Journal of Surface Engineered Materials and Advanced Technology
Vol.2 No.2(2012), Article ID:18808,5 pages DOI:10.4236/jsemat.2012.22017
Potentiodynamical Research of Zn-Al-Mg Alloy System in the Neutral Ambience of NaCl Electrolyte and Influence of Mg on the Structure
1Department of Material Science and Engineering, Islamic Azad University, Majlesi Branch, Isfahan, Iran; 2Tajikistan Academy of Sciences, Chemistry Institute, Dushanbe, Tajikistan.
Email: reza.amini@mail.ru
Received October 24th, 2011; revised December 3rd, 2011; accepted February 25th, 2012
Keywords: Zn5Al; Zn55Al; Magnesium; NaCl Electrolyte; Corrosion Resistant
ABSTRACT
The results of anodic behavior of Zn5Al, Zn55Al alloys alloyed with Magnesium in the environment and their microstructures are studied. A potentiodynamical research carried out by Zinc-Aluminum alloys, alloyed with Magnesium obtained a corrosion-resistant coating with optimum concentration of Magnesium about 0.005 - 0.1 wt%. The rate of corrosion of these alloys is 2 - 2.5 times lower than Zn5Al, Zn55Al initial alloys. It is explained by the effect of modification on the structure of original alloys and they can be used as an anticorrosion coating for protecting corrosion of steel frames.
1. Introduction
Rapid growth in manufactured metal is accompanied by rapid growth in economic losses due to corrosion attack. The urgency of protecting metals against the corrosion is one of the oldest technical problems. It begins with the selection of material to generate products. Requirements for corrosion resistance of materials widely depend on the destination of the product, conditions of the working and the life of designed service. Products and structures which are made of metal. They are the most significant and valuable part of the fixed assets of any industrialized country and their protection from corrosion is a major problem. For these industries, corrosion resistance or chemical resistance of structural materials is one of the most important, and often the most vital characteristic that determines the reliability and service life of process equipment [1].
At present, almost, there is a large deficit in the non-ferrous metals in all industrialized countries. The most widely used metallic coating for the protection of corrosion of metals is Zinc (galvanize). It offers a very good combination of galvanic and barrier protection. Its excellent performance for many applications is well documented [2]. However, in the desire to improve, there is always a quest to find even better products. Researchers continually attempt to develop superior metal coatings that can be commercially applied. Often, the target is to find better products for specific end uses or environments, e.g. one is having superior corrosion resistance, or better coating formability. These attempts have met with little success most of the time, due either to an undesirable product attribute, or because manufacturing is too expensive and difficult [3]. In connection with making new alloys and introducing them into the technique, using of Zinc-Aluminum and their increased alloys especially in destructive environments which improves the corrosion resistance becomes relevant [4,5].
Although the literature on the protective materials of corrosion for natural weathering conditions is quite large, much less is known on the Zn-55Al (e.g. Galvalume) and Zn-5Al (e.g. Galfan) used for the building applications. It is generally accepted that these coatings performed better in marine and industrial atmospheres than hot-dip galvanised coatings with regards to general corrosion protection [6].
The research dedicated to study the influence of additive Magnesium on the anodic behavior of Zn5Al and Zn55Al alloys and their microstructures which designed for applying protective coatings in hot methods.
2. Experimental Procedure
Raw materials, which are used in the research are Aluminum A7 grade, granular Zinc C.D.A grade, and granular pure Magnesium. These alloys were obtained in crucibles, which made of Aluminum oxide in a shaft furnace of CSHOL model in the temperature range of about 750˚C - 850˚C. Alloys were casted in a graphite mold with a diameter of 8 and 140 mm. Non-operating parts of samples were isolated with a resin (a mixture of 50% rosin and 50% paraffin). Ends of the working parts were cleaned out with sandpaper, polishing, degreasing and were etched in 10% solution of NaOH. They were washed thoroughly with alcohol and then immersed in a solution of NaCl. The temperature of solution in the cell was maintained constantly (20˚C) with a MLSH-8 thermostat. Reference electrodes were a saturated silver chloride with a subsidiary—Pt.
The anodic behavior of Zn5Al and Zn55Al alloyed with Magnesium was carried out in NaCl electrolytes with concentrations of 0.03%, 0.3% and 3% in PI-50.1.1, potentiostat with a 2 mV/sec potential velocity by the method which Ganiev has described [7]. Current-free potential set under submerging in electrochemical studies of anodic polarized potentiodynamic samples before a sharp increase in current because of pitting formation. then samples were polarized in the opposite direction to the potential of 1400 mV and passive electrode layers were made consequently. Finally, the samples were polarized again in a positive direction. Extrapolation of the cathodic curve was conducted to –1.600 - –1.800 V potential. The current corrosion density was carried out to a main electrochemical process of corrosion, which was determined by the cathodic curve that was taken to the tofely straight line is equal to bк = 0.124 V [8]. As an example, Figure 1 shows the potentiodynamic polarization curve of the Zn55Al alloy, which was alloyed with 0.05 wt% of additive Magnesium. Calculation of the current corrosion density (i, A/m2) was carried out by the following equations:
Figure 1. Potentiodynamic polarization curve of the Zn55Al alloy which contains of 0.05 wt% Magnesium in 3% solution of NaCl. E—potential (V), i—current density (A·m–2).
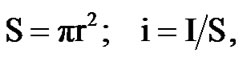
where S is the working surface of round specimen, m2; I is the current strength of corrosion, A. Corrosion rate is a function of current corrosion, which was found by:
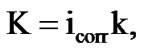
where k is electrochemical equivalent, the numerical value, which for Zinc is 1.22 (for the Zn5Al alloy) and 0.778 g/A∙h is the average of Zinc (1.22) and Aluminum (0.335) for the Zn55Al alloy, which half of it is made of Aluminum [9].
Generally, accelerated corrosion test assisted to obtain comparative data of the corrosion resistance of alloys that was carried out in accordance with GOST 9.017-86. The tests were conducted on samples of identical size and shape, by the surface preparation, cutting the sample in one direction to the direction of casting. Surface of sample was degreased with acetone, etched with 10% sodium hydroxide solution at a temperature of 55˚C for 1 - 2 minutes. Then the samples were washed with the same water, clarified in 30% nitric acid solution for 2 - 3 minutes and again washed in the distilled water at a temperature of 70˚C - 90˚C and were dried.
The corrosion resistance of alloys was evaluated by weighing on an analytical balance with an accuracy more than 0.0001 g before and after the tests in a solution of 3% NaCl before removing corrosion products. Removed corrosion products were produced at a temperature of 95˚C - 98˚C and were held for 10 - 30 min in a solution contained of 35 ml of 85% phosphoric acid, 20 g of chromic anhydride and 945 ml of distilled water. After removing corrosion products, samples were washed in tap water, then in distilled water, were dried in an oven at 105˚C ± 2˚C for 5 minutes, were placed in a desiccators for 24 hours and then were weighed. Lost corrosion of the sample (Δm) in g/m2 was calculated by the formula:
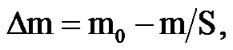
where m0 is the mass of the sample before the test, m is the mass of the sample after removed corrosion products, S is the sample surface before the test (m2). The corrosion rate (K) in g/m2∙h was calculated with the formula:
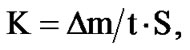
where it is the duration of the tests per hour. Character of the corrosion was determined visually. Metallographical studies were carried out by preparing surface, specimens were cut to cylindrical shape with 1 cm × 1 cm sizes then they were sanded, polished, etched and dried then the test was carried out by AIS2100 Scanning Electron Microscope.
3. Results and Discussion
The change in the corrosion potential of Zn5Al alloy, with additive Magnesium in the time in the ambience of 3% NaCl electrolytes is shown in Figure 2. It was fixed within an hour, which shows that the first minute of immersion of the alloy in the solution was occurred with a sharp changing potential in the positive region. The concentration of chloride ions in the electrolyte corrosion potential (–Ecor) decreases. It indicates that the corrosion resistance of the alloy in a NaCl ambience has been decreased. Certainly, chemical composition of all alloys mentioned to a sharp changing potential over the time in the positive region, describes dynamic formation of the protective oxide film just ends in 35 minutes from the start of immersion of the electrode into the electrolyte and little depends on their chemical composition. Also the dependence were observed in the Zn55Al alloy, which has been contained of Magnesium in various concentrations in the studied environments, 0.03%, 0.3% and 3% solutions of NaCl. The results are listed in Table 1.
However, for Zn55Al alloy with additive Magnesium, the change in the potential of steady state on time shows the formation of protective oxide film in 45 minutes (Table 1).
Studied results of anodic behavior of Zn5Al and Zn55Al alloys, with additive Magnesium has been presented in Tables 2 and 3, which indicate that the additive Magnesium in the ranges of about 0.005 - 0.1 wt% Shifts corrosion potentials of Zn5Al and Zn55Al source alloys to the positive area. However, increasing the content of Magnesium in the alloys up to 2.0 wt% shifts Ecorr to the negative area, therefore, the visible event due to shift pitting (Ept) and repassive potential (Erp) to the negative direction. The above mentioned features were observed in three studied ambiences.
Figure 2. Change in the free potential of corrosion Ecorr (V) in time τ (min) for Zn5Al alloys (1), contained of Magnesium (2 - 8) in the electrolyte ambience of 3% NaCl. CMg (wt%): (2) 0.005; (3) 0.01; (4) 0.05; (5) 0.1; (6) 0.5; (7) 1.0; (8) 2.0.
Table 1. Change in the free potential of corrosion (–Efree.corr, V) for Zn55Al alloy with additive Magnesium in time in the ambience of NaCl electrolytes.
Table 2. Electrochemical characteristics of corrosion of the Zn5Al alloy with additive Magnesium in the ambience of NaCl electrolytes.
Table 3. Electrochemical characteristics of corrosion of the Zn55Al alloy with additive Magnesium in the ambience of NaCl electrolytes.
Figure 3. Microstructures (300×) of (a) Zn55Al original alloys, (b) with 0.01 Mg, (c) Zn5Al original alloys and (d) with 0.01 Mg.
According to Tables 2 and 3, when the concentration of chloride ions, corrosion and pitting potentials and the rate of corrosion of Zn5Al and Zn55Al alloys increase the additive Magnesium decreases, which indicates a decrease in corrosion resistance of alloys under the influence of chloride ions. Therefore the alloys with additive Magnesium has lower corrosion rates than the original Zinc-Aluminum alloys (Tables 2 and 3), which confirm the gravimetric study. Particularly, the corrosion rate of the original alloy which is defined by this method was 0.40 - 0.42 g/m2∙hour, and an average of three determinations for the containing of 0.1% Mg alloy is K = 0.49 - 0.51 g/m2∙hour.
According to the phase diagram of Al-Zn-Mg at the ambience temperature [10], the structure of Zn55Al alloy consists of α-Al + γ-Zn (Figure 3(a)). When it is alloyed with magnesium, three-phase are formed in amounts of more than 1% which consist of α-Al + γ-Zn phases and υ-Mg. As an example, Figure 3(b) shows the microstructure of Zn55Al alloy which contains 0.01% magnesiums. The composition of phases does not go beyond twophase region which is α-Al + γ-Zn. However, the additive magnesium dissolved in α-Al and γ-Zn solid and greatly refines the structure and transforms it from a needle shape to spherical structure. The role of Mg is modifying Zn55Al alloys structure.
The Zn5Al alloy has different phase ratios of α-Al and γ-Zn from Zn55Al alloy, it means that the content of γ-Zn is significantly higher than Zn55Al alloy. The mechanism of action of magnesium is the same for both alloys. Thus dissolved magnesium does not form new phases, but it plays like a modifier role for the structure (Figures 3(c)-(d)).
Generally, the studied electrochemical research of Zn5Al and Zn55Al alloys with additive Magnesium determined a coating corrosion resistant with optimum concentrations of Magnesium about 0.005 - 0.1 wt%. Corrosion rates of these alloys are 2 - 2.5 times lower than the original alloys and they can be used as an anodic coatings to protect corrosion of steel structures.
4. Conclusion
The regularities of changing have been established in the electrochemical characteristics of Zn5Al and Zn55Al alloys with additive Magnesium in NaCl solutions with concentrations of 0.03%, 0.3% and 3%. Particularly, we showed that increasing concentration of chloride ions in the electrolyte contributes to decrease corrosion potential. It has been determined that the potential of the pitting repassiving with increasing concentration of elements in alloys were shifted to the positive area, and with increaseing concentration of chloride ions in the solution, were shifted to the negative area. The corrosion rate of Zn5Al and Zn55Al source alloys decrease 2 - 2.5 times in order to modify grains by adding Magnesium to 0.1% which transform needle shape to spherical structure.
5. Acknowledgements
This work was supported by department of Material Science and Engineering form Islamic Azad University, Majlesi Branch, Isfahan, Iran.
REFERENCES
- A. M. Shluger, F. F. Azhogin and E. A. Efimov, “Corrosion and Protection of Metals,” Metallurgia, Moscow, 1981.
- K. Asano and K. Hirano, “Precipitation Process in an AlZn-Mg Alloy,” Transactions of the Japan Institute of Metals, Vol. 9, 1968, pp. 24-34.
- I. J. Polmear, “Light Alloys—Metallurgy of the Light Metals,” St. Edmundsbury Press Ltd., Suffolk, 1995.
- A. P. Smiryagin, “Industrial of Non-Ferrous Metals and Alloys,” Metallurgia, Moscow, 1981, p. 560.
- V. A. Kechin and E. Y. Lublin, “Zinc Alloys,” Metallurgia, Moscow, 1986, p. 247.
- H. Dafydd, D. A. Worsley and H. N. Mc Murray, “The Kinetics and Mechanism of Cathodic Oxygen Reduction on Zinc and Zinc-Aluminium Alloy Galvanized Coatings,” Corrosion Science, Vol. 47, No. 12, 2005, pp. 3006- 3018. doi:10.1016/j.corsci.2005.05.036
- I. N. Ganiev and T. M. Umarova, “Corrosion of Double Aluminium Alloys in the Neutral Ambience,” Danish, Dushanbe, 2007.
- V. Romanov, “Methods of Studying Corrosion of Metals,” Metallurgiya, Moscow, 1965, p. 249.
- J. M. Kolotyrkin, “Metal and Corrosion,” Metallurgia, Moscow, 1985.
- N. R. Bochvar and E. S. Kadaner, “The Phase Diagrams of Systems Based on Aluminum and Magnesium Handbook,” Nauka, Moscow, 1977.