 Circuits and Systems, 2016, 7, 622-629 Published Online May 2016 in SciRes. http://www.scirp.org/journal/cs http://dx.doi.org/10.4236/cs.2016.76053 How to cite this paper: Suriyakrishnaan, K. and Sridharan, D. (2016) Critical Data Delivery Using TOPSIS in Wireless Body Area Networks. Circuits and System s, 7, 622-629. http://dx.doi.org/10. 4236/cs.2016.76053 Critical Data Delivery Using TOPSIS in Wireless Body Area Networks K. Suriyakrishnaan, D. Sridharan Department of ECE, College of Engineering, Anna University, Guindy Campus, Chennai, India Received 16 March 2016; accepted 3 May 2016; published 6 May 2016 Copyright © 2016 by authors and Scientific Research Publishing Inc. This work is licensed under the Creative Commons Attribution International License (CC BY). http://creativecommons.org/licenses/by/4.0/ Abstract Technology development in the Wireless Sensor Network (WSN) has paved the way for body area network for remote health monitoring. For th i s purpose, a message or a dat a packet should be transmitted from the patient to t he me dic al evaluator without any loss. In th is work, Technique for Order of Preference by Similar ity to Ideal Solution (TOPSIS) algorithm is implemented for Wireless Body Area Networks (WBAN) to deliver the message, based on the criticality of t he pa- tient. Fi nally, we compare the results for both the re al -ti me d ata and the si mu late d (random) val- ues in terms of packet-size forwarding for different parameters such as Packet Delivery Rati o (PDR), Delay and Throughput. The result shows better performance in the real ti me v alue by u si ng TOPSIS scheme in the WBAN. By improvi ng the quality of service in body area networks, health monitoring system can be automated with good perfo rm ance. Keywords E-Health, Packet Siz e, Reliab ili ty, TOPSIS, WBAN, WSN 1. Introduction The main cause of demise in the world is due to lack of proper attention in emergency situation these days. So the growth of the sensor nodes in the emerging world has paved the way for health monitoring to improve the WBAN for ubiquitous monitoring. The health car e system is more important in modern days. The elderly people and the patient’s health data should be transmitted in an efficient manner for continuous monitoring for a long time [1] [2]. It is essential in day care and government hospitals to monitor the real-time data from patient’s body such as Blood pressure (BP), Electrocardiogram (ECG), Electromyocardiogram (EMG), and SPO2 for oxygen saturation to measure the oxygen in haemoglobin [1]. Th e s e collected data from the patient should be transmitted through controller node to the base station and the info rmation can be shared by the internet services. From that, the doctor or the medical evaluator/care giver can evaluate the health details of the particular critical
 K. Suriyakrishnaan, D. Sridharan patient and do th eir medical needs to save the patien t life. This will in turn benefit the patient in general hospital and day care. 1.1. Motivation More chronic health diseases are associated with changing lifestyle behaviour due to the physical inactivity and poor dietary choices. The critical data from the patient’s body has to be transmitted in a reliable manner without affecting the actual observed body parameters to the medical evaluator [3] [4]. So, in this case, finding the criti- cal data is more important for selecting the patient with abnormalities to detect and diagnose the patient to save their life through e-health [5]. 1.2. Contribution This paper work addresses the reliable monitoring bas ed allocation of time slots bas ed on the emergency/criti- cality of the patient’s health data. The contribution of the paper is that the time critical health data should be found out by the decision making algorithm. Here we implement the T OP SI S [6] developed originally by Hwang and Yoon in 1981 for selecting the abnormal value and allocating slots in the controller node based on the urgency of the patient’s health information. According to it, time slot is allocated and the critical data is transmitted by the controller node through the base station (BS) to the receiver side (medical server). The infor- mation is obtained by the medical evaluator/doctor through the internet. 2. Related Works Sudip misra et al. [3] proposed a Priority based Allocation of Time Slots (PATS) algorithm which considers the emergency health data to be transmitted to the LDPU based on the seriousness and urgency. However, the au- thor does not highlight the decision making algorithm for implementation. Lahby et al. [6] proposed a Multiple Analytic Hierarchy Process (M-AHP) which chooses the ideal solution but the author did not use the real time health data for the simulation for getting optimal so lution. In Body Area Networks (BAN), health parameters that are observed from the body are transmitted u s in g wak eu p radio concept [7]. Here the data packets are imparted by emergency communication. In our work, the critical health data are disseminated by selecting the abnormalities of the patient body health parame t ers. For this intent we implement the TOPSIS scheme for selecting the ideal solution. During the transmission process the information or the data packets from the patient is disseminated by relay- ing method [8]. H owever the author does not enlighten the delay, thro ughput, packet size and normalized over- heads. Misra et al. [4] also proposed a data rate tuning mechanism to improve the quality of service in BAN. In Nag anawa et al. [9], the path loss can be provoked by more number of body nodes which are placed in different postures of human body that are generated by time domain method and the performance is ev a l uated using two hop relaying scheme. So for any information in BAN, data should be highly reliable [10]. Medium Access Control (MAC) layer improvement is done by using fuzzy logic system, thereby achieving quality of service and better energy consumption in BAN by fuzzy logic system [10]. The topology and the BAN architecture for on body and in body communication is illustrated in MAC, PHY and Network layer [11], [9] and ongoing r esear ch are highlighted [12]. A stochastic link cost formulation is developed in [13]. Ludoric et al. [14] proposed a performance evaluation scheme for BAN with blocking effects using one bounce model for their evaluation. So while designing the BA N , the obstacle size should be considered. Samant a et al. [15] proposed a quality link establishment for allocation with balancing load in BAN network. During the transmission of data packets, the delay factor should be considered for strict requirement of the reliable and efficient BAN. For this, ch angyen et al. [16] proposed a compatible mechanism for medical data with emergency transmission in E-Health net- works. 3. Network Model and Arc hitecture The wire le ss body area network IEEE 802.15.6 (WBAN) is intended to support the ubiquitous monitoring for rural and urban people. The ultimate moto of the BAN is to detect the abnormalities in a patient’s body and to transmit immediately for diagnosis. The network model is given in Figure 1.
 K. Suriyakrishnaan, D. Sridharan Figure 1. WBAN communication architecture. 3.1. System Model In our proposed system there are several sensors mounted on a human body. For example, ECG, EEG, BP and EMG are placed in appropriate positions of the human body that continuously sense the information from the patient and transmit to the controller node. Here the body sensors are placed at the minimum distance required to avoid interference issue and these collected information is aggregated and sent to the controller node. The con- troller node will take the decision according to the patient’s critical situation ba sed on the observed health pa- rameters. More number of data packets are arriv ed at the controller node and so collision problem may occur. For that, Carrier Sense Multiple Access with Collision Avoidance (CSMA/CA) [17] is employed to provide Collison free transmission based on the emergency and detailed method for routing protocol is illustrated [1] [18] for packet forwarding. Th e controller node will take the decision based on the critical data packets of the pa- tient’s medical history an d take decision based on the TOPSIS scheme and transferred to the medical server. From this server the patient information is shared to care giver or medical evaluator/ doctor for diagnosis through the internet and save the risk of the patient life. 3.2. Simulation Scenario Here the biosensors such as ECG, EMG, BP and SPO2 sensors are placed on human body for observing the bio signals. Data aggregation is done by controller node. Wireless channel model of IEEE 802.15.6 has been con- side red as channel model with initial energy of 100 J. The path loss for this channel is being considered from Two Ray Ground Propagation model. The transmission and receiving power are 0.02 J and 0.01 J respectively. Omni directional antenna has been chose for a network diameter of 30 m. The simulation is carried out using Network Simulator-2 (NS-2). Simulated results has been discussed in the following section. 4. Proposed System The wireless body area network is deployed in a predetermined structure. The human body is monitored by dif- ferent types of sensor namely EEG, ECG, EMG and BP. These sensors are connected by controller node and hence the sensed data from all sensors are reported to controller node whenever the emergency data is generated.
 K. Suriyakrishnaan, D. Sridharan In lowest level sensor communicates with controller node and which in turn connects with the medical server in the next level. In next higher level the server is connected with internet to forward the received data from con- troller node. In the same level the proxy serve r is connected with internet to accomplish the multihop communi- cation with controller nod es to form this architecture. The server send s announcement message as periodical broadcast message to communicate with internet and internet node sends broadcast message as periodical message. Receiving controller nodes create the communica- tion with server via internet. Then controller nodes broadcast controller announcement message with its location in wireless medium. Receiver nodes checks for nearest node by verifying location, distance and stores the near- est controller node id. All the sensor nodes maintain its local controller unit id to forward the sensed data. Once the structure is formed, each sensor nodes performs sensing process, and forward the data to its local controller unit. The controller node collects the sens ed data from all connected sensors and forward the emer- gency data to the server via internet. The algorithm for the proposed system is given below. In our proposed system, the patient’s health information is collected through biosensors and then it is given as input to the TOPSIS algorithm. From this the evaluation matrix is formed followed by the normalization and calculation of the weight. Once the normalized weighted decision matrix is formed, thresholds for both upper and lower limit should be found out. From that calculate the target distance and find out whether the data is critical or not. If it is critical, forward the data immediately to the medical server. If it is not, discard the data packet. 5. Result and Discussion In this work, the real value of TOPSIS is compared with simulated value. The real values used for the simulation is observed from patients in nearby hospital based on a survey conducted by us. Destination Sequenced Distance Vector (DSDV) routing protocol is employed in this simulation. By comparing simulated data with real time value, real time values will outperform in terms of PDR, Throughput, Delay, Jitter, Dropping ratio, and Packets dropped. The following graphs are plotted based on the critical data packet transmission from the patient to the medical evaluator. Figure 2 shows a constant difference between TOPSIS simulated value and TOPSIS real value. With increase in packet size, the Packet Delivery Ratio (PDR) starts increasing initially and later decreases and TOPSIS real value achiev es maximum (99%) reliability. PDR can be calculated by the ratio of the number of delivered data packet to the destination. This illustrates the level of delivered data to the destination. Comparatively TOPSIS real value has greater PDR than the TOPSIS value. Figure 3 shows a constant difference between TOPSIS value and TOPSIS real value. With increase in packet size, the dropping ratio is slightly increasing. The dropping ratio can be measured by averag e number of packets dropped during the transmission. So comparatively TOPSIS real value has lesser dropping ratio when compared with the TOPSIS value. Figure 4 shows a constant difference between TOPSIS value and TOPSIS real value. With increase in packet size, the delay is slightly increasing. The delay of a network specifies the duration it takes for a bit of data to travel across the network from one node to end point or another. Typically it can be calculated in multiples or fractions of seconds. So, TOPSI S real value has lesser delay when compared with the TOPSIS value.
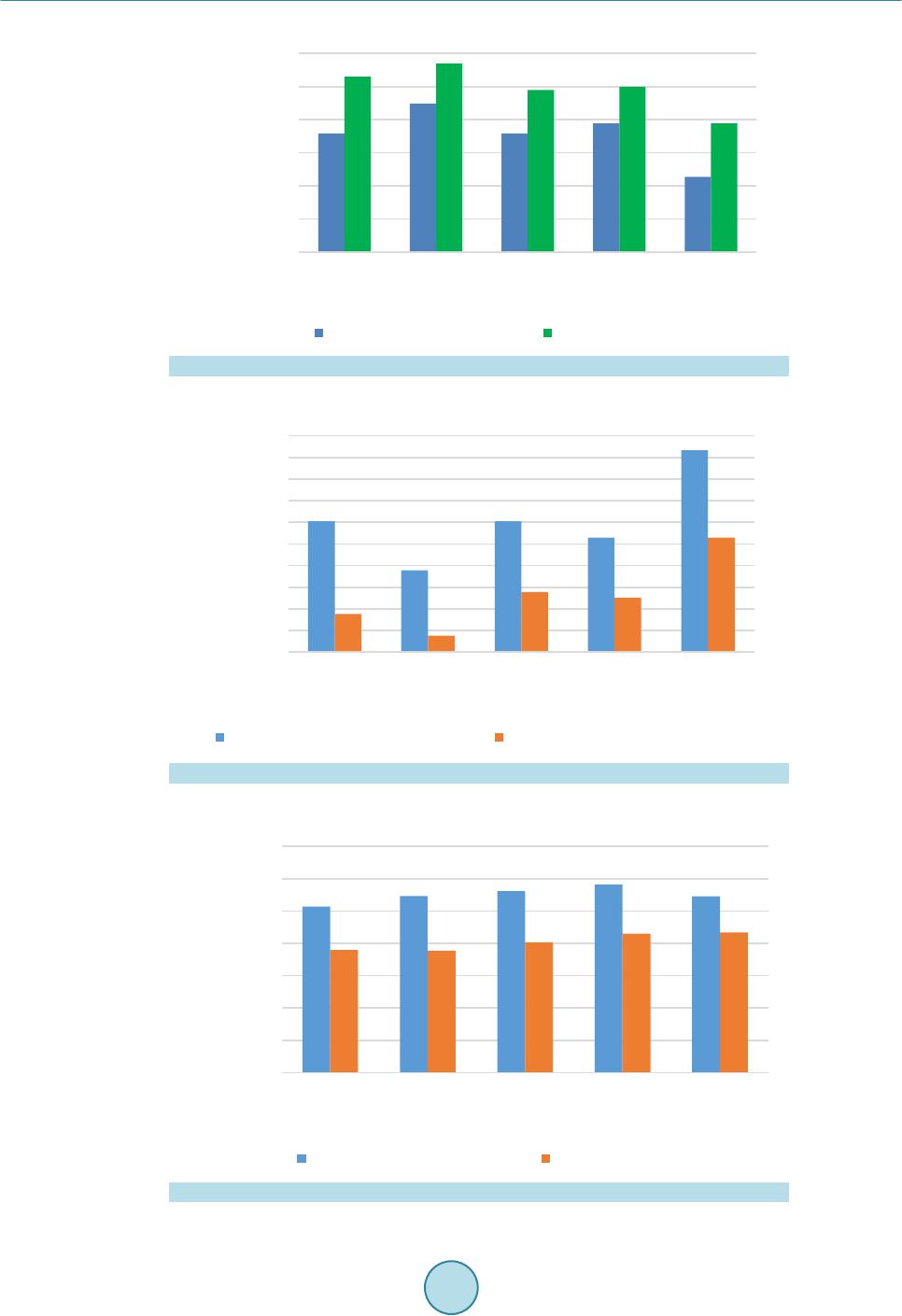 K. Suriyakrishnaan, D. Sridharan Figure 2. Packet size vs PDR. Figure 3. Packet size vs dropping ratio. Figure 4. Packet size vs delay. 0 0.2 0.4 0.6 0.8 1 1.2 1.4 1.6 1.8 2 Packet size Vs Dropping Ratio Dro pping Ratio TO PSI S Sim ulated Dropping Ratio TOPSIS Real 0 0.02 0.04 0.06 0.08 0.1 0.12 0.14 1800 1900 2000 2100 2200 Delay ( m s) Pack et size (bytes) Packet size Vs Delay Dela y TOPSIS S imulatedDelay TOPSIS Real
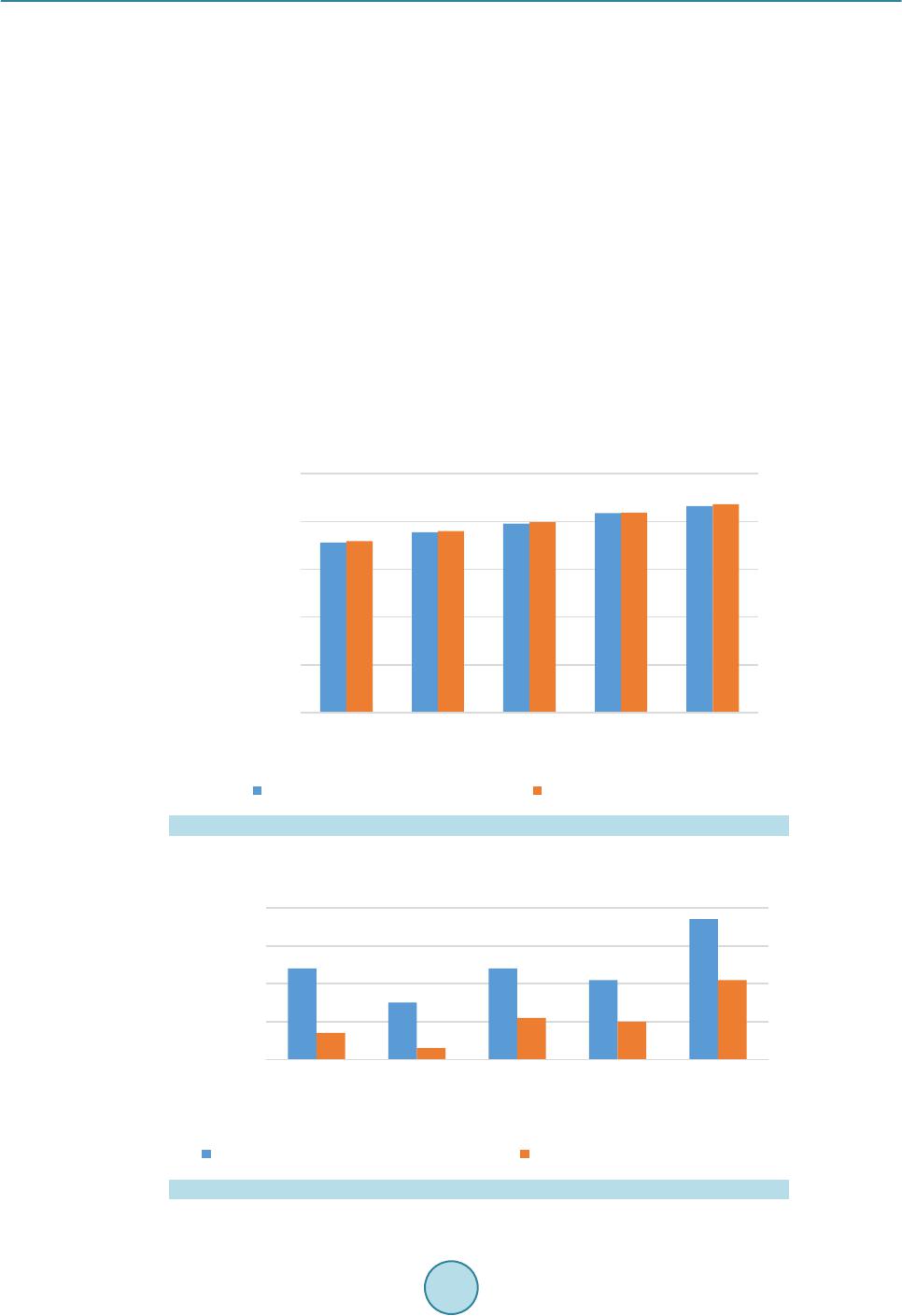 K. Suriyakrishnaan, D. Sridharan Figure 5 shows that there is no difference between TOPSIS value and TOPSIS real value for throughput. With increase in packet size, the throughput is slightly increasing and achieves maximum level. Throughput is the amount of work that a node can do in a given time period. Typically, throughput has been a measure of the comparative effectiveness of dense network that run many tasks concurrently. So comparatively TOPSIS real value and the simulated value has same throughput values. Figure 6 shows a constant difference between simulated value and TOPSIS real value. With increase in packet size, the packets dropped is slightly increasing and are less in real time value. The packets dropped is the ratio of packet loss with respect to the packet sent. So, TOPSIS real value has lesser packets dropped than the TOPSIS value. Figure 7 shows a constant difference between TOPSIS value and TOPSIS real value. With increase in packet size, the jitter is slig htly increasing and later become constant for real value. The jitter period in a network is the interval between two times of maximum effect of a data characteristic that varies regularly with time at receiver end. So comparativ ely TOPSIS real value has lesser jitter tha n the TOPSIS value. 6. Conclusion In this paper, we proposed TOPSIS scheme implementation for the continuous monitoring for patients health in hospitals and day care. The critical data should be given preference during transmission in order to avoid casualty. This work utilizes the TOPSIS algor ithm in finding the cr iticality of the data. The simulation is carr ied Figure 5. Packet size vs throughput. Figure 6. Packet size vs packets dropped. 0 50000 10000 0 15000 0 20000 0 25000 0 1800 1900 2000 2100 2200 Throughput (bytes/sec) Pack et size (bytes) Packet size Vs Throughput Throughput TOPSIS SimulatedThroughput TOPSIS Real 0 10 20 30 40 1800 1900 2000 2100 2200 Packets Dropped (%) Pack et size (bytes) Packet size Vs Packets Dropped Packets Dropped TOPSIS SimulatedPackets Dropped TOPSIS Real
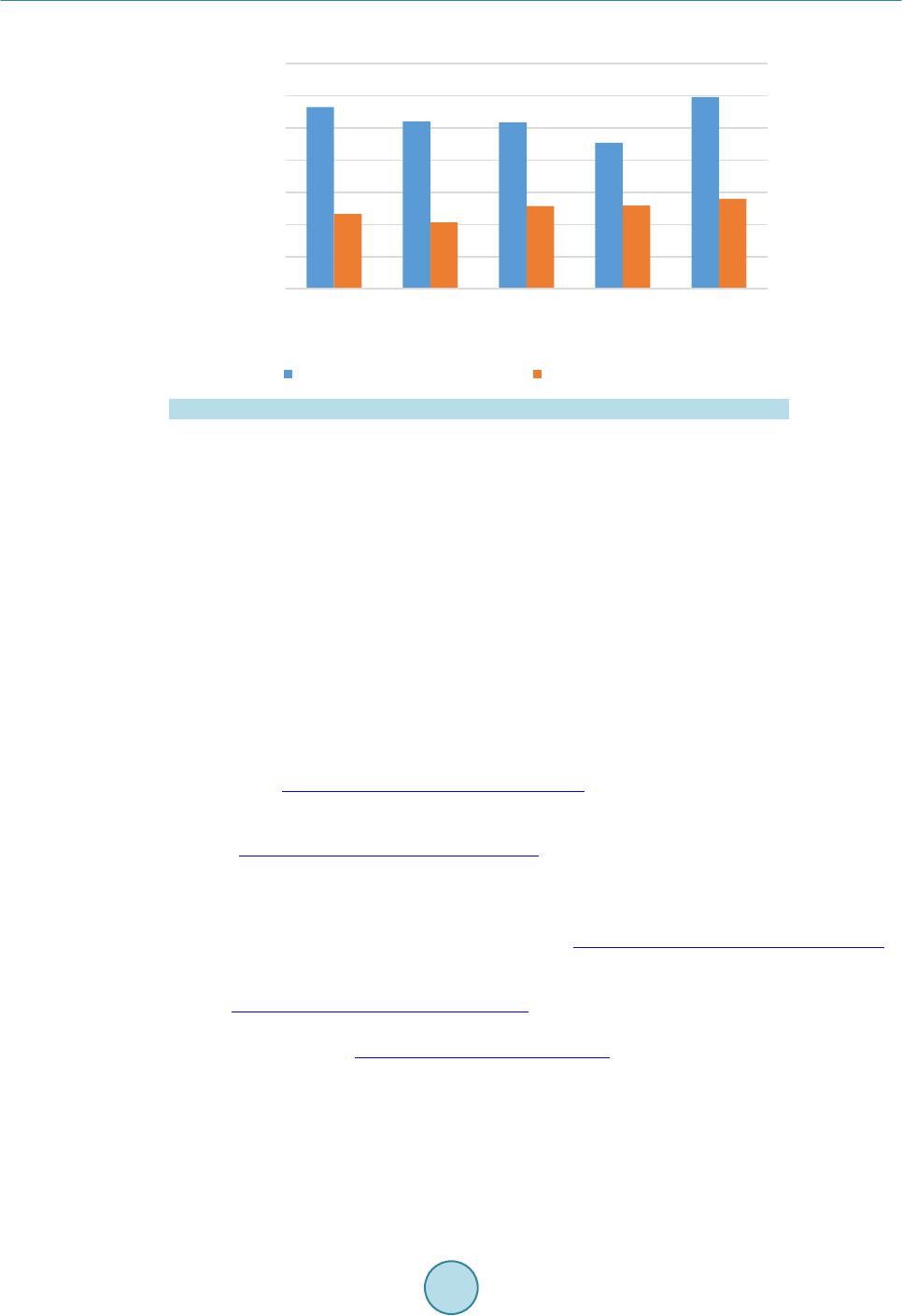 K. Suriyakrishnaan, D. Sridharan Figure 7. Packet size vs jitter. out for the real time data observed from the hospital based on a survey observed by us and compared with ran- domly (simulated) generated data. The TOPSIS algorithm in wireless body area network performs well for transmitting the critical data packets from patients to the doctor/caregiver in terms of PDR, Delay, Dropping ra- tio, Packets dropped, Jitter and throughput. Finally the maximum amount of critical data packets (99 % of PDR) is delivered from source to the destination, increasing the reliability of the algorithm in BAN. Acknowledgements We acknowledge the support provided under the Anna Centenary Res earch Fellowship (ACRF) scheme of Anna University, Chennai and the cooperation given by the patients of the surrounding locality. References [1] Sharavanan, P.T., Kumar, R. and Sridharan, D. (2015) A Comparative Stu dy of Cross Layer Protocols in WBAN. Aus- tralian Journal of Basic and Applied Sciences, 9, 294-300. [2] Lim, C.Y. and Kim, K. (2014) A Stu dy on Healthcare Syst em Using Smart Clothes. Journal of Electrical Engineering & Technology, 9, 372-377. http://dx.doi.org/10.5370/JEET.2014.9.1.372 [3] Misra, S. and Sarkar, S. (2015) Priority-Based Time-Slot Allocation in Wireless Body Area Networks during Medical Emergency Situations: An Evolutionary Game-Theoretic Perspective. IEEE Journal of Biomedical and Health Infor- matics, 19, 541-548. http://dx.doi.org/10.1109/jbhi.2014.2313374 [4] Misra, S., Moulik, S. and Chao, H.-C. (2015) A Cooperative Bargaining Solution for Priority-Based Data-Rate Tuning in a Wireless Body Area Network. IEEE Transactions on Wireless Communications, 14, 2769-2777. [5] Kim, S.-K., Kim, T.-K. and Koh, J.-H. (2013) Energy Efficient Wireless Data Transmission for Personal Health De- vices. Journal of Electrical Engineering & Technology, 8, 1559-1570. http://dx.doi.org/10.5370/JEET.2013.8.5.1559 [6] Lahby, M., Cherkaoui, L. and Adib, A. (2013) Hybrid Network Selection Strategy by Using M-AHP/E-TOPSIS for Heterogeneous Networks. The 8th International Conference on Intelligent Systems: Theories and Applications (SITA), 8-9 May 2013, 1-6. http://dx.doi.org/10.1109/sita.2013.6560784 [7] Al Ameen, M. and Hong, C. (2015) An On-Demand Emergency Packet Transmission Scheme for Wireless Body Area Networks. Sensors, 15, 30584-30616. http://dx.doi.org/10.3390/s151229819 [8] Pan, R., Chua, D.J., Pathmasuntharam, J.S. and Xu, Y.P. (2015) An Opportunistic Relay Protocol with Dynamic Scheduling in Wireless Body Area Sensor Network. IEEE Sensors Journal, 15, 3743-3750. [9] Suriyakrishnaan, K. and Sridharan, D. (2014) A Review of Reliable and Secure Communication in Wireless Body Area Networks. Proceedings of Thirteenth IRF International Conference, Chennai, 14 September 2014, 32-44. [10] Otal, B., Alonso, L. and Verikoukis, C. (2009) Highly Reliable Energy-S aving Mac for Wireless Body Sensor Net- works in Healthcare Systems. IEEE Journal on Selected Areas in Communications, 27, 553-565. 0.078 0.079 0.08 0.081 0.082 0.083 0.084 0.085 1800 1900 2000 2100 2200 Jitter (bits/sec) Pack et size (bytes) Packet size Vs Jitter Jitter TOPSIS SimulatedJitter TOPSIS Real
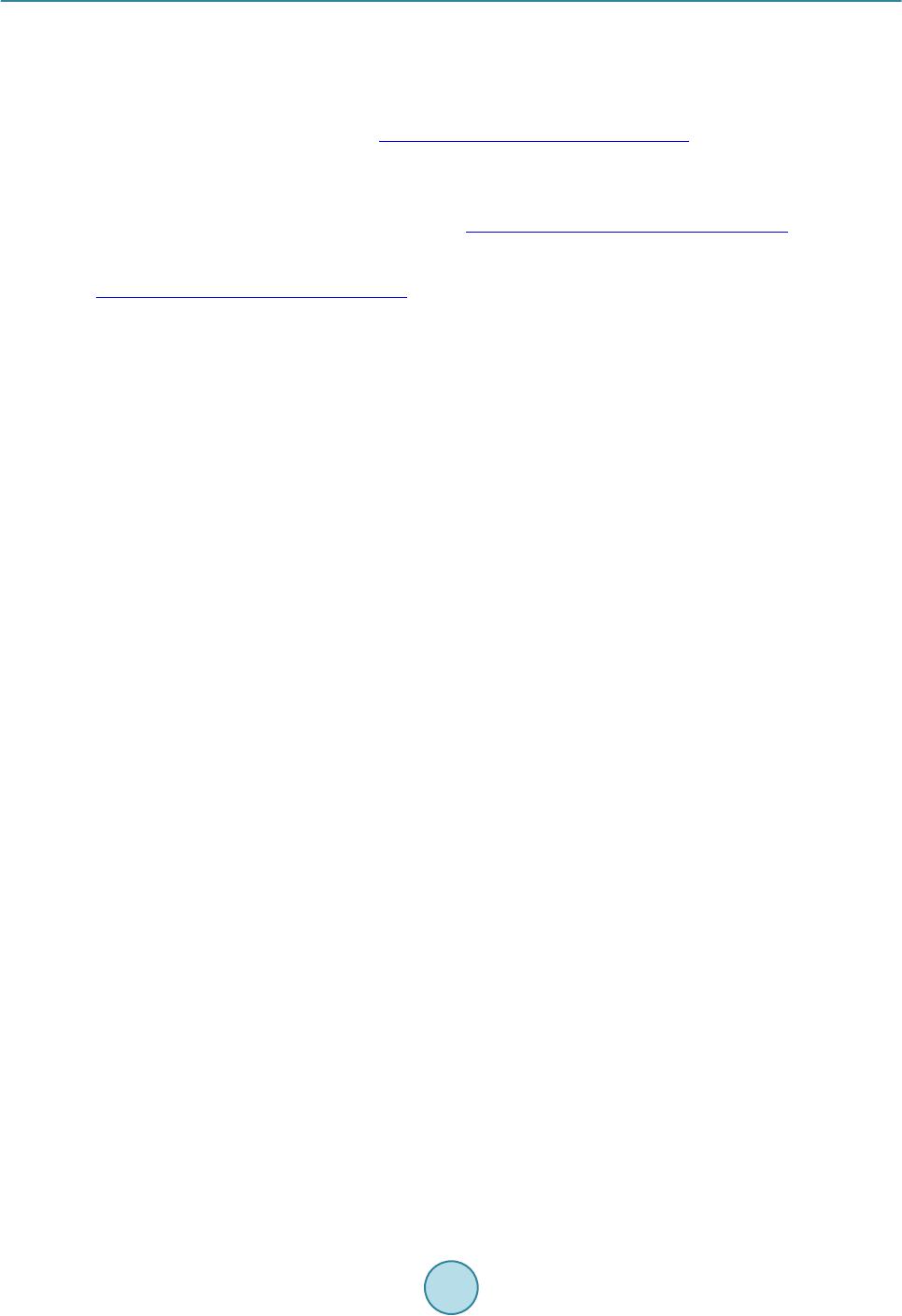 K. Suriyakrishnaan, D. Sridharan [11] Ullah, S., Higgins, H., Braem, B., Latre, B., Blondie, C., Merman, I., Saleem, S., Rahman, Z. and Kwak, K.S. (2012) A Comprehensive Survey of Wireless Body Area Networks. Journal of Medical Systems, 36, 1065-1094. [12] Cao, H.S., Leung, V., Chow, C. and Chan, H. (2009) Enabling Technologies for Wireless Body Area Networks: A Survey and Outlook. IEEE Communications Magazine, 47, 84-93. [13] Quwaider, M. and Biswas, S. (2010) DTN Routing in Body Sensor Networks with Dynamic Postural Partitioning. Journal of Ad Hoc Networks, 8, 824-841. http://dx.doi.org/10.1016/j.adhoc.2010.03.002 [14] Chevalier, L., Sahuguede, S. and Julien-Vergonjanne, A. (2015) Performance Evaluation of Wireless Optical Commu- nication for Mobile Body Area Network Scenario with Blocking Effects. Optoelectronics, IET, 9, 211-217. [15] Samanta, A., Bera, S. and Misra, S. (2015) Link-Quality-Aware Resource Allocation with Load Balance in Wireless Body Area Networks. IEEE Systems Journal, PP, 1-8. http://dx.doi.org/10.1109/JSYST.2015.2458586 [16] Yi, C. , Alfa, A.S. and Cai, J. (2015) An Incentive-Compatible Mechanism for Transmission Scheduling of Delay-Sen- sitive Medical Packets in E-Health Networks. IEEE Transactions on Mobile Computing, PP, 1-13. http://dx.doi.org/10.1109/TMC.2015.2500241 [17] Chen, M., Gunzalez-Valenzuela, S., Vasilakos, A., Cao, H.S. and Leung, V.C.M. (2011) Body Area Netwo rks: A Sur- vey. Journal of Mobile Networks and Applications—MONET, 16, 171-193. [18] Movassaghi, S., Abolhasan, M. and Lipman, J. (2013) A Review of Routing Protocols in Wireless Body Area Net- works. Journal of Networks, 8, 559-575.
|