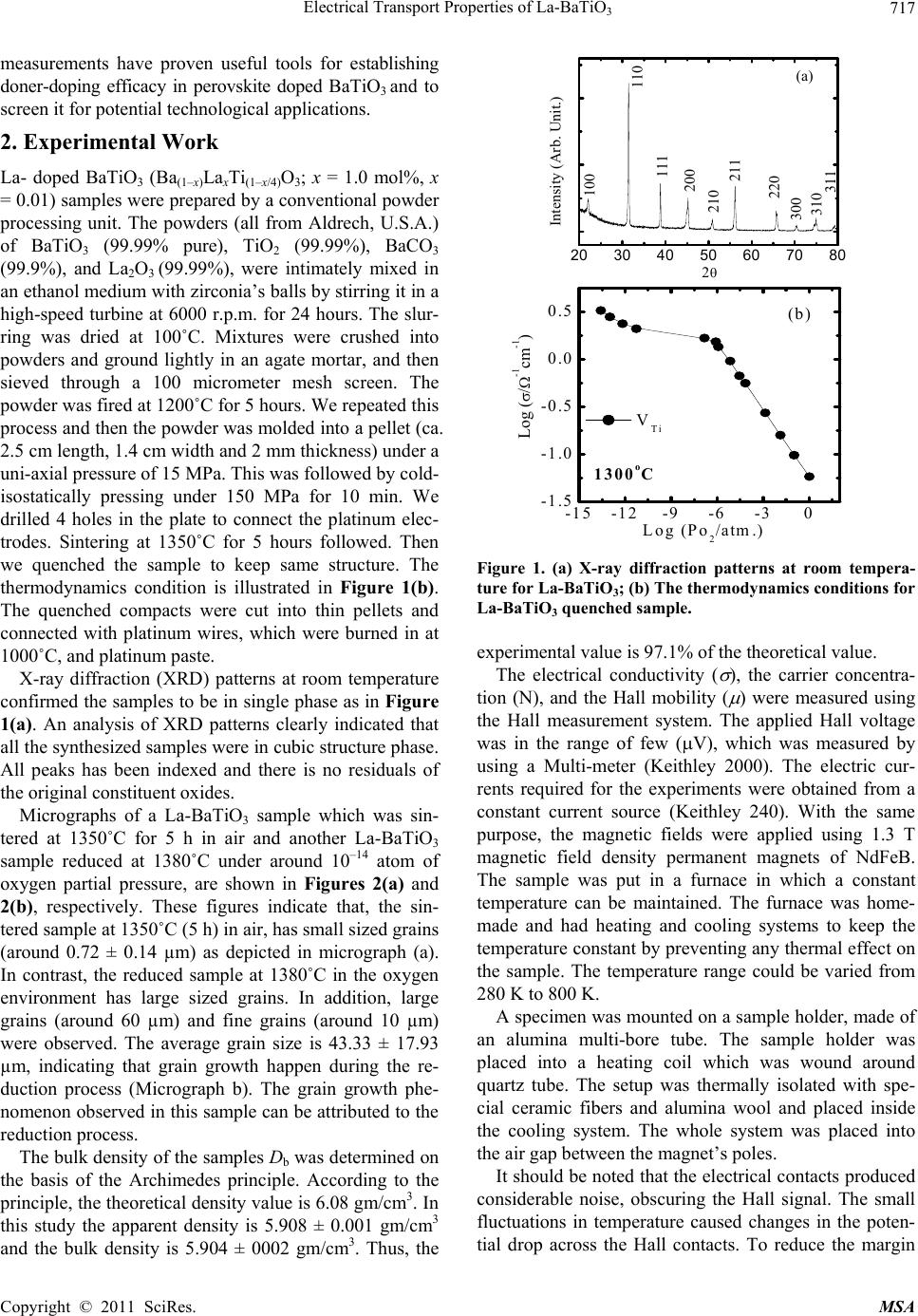
Electrical Transport Properties of La-BaTiO717
3
measurements have proven useful tools for establishing
doner-doping efficacy in perovskite doped BaTiO3 and to
screen it for potential technological applications.
2. Experimental Work
La- doped BaTiO3 (Ba(1–x)LaxTi(1–x/4)O3; x = 1.0 mol%, x
= 0.01) samples were prepared by a conventional powder
processing unit. The powders (all from Aldrech, U.S.A.)
of BaTiO3 (99.99% pure), TiO2 (99.99%), BaCO3
(99.9%), and La2O3 (99.99%), were intimately mixed in
an ethanol medium with zirconia’s balls by stirring it in a
high-speed turbine at 6000 r.p.m. for 24 hours. The slur-
ring was dried at 100˚C. Mixtures were crushed into
powders and ground lightly in an agate mortar, and then
sieved through a 100 micrometer mesh screen. The
powder was fired at 1200˚C for 5 hours. We repeated this
process and then the powder was molded into a pellet (ca.
2.5 cm length, 1.4 cm width and 2 mm thickness) under a
uni-axial pressure of 15 MPa. This was followed by cold-
isostatically pressing under 150 MPa for 10 min. We
drilled 4 holes in the plate to connect the platinum elec-
trodes. Sintering at 1350˚C for 5 hours followed. Then
we quenched the sample to keep same structure. The
thermodynamics condition is illustrated in Figure 1(b).
The quenched compacts were cut into thin pellets and
connected with platinum wires, which were burned in at
1000˚C, and platinum paste.
X-ray diffraction (XRD) patterns at room temperature
confirmed the samples to be in single phase as in Figure
1(a). An analysis of XRD patterns clearly indicated that
all the synthesized samples were in cubic structure phase.
All peaks has been indexed and there is no residuals of
the original constituent oxides.
Micrographs of a La-BaTiO3 sample which was sin-
tered at 1350˚C for 5 h in air and another La-BaTiO3
sample reduced at 1380˚C under around 10–14 atom of
oxygen partial pressure, are shown in Figures 2(a) and
2(b), respectively. These figures indicate that, the sin-
tered sample at 1350˚C (5 h) in air, has small sized grains
(around 0.72 ± 0.14 µm) as depicted in micrograph (a).
In contrast, the reduced sample at 1380˚C in the oxygen
environment has large sized grains. In addition, large
grains (around 60 µm) and fine grains (around 10 µm)
were observed. The average grain size is 43.33 ± 17.93
µm, indicating that grain growth happen during the re-
duction process (Micrograph b). The grain growth phe-
nomenon observed in this sample can be attributed to the
reduction process.
The bulk density of the samples Db was determined on
the basis of the Archimedes principle. According to the
principle, the theoretical density value is 6.08 gm/cm3. In
this study the apparent density is 5.908 ± 0.001 gm/cm3
and the bulk density is 5.904 ± 0002 gm/cm3. Thus, the
20 30 40 5060 70 80
(a)
311
100
210
211
310
300
220
200
111
2
Intensity (Arb. Unit.)
110
-15 -12-9-6-30
-1.5
-1.0
-0.5
0.0
0.5 (b)
VTi
1300oC
Log (/-1cm-1)
Log (Po2/atm.)
Figure 1. (a) X-ray diffraction patterns at room tempera-
ture for La-BaTiO3; (b) The thermodynamics conditions for
La-BaTiO3 quenched sample.
experimental value is 97.1% of the theoretical value.
The electrical conductivity (
), the carrier concentra-
tion (N), and the Hall mobility (
) were measured using
the Hall measurement system. The applied Hall voltage
was in the range of few (V), which was measured by
using a Multi-meter (Keithley 2000). The electric cur-
rents required for the experiments were obtained from a
constant current source (Keithley 240). With the same
purpose, the magnetic fields were applied using 1.3 T
magnetic field density permanent magnets of NdFeB.
The sample was put in a furnace in which a constant
temperature can be maintained. The furnace was home-
made and had heating and cooling systems to keep the
temperature constant by preventing any thermal effect on
the sample. The temperature range could be varied from
280 K to 800 K.
A specimen was mounted on a sample holder, made of
an alumina multi-bore tube. The sample holder was
placed into a heating coil which was wound around
quartz tube. The setup was thermally isolated with spe-
cial ceramic fibers and alumina wool and placed inside
the cooling system. The whole system was placed into
the air gap between the magnet’s poles.
It should be noted that the electrical contacts produced
considerable noise, obscuring the Hall signal. The small
fluctuations in temperature caused changes in the poten-
tial drop across the Hall contacts. To reduce the margin
Copyright © 2011 SciRes. MSA