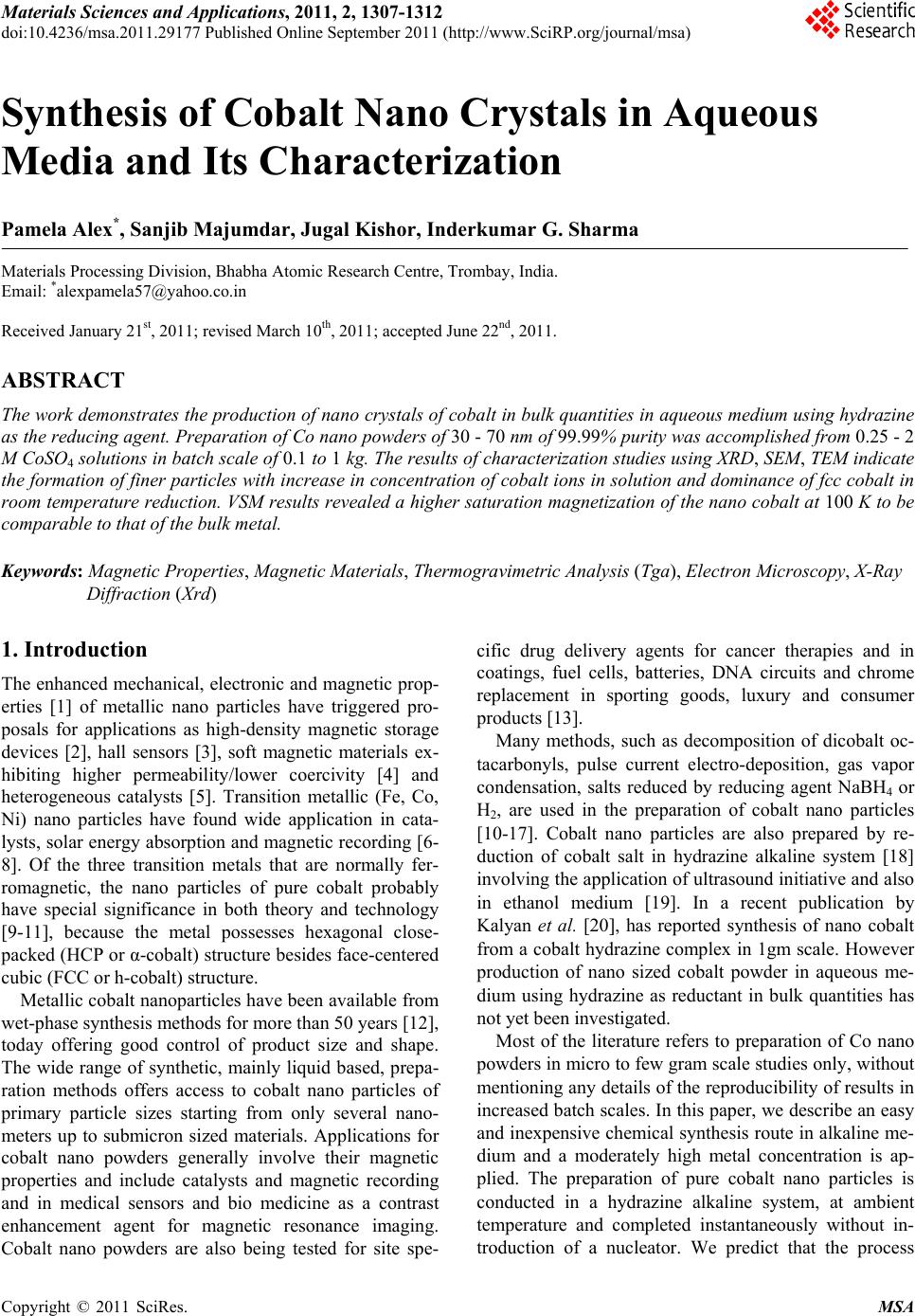 Materials Sciences and Applicatio ns, 2011, 2, 1307-1312 doi:10.4236/msa.2011.29177 Published Online September 2011 (http://www.SciRP.org/journal/msa) Copyright © 2011 SciRes. MSA 1307 Synthesis of Cobalt Nano Crystals in Aqueous Media and Its Characterization Pamela Alex*, Sanjib Majumdar, Jugal Kishor, Inderkumar G. Sharma Materials Processing Division, Bhabha Atomic Research Centre, Trombay, India. Email: *alexpamela57@yahoo.co.in Received January 21st, 2011; revised March 10th, 2011; accepted June 22nd, 2011. ABSTRACT The work demonstrates the production of nano crystals of cobalt in bulk quantities in aqueous medium using hydrazine as the reducing agent. Preparation of Co nano powders of 30 - 70 nm of 99.99% purity was accomplished from 0.25 - 2 M CoSO4 solutions in batch scale of 0.1 to 1 kg. The results of characterization studies using XRD, SEM, TEM indicate the formation of finer particles with increase in concentration of cobalt ions in solution and dominance of fcc cobalt in room temperature reduction. VSM results revealed a higher saturation magnetization of the nano cobalt at 100 K to be comparable to that of the bulk metal. Keywords: Magnetic Properties, Magnetic Materials, Thermogravimetric Analysis (Tga), Electron Microscopy, X-Ray Diffraction (Xrd) 1. Introduction The enhanced mechanical, electronic and magnetic prop- erties [1] of metallic nano particles have triggered pro- posals for applications as high-density magnetic storage devices [2], hall sensors [3], soft magnetic materials ex- hibiting higher permeability/lower coercivity [4] and heterogeneous catalysts [5]. Transition metallic (Fe, Co, Ni) nano particles have found wide application in cata- lysts, solar energy absorption and magnetic recording [6- 8]. Of the three transition metals that are normally fer- romagnetic, the nano particles of pure cobalt probably have special significance in both theory and technology [9-11], because the metal possesses hexagonal close- packed (HCP or α-cobalt) structure besides face-centered cubic (FCC or h-cobalt) structure. Metallic cobalt nanoparticles have been available from wet-phase synthesis methods for more than 50 years [12], today offering good control of product size and shape. The wide range of synthetic, mainly liquid based, prepa- ration methods offers access to cobalt nano particles of primary particle sizes starting from only several nano- meters up to submicron sized materials. Applications for cobalt nano powders generally involve their magnetic properties and include catalysts and magnetic recording and in medical sensors and bio medicine as a contrast enhancement agent for magnetic resonance imaging. Cobalt nano powders are also being tested for site spe- cific drug delivery agents for cancer therapies and in coatings, fuel cells, batteries, DNA circuits and chrome replacement in sporting goods, luxury and consumer products [13]. Many methods, such as decomposition of dicobalt oc- tacarbonyls, pulse current electro-deposition, gas vapor condensation, salts reduced by reducing agent NaBH4 or H2, are used in the preparation of cobalt nano particles [10-17]. Cobalt nano particles are also prepared by re- duction of cobalt salt in hydrazine alkaline system [18] involving the application of ultrasound initiative and also in ethanol medium [19]. In a recent publication by Kalyan et al. [20], has reported synthesis of nano cobalt from a cobalt hydrazine complex in 1gm scale. However production of nano sized cobalt powder in aqueous me- dium using hydrazine as reductant in bulk quantities has not yet been investigated. Most of the literature refers to preparation of Co nano powders in micro to few gram scale studies only, without mentioning any details of the reproducibility of results in increased batch scales. In this paper, we describe an easy and inexpensive chemical synthesis route in alkaline me- dium and a moderately high metal concentration is ap- plied. The preparation of pure cobalt nano particles is conducted in a hydrazine alkaline system, at ambient temperature and completed instantaneously without in- troduction of a nucleator. We predict that the process
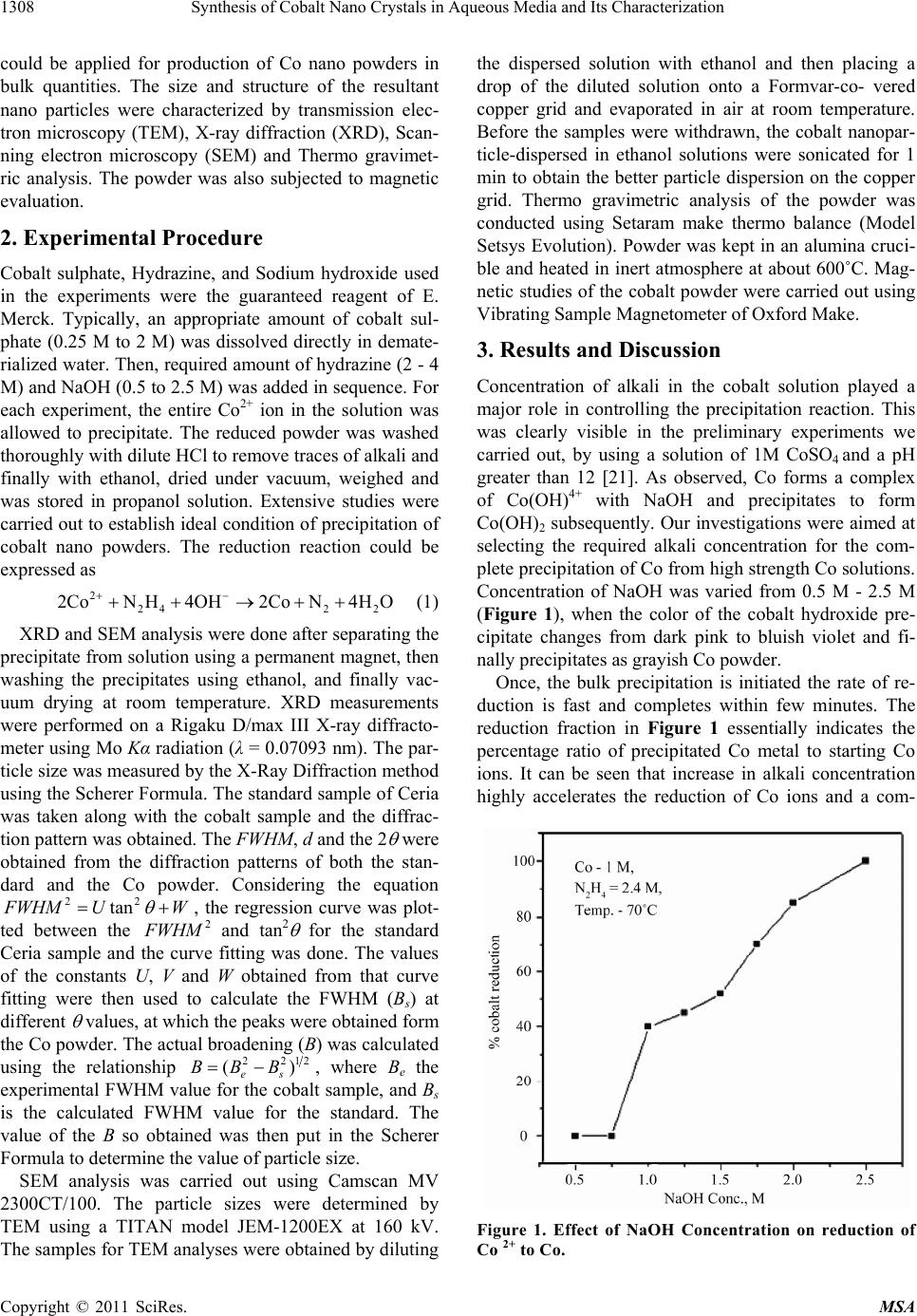 Synthesis of Cobalt Nano Crystals in Aqueous Media and Its Characterization 1308 could be applied for production of Co nano powders in bulk quantities. The size and structure of the resultant nano particles were characterized by transmission elec- tron microscopy (TEM), X-ray diffraction (XRD), Scan- ning electron microscopy (SEM) and Thermo gravimet- ric analysis. The powder was also subjected to magnetic evaluation. 2. Experimental Procedure Cobalt sulphate, Hydrazine, and Sodium hydroxide used in the experiments were the guaranteed reagent of E. Merck. Typically, an appropriate amount of cobalt sul- phate (0.25 M to 2 M) was dissolved directly in demate- rialized water. Then, required amount of hydrazine (2 - 4 M) and NaOH (0.5 to 2.5 M) was added in sequence. For each experiment, the entire Co2+ ion in the solution was allowed to precipitate. The reduced powder was washed thoroughly with dilute HCl to remove traces of alkali and finally with ethanol, dried under vacuum, weighed and was stored in propanol solution. Extensive studies were carried out to establish ideal condition of precipitation of cobalt nano powders. The reduction reaction could be expressed as 2 242 2 2CoNH 4OH2CoN 4HO (1) XRD and SEM analysis were done after separating the precipitate from solution using a permanent magnet, then washing the precipitates using ethanol, and finally vac- uum drying at room temperature. XRD measurements were performed on a Rigaku D/max III X-ray diffracto- meter using Mo Kα radiation (λ = 0.07093 nm). The par- ticle size was measured by the X-Ray Diffraction method using the Scherer Formula. The standard sample of Ceria was taken along with the cobalt sample and the diffrac- tion pattern was obtained. The FWHM, d and the 2 were obtained from the diffraction patterns of both the stan- dard and the Co powder. Considering the equation 22 tan WHM UW , the regression curve was plot- ted between the 2 WHM and tan2 for the standard Ceria sample and the curve fitting was done. The values of the constants U, V and W obtained from that curve fitting were then used to calculate the FWHM (Bs) at different values, at which the peaks were obtained form the Co powder. The actual broadening (B) was calculated using the relationship 221 ( es BBB 2 ), where Be the experimental FWHM value for the cobalt sample, and Bs is the calculated FWHM value for the standard. The value of the B so obtained was then put in the Scherer Formula to determine the value of particle size. SEM analysis was carried out using Camscan MV 2300CT/100. The particle sizes were determined by TEM using a TITAN model JEM-1200EX at 160 kV. The samples for TEM analyses were obtained by diluting the dispersed solution with ethanol and then placing a drop of the diluted solution onto a Formvar-co- vered copper grid and evaporated in air at room temperature. Before the samples were withdrawn, the cobalt nanopar- ticle-dispersed in ethanol solutions were sonicated for 1 min to obtain the better particle dispersion on the copper grid. Thermo gravimetric analysis of the powder was conducted using Setaram make thermo balance (Model Setsys Evolution). Powder was kept in an alumina cruci- ble and heated in inert atmosphere at about 600˚C. Mag- netic studies of the cobalt powder were carried out using Vibrating Sample Magnetometer of Oxford Make. 3. Results and Discussion Concentration of alkali in the cobalt solution played a major role in controlling the precipitation reaction. This was clearly visible in the preliminary experiments we carried out, by using a solution of 1M CoSO4 and a pH greater than 12 [21]. As observed, Co forms a complex of Co(OH)4+ with NaOH and precipitates to form Co(OH)2 subsequently. Our investigations were aimed at selecting the required alkali concentration for the com- plete precipitation of Co from high strength Co solutions. Concentration of NaOH was varied from 0.5 M - 2.5 M (Figure 1), when the color of the cobalt hydroxide pre- cipitate changes from dark pink to bluish violet and fi- nally precipitates as grayish Co powder. Once, the bulk precipitation is initiated the rate of re- duction is fast and completes within few minutes. The reduction fraction in Figure 1 essentially indicates the percentage ratio of precipitated Co metal to starting Co ions. It can be seen that increase in alkali concentration highly accelerates the reduction of Co ions and a com- Figure 1. Effect of NaOH Concentration on reduction of Co 2+ to Co. Copyright © 2011 SciRes. MSA
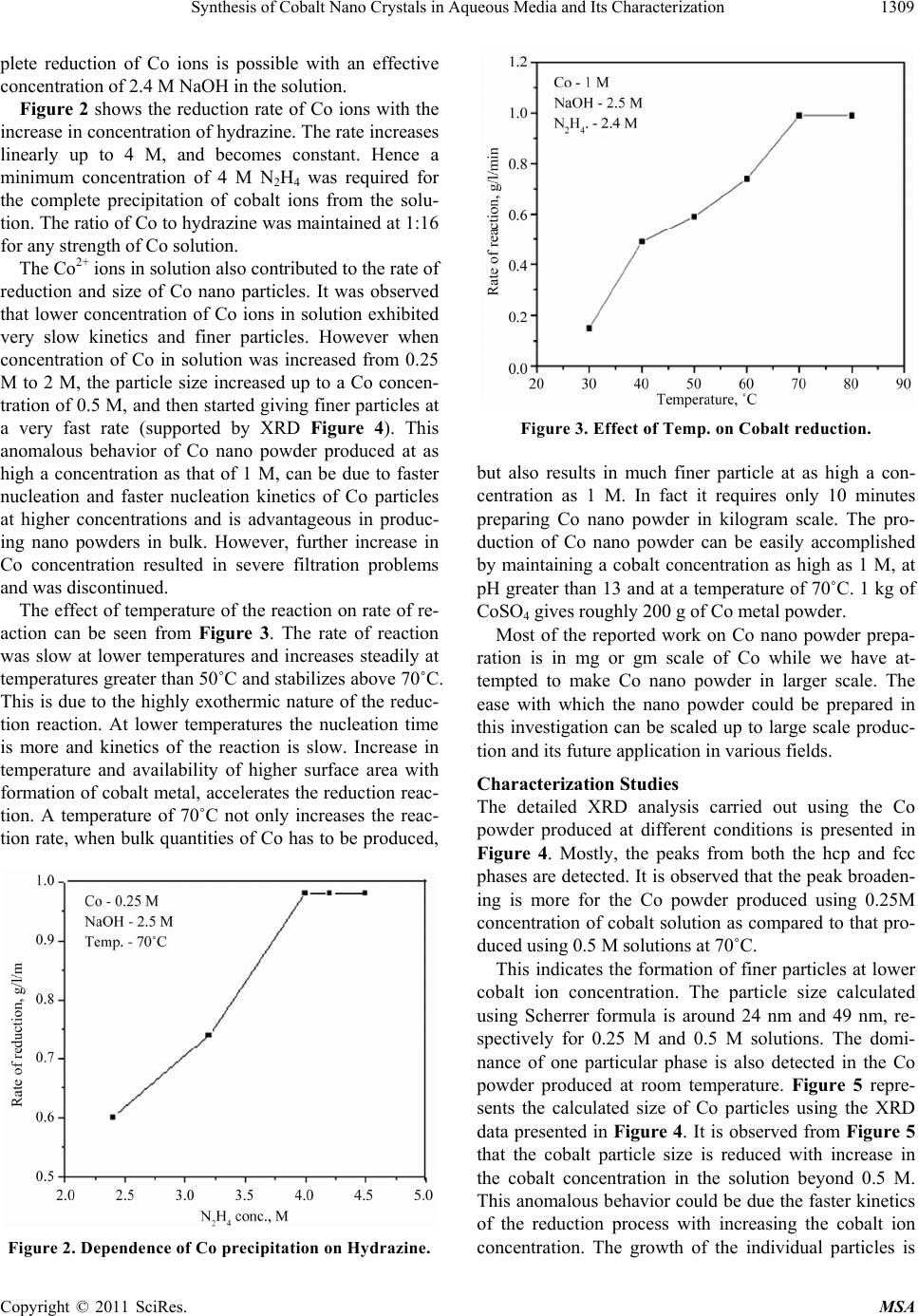 Synthesis of Cobalt Nano Crystals in Aqueous Media and Its Characterization1309 plete reduction of Co ions is possible with an effective concentration of 2.4 M NaOH in the solution. Figure 2 shows the reduction rate of Co ions with the increase in concentration of hydrazine. The rate increases linearly up to 4 M, and becomes constant. Hence a minimum concentration of 4 M N2H4 was required for the complete precipitation of cobalt ions from the solu- tion. The ratio of Co to hydrazine was maintained at 1:16 for any strength of Co solution. The Co2+ ions in solution also contributed to the rate of reduction and size of Co nano particles. It was observed that lower concentration of Co ions in solution exhibited very slow kinetics and finer particles. However when concentration of Co in solution was increased from 0.25 M to 2 M, the particle size increased up to a Co concen- tration of 0.5 M, and then started giving finer particles at a very fast rate (supported by XRD Figure 4). This anomalous behavior of Co nano powder produced at as high a concentration as that of 1 M, can be due to faster nucleation and faster nucleation kinetics of Co particles at higher concentrations and is advantageous in produc- ing nano powders in bulk. However, further increase in Co concentration resulted in severe filtration problems and was discontinued. The effect of temperature of the reaction on rate of re- action can be seen from Figure 3. The rate of reaction was slow at lower temperatures and increases steadily at temperatures greater than 50˚C and stabilizes above 70˚C. This is due to the highly exothermic nature of the reduc- tion reaction. At lower temperatures the nucleation time is more and kinetics of the reaction is slow. Increase in temperature and availability of higher surface area with formation of cobalt metal, accelerates the reduction reac- tion. A temperature of 70˚C not only increases the reac- tion rate, when bulk quantities of Co has to be produced, Figure 2. Dependence of Co precipitation on Hydrazine. Figure 3. Effect of Temp. on Cobalt reduction. but also results in much finer particle at as high a con- centration as 1 M. In fact it requires only 10 minutes preparing Co nano powder in kilogram scale. The pro- duction of Co nano powder can be easily accomplished by maintaining a cobalt concentration as high as 1 M, at pH greater than 13 and at a temperature of 70˚C. 1 kg of CoSO4 gives roughly 200 g of Co metal powder. Most of the reported work on Co nano powder prepa- ration is in mg or gm scale of Co while we have at- tempted to make Co nano powder in larger scale. The ease with which the nano powder could be prepared in this investigation can be scaled up to large scale produc- tion and its future application in various fields. Characterization Studies The detailed XRD analysis carried out using the Co powder produced at different conditions is presented in Figure 4. Mostly, the peaks from both the hcp and fcc phases are detected. It is observed that the peak broaden- ing is more for the Co powder produced using 0.25M concentration of cobalt solution as compared to that pro- duced using 0.5 M solutions at 70˚C. This indicates the formation of finer particles at lower cobalt ion concentration. The particle size calculated using Scherrer formula is around 24 nm and 49 nm, re- spectively for 0.25 M and 0.5 M solutions. The domi- nance of one particular phase is also detected in the Co powder produced at room temperature. Figure 5 repre- sents the calculated size of Co particles using the XRD data presented in Figure 4. It is observed from Figure 5 that the cobalt particle size is reduced with increase in the cobalt concentration in the solution beyond 0.5 M. This anomalous behavior could be due the faster kinetics of the reduction process with increasing the cobalt ion concentration. The growth of the individual particles is Copyright © 2011 SciRes. MSA
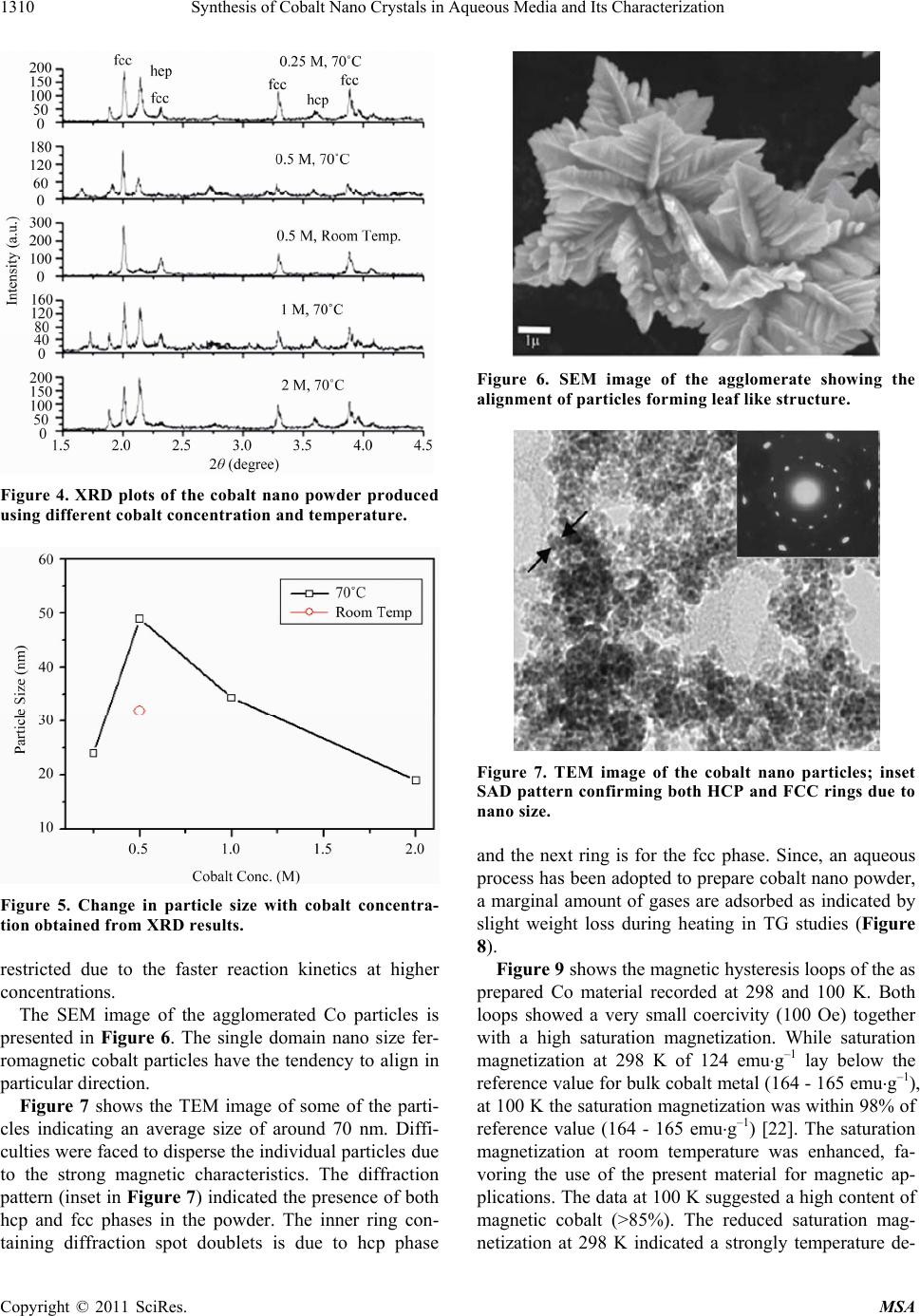 Synthesis of Cobalt Nano Crystals in Aqueous Media and Its Characterization 1310 Figure 4. XRD plots of the cobalt nano powder produced using di ffer ent c obal t co nce ntr atio n and te mper at ure. Figure 5. Change in particle size with cobalt concentra- tion obtained from XRD results. restricted due to the faster reaction kinetics at higher concentrations. The SEM image of the agglomerated Co particles is presented in Figure 6. The single domain nano size fer- romagnetic cobalt particles have the tendency to align in particular direction. Figure 7 shows the TEM image of some of the parti- cles indicating an average size of around 70 nm. Diffi- culties were faced to disperse the individual particles due to the strong magnetic characteristics. The diffraction pattern (inset in Figure 7) indicated the presence of both hcp and fcc phases in the powder. The inner ring con- taining diffraction spot doublets is due to hcp phase Figure 6. SEM image of the agglomerate showing the alignment of particles forming leaf like structure. Figure 7. TEM image of the cobalt nano particles; inset SAD pattern confirming both HCP and FCC rings due to nano size. and the next ring is for the fcc phase. Since, an aqueous process has been adopted to prepare cobalt nano powder, a marginal amount of gases are adsorbed as indicated by slight weight loss during heating in TG studies (Figure 8). Figure 9 shows the magnetic hysteresis loops of the as prepared Co material recorded at 298 and 100 K. Both loops showed a very small coercivity (100 Oe) together with a high saturation magnetization. While saturation magnetization at 298 K of 124 emug–1 lay below the reference value for bulk cobalt metal (164 - 165 emug–1), at 100 K the saturation magnetization was within 98% of reference value (164 - 165 emug–1) [22]. The saturation magnetization at room temperature was enhanced, fa- voring the use of the present material for magnetic ap- plications. The data at 100 K suggested a high content of magnetic cobalt (>85%). The reduced saturation mag- netization at 298 K indicated a strongly temperature de- Copyright © 2011 SciRes. MSA
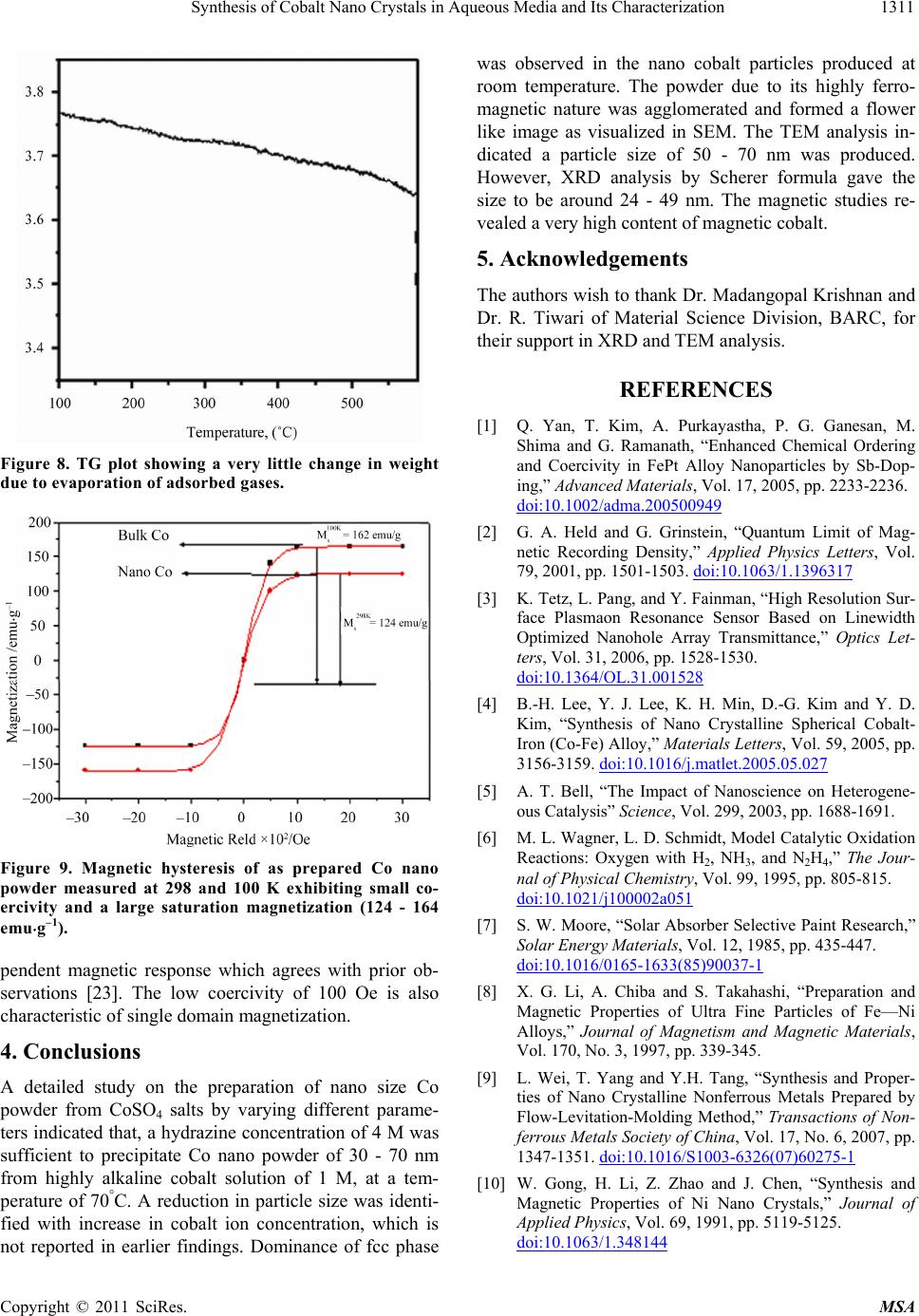 Synthesis of Cobalt Nano Crystals in Aqueous Media and Its Characterization1311 Figure 8. TG plot showing a very little change in weight due to evaporation of adsorbed gases. Figure 9. Magnetic hysteresis of as prepared Co nano powder measured at 298 and 100 K exhibiting small co- ercivity and a large saturation magnetization (124 - 164 emug–1). pendent magnetic response which agrees with prior ob- servations [23]. The low coercivity of 100 Oe is also characteristic of single domain magnetization. 4. Conclusions A detailed study on the preparation of nano size Co powder from CoSO4 salts by varying different parame- ters indicated that, a hydrazine concentration of 4 M was sufficient to precipitate Co nano powder of 30 - 70 nm from highly alkaline cobalt solution of 1 M, at a tem- perature of 70°C. A reduction in particle size was identi- fied with increase in cobalt ion concentration, which is not reported in earlier findings. Dominance of fcc phase was observed in the nano cobalt particles produced at room temperature. The powder due to its highly ferro- magnetic nature was agglomerated and formed a flower like image as visualized in SEM. The TEM analysis in- dicated a particle size of 50 - 70 nm was produced. However, XRD analysis by Scherer formula gave the size to be around 24 - 49 nm. The magnetic studies re- vealed a very high content of magnetic cobalt. 5. Acknowledgements The authors wish to thank Dr. Madangopal Krishnan and Dr. R. Tiwari of Material Science Division, BARC, for their support in XRD and TEM analysis. REFERENCES [1] Q. Yan, T. Kim, A. Purkayastha, P. G. Ganesan, M. Shima and G. Ramanath, “Enhanced Chemical Ordering and Coercivity in FePt Alloy Nanoparticles by Sb-Dop- ing,” Advanced Materials, Vol. 17, 2005, pp. 2233-2236. doi:10.1002/adma.200500949 [2] G. A. Held and G. Grinstein, “Quantum Limit of Mag- netic Recording Density,” Applied Physics Letters, Vol. 79, 2001, pp. 1501-1503. doi:10.1063/1.1396317 [3] K. Tetz, L. Pang, and Y. Fainman, “High Resolution Sur- face Plasmaon Resonance Sensor Based on Linewidth Optimized Nanohole Array Transmittance,” Optics Let- ters, Vol. 31, 2006, pp. 1528-1530. doi:10.1364/OL.31.001528 [4] B.-H. Lee, Y. J. Lee, K. H. Min, D.-G. Kim and Y. D. Kim, “Synthesis of Nano Crystalline Spherical Cobalt- Iron (Co-Fe) Alloy,” Materials Letters, Vol. 59, 2005, pp. 3156-3159. doi:10.1016/j.matlet.2005.05.027 [5] A. T. Bell, “The Impact of Nanoscience on Heterogene- ous Catalysis” Science, Vol. 299, 2003, pp. 1688-1691. [6] M. L. Wagner, L. D. Schmidt, Model Catalytic Oxidation Reactions: Oxygen with H2, NH3, and N2H4,” The Jour- nal of Physical Chemistry, Vol. 99, 1995, pp. 805-815. doi:10.1021/j100002a051 [7] S. W. Moore, “Solar Absorber Selective Paint Research,” Solar Energy Materials, Vol. 12, 1985, pp. 435-447. doi:10.1016/0165-1633(85)90037-1 [8] X. G. Li, A. Chiba and S. Takahashi, “Preparation and Magnetic Properties of Ultra Fine Particles of Fe—Ni Alloys,” Journal of Magnetism and Magnetic Materials, Vol. 170, No. 3, 1997, pp. 339-345. [9] L. Wei, T. Yang and Y.H. Tang, “Synthesis and Proper- ties of Nano Crystalline Nonferrous Metals Prepared by Flow-Levitation-Molding Method,” Transactions of Non- ferrous Metals Society of China, Vol. 17, No. 6, 2007, pp. 1347-1351. doi:10.1016/S1003-6326(07)60275-1 [10] W. Gong, H. Li, Z. Zhao and J. Chen, “Synthesis and Magnetic Properties of Ni Nano Crystals,” Journal of Applied Physics, Vol. 69, 1991, pp. 5119-5125. doi:10.1063/1.348144 Copyright © 2011 SciRes. MSA
 Synthesis of Cobalt Nano Crystals in Aqueous Media and Its Characterization Copyright © 2011 SciRes. MSA 1312 [11] E. Sowakai, M. Leonowiczi, B. Andrzejewski and G. I. Dzhardimaliev, “A Cobalt Nanoparticles Processed by Thermal Decomposition of Metal-Containing Mono- mers,” Materials Science-Poland, Vol. 24, No. 1-2, 2006, pp. 312-317. [12] G. N. Glavee, K. J. Klabunde, C. M. Sorensen, G. C. Hadjipanayis, “Sodium Borohydride Reduction of Cobalt Ions in Nonaqueous Media. Formation of ultrafine Parti- cles (Nanoscale) of Cobalt Metal,” Inorganic Chemistry, Vol. 32, No. 4, 1993, pp. 474-477. [13] E. Erb and A. M. El-Sherik, U.S. Patent, No. 5353266, 1994. [14] J.C. Sanchez-Lopez, A.R. Gonzalez-Elipe, A. Fernandez, “Passivation of Nano Crystalline Al Prepared by the Gas,” Journal of Materials Research, Vol. 13, No. 3, March 1998, pp. 703-707. [15] P. Saravanana, K. Raob, M. Premkumara and A. K. Sin- gha, “Spherical Granular Structures of Ag/Co Nanoparti- cles: Synthesis, Characterization and Magnetic Proper- ties,” Journal of Alloy and Compounds, Vol. 509, 2011, pp. 3880-3885. doi:10.1016/j.jallcom.2010.12.141 [16] K. Simeonidis, S. Mourdikoudis, A. Vilalta-Clemente, I. Tsiaoussis, M. Angelakeris and O. Kalogirou, “Shape and Composition Oriented Synthesis of Cobalt Nanoparti- cles,” Physics of Advanced Materials Winter School, 2008. [17] B. Q. Xie, Y. T. Qian, S. Y. Zhang, S. Q. Fu and W. C. Yu, “A Hydrothermal Reduction Route to Single-Crys- talline Hexagonal Cobalt Nanowires,” European Journal of Inorganic Chemistry, Vol. 12, No. 2, 2006, pp. 2454- 2459. [18] C. P. Gibson and K. J. Putzer, “Synthesis and Charecteri- zation of Anisotropic Co Nano Crystals,” Science, Vol. 267, 1995, pp. 1338-1340. [19] H.-G. Zheng, J. Lianga, J. Zenga and Y. Qiana, “Prepara- tion of Nickel Nanopowders in Ethanol-Water System (EWS),” Materials Research Bulletin, Vol. 36, No. 5-6, 2001, pp. 947-952. [20] S. S. Kalyan Kamal, P. K. Sahoo, M. Premkumarand and K. Chandra Sekar, “Synthesis of Co Nano Particles by a Modified Polyol Method,” Journal of Alloys and Com- pounds, Vol. 474, 2009, pp. 214-218. doi:10.1016/j.jallcom.2008.06.160 [21] S. S. Djokic, “Electron Microscope Study of the Nuclea- tion and Growth of Electroless Cobalt and Nickel,” Jour- nal of Electrochemistry, Vol. 7, July 1997, pp. 144-149. [22] R. Pauthenet, “Experimental Verification of Spin Wave Theory in High Fields,” Journal of Applied Physics, Vol. 53, 1982, pp. 8187-8190. doi:10.1063/1.330287 [23] C. M. Schneider, P. Bressler, P. Schuster, J. Kirschner, J. J. de Miguel, R Miranda and S Ferrer, “Characterization of New Materials by Surface-Sensitive Techniques,” Vac- uum, Vol. 41, 1990, pp. 503-505.
|