallurgical Transaction: A, Vol. 29, 1998, pp. 2455-2467. doi:10.1007/s11661-998-0217-8 [15] F. Prima, “Etude Métallurgique D’un Nouvel Alliage de rton, P. Jacques, N. Clément and J. Lecomte- and R. J. Dippenaar, “Development Titane β-métastable,” PhD Thesis, INSA Rennes, France, 2000. [16] M. Ca Beckers, “Study of Transformations and Microstructural Modifications in Ti-LCB and Ti-555 Alloys Using Dif- ferential Scanning Calorimetry,” In: M. Nimoni, S. Aki- yama, M. Ikeda, M. Hagiwara, K. Maruyama, Eds., Ti-2007 Science and Technology, The Japan Institute, Vol. 1, 2007, pp. 491-494. [17] A. Dehghan-Manshadi of α-Phase Morphologies During Low Temperature Iso- thermal Heat Treatment of a Ti-5Al-5Mo-5V-3Cr Alloy,” Material Sciences and Engineering: A, Vol. 528, 2011, pp. 1833-1839. doi:10.1016/j.msea.2010.09.061 [18] N. Clément, “Phase Transformations and Mechanical Properties of the Ti-5553 β-metastable Titanium Alloy,” PhD Thesis, UCL, Belgium, 2010. Copyright © 2011 SciRes. OJMetal
|