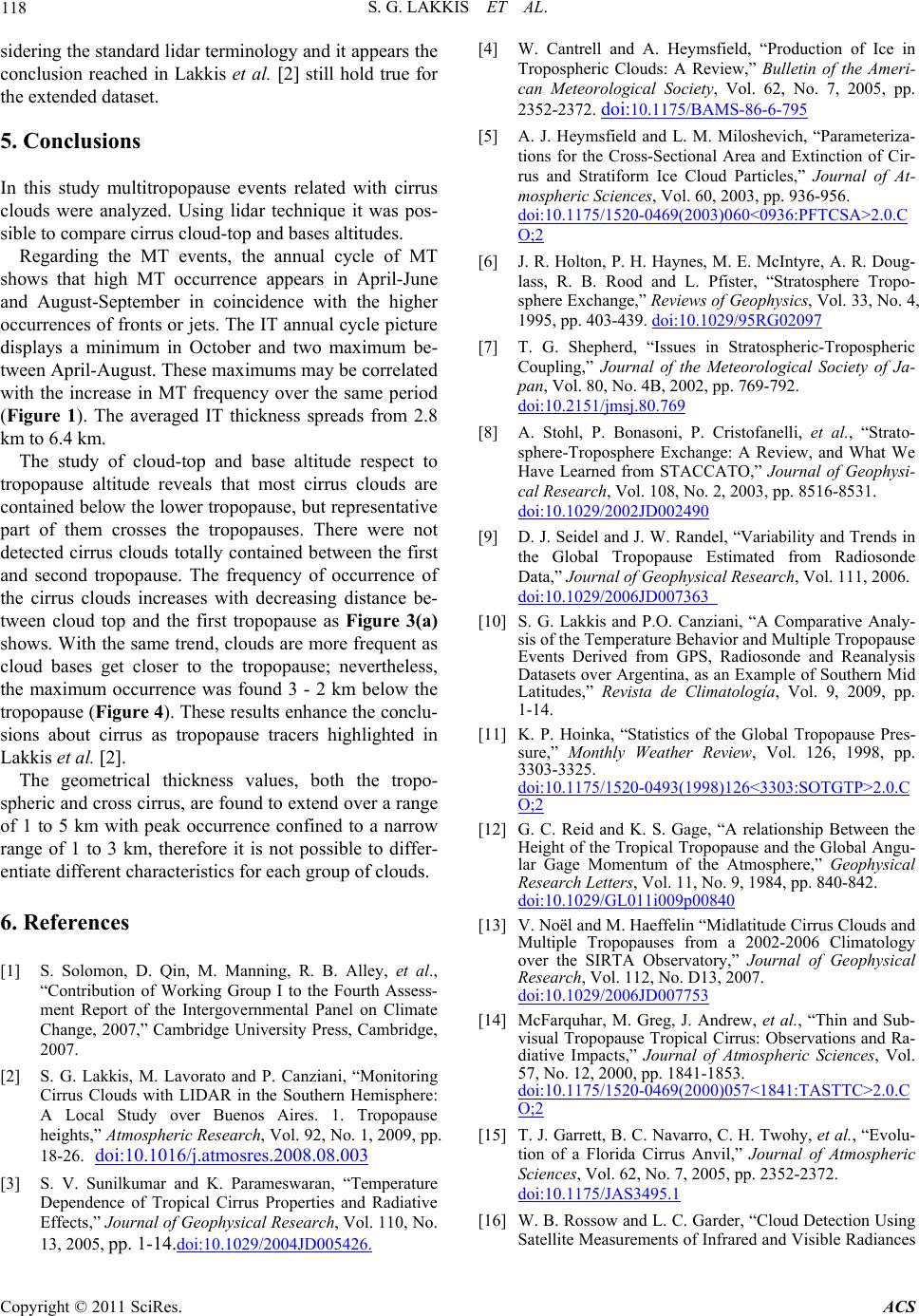
118 S. G. LAKKIS ET AL.
sidering the standard lidar terminology and it appears the
conclusion reached in Lakkis et al. 2 still hold true for
the extended dataset.
5. Conclusions
In this study multitropopause events related with cirrus
clouds were analyzed. Using lidar technique it was pos-
sible to compare cirrus cloud-top and bases altitudes.
Regarding the MT events, the annual cycle of MT
shows that high MT occurrence appears in April-June
and August-September in coincidence with the higher
occurrences of fronts or jets. The IT annual cycle picture
displays a minimum in October and two maximum be-
tween April-August. These maximums may be correlated
with the increase in MT frequency over the same period
(Figure 1). The averaged IT thickness spreads from 2.8
km to 6.4 km.
The study of cloud-top and base altitude respect to
tropopause altitude reveals that most cirrus clouds are
contained below the lower tropopause, but representative
part of them crosses the tropopauses. There were not
detected cirrus clouds totally contained between the first
and second tropopause. The frequency of occurrence of
the cirrus clouds increases with decreasing distance be-
tween cloud top and the first tropopause as Figure 3(a)
shows. With the same trend, clouds are more frequent as
cloud bases get closer to the tropopause; nevertheless,
the maximum occurrence was found 3 - 2 km below the
tropopause (Figure 4). These results enhance the conclu-
sions about cirrus as tropopause tracers highlighted in
Lakkis et al. 2.
The geometrical thickness values, both the tropo-
spheric and cross cirrus, are found to extend over a range
of 1 to 5 km with peak occurrence confined to a narrow
range of 1 to 3 km, therefore it is not possible to differ-
entiate different characteristics for each group of clouds.
6. References
[1] S. Solomon, D. Qin, M. Manning, R. B. Alley, et al.,
“Contribution of Working Group I to the Fourth Assess-
ment Report of the Intergovernmental Panel on Climate
Change, 2007,” Cambridge University Press, Cambridge,
2007.
[2] S. G. Lakkis, M. Lavorato and P. Canziani, “Monitoring
Cirrus Clouds with LIDAR in the Southern Hemisphere:
A Local Study over Buenos Aires. 1. Tropopause
heights,” Atmospheric Research, Vol. 92, No. 1, 2009, pp.
18-26. doi:10.1016/j.atmosres.2008.08.003
[3] S. V. Sunilkumar and K. Parameswaran, “Temperature
Dependence of Tropical Cirrus Properties and Radiative
Effects,” Journal of Geophysical Research, Vol. 110, No.
13, 2005, pp. 1-14.doi:10.1029/2004JD005426.
[4] W. Cantrell and A. Heymsfield, “Production of Ice in
Tropospheric Clouds: A Review,” Bulletin of the Ameri-
can Meteorological Society, Vol. 62, No. 7, 2005, pp.
2352-2372. doi:10.1175/BAMS-86-6-795
[5] A. J. Heymsfield and L. M. Miloshevich, “Parameteriza-
tions for the Cross-Sectional Area and Extinction of Cir-
rus and Stratiform Ice Cloud Particles,” Journal of At-
mospheric Sciences, Vol. 60, 2003, pp. 936-956.
doi:10.1175/1520-0469(2003)060<0936:PFTCSA>2.0.C
O;2
[6] J. R. Holton, P. H. Haynes, M. E. McIntyre, A. R. Doug-
lass, R. B. Rood and L. Pfister, “Stratosphere Tropo-
sphere Exchange,” Reviews of Geophysics, Vol. 33, No. 4,
1995, pp. 403-439. doi:10.1029/95RG02097
[7] T. G. Shepherd, “Issues in Stratospheric-Tropospheric
Coupling,” Journal of the Meteorological Society of Ja-
pan, Vol. 80, No. 4B, 2002, pp. 769-792.
doi:10.2151/jmsj.80.769
[8] A. Stohl, P. Bonasoni, P. Cristofanelli, et al., “Strato-
sphere-Troposphere Exchange: A Review, and What We
Have Learned from STACCATO,” Journal of Geophysi-
cal Research, Vol. 108, No. 2, 2003, pp. 8516-8531.
doi:10.1029/2002JD002490
[9] D. J. Seidel and J. W. Randel, “Variability and Trends in
the Global Tropopause Estimated from Radiosonde
Data,” Journal of Geophysical Research, Vol. 111, 2006.
doi:10.1029/2006JD007363
[10] S. G. Lakkis and P.O. Canziani, “A Comparative Analy-
sis of the Temperature Behavior and Multiple Tropopause
Events Derived from GPS, Radiosonde and Reanalysis
Datasets over Argentina, as an Example of Southern Mid
Latitudes,” Revista de Climatología, Vol. 9, 2009, pp.
1-14.
[11] K. P. Hoinka, “Statistics of the Global Tropopause Pres-
sure,” Monthly Weather Review, Vol. 126, 1998, pp.
3303-3325.
doi:10.1175/1520-0493(1998)126<3303:SOTGTP>2.0.C
O;2
[12] G. C. Reid and K. S. Gage, “A relationship Between the
Height of the Tropical Tropopause and the Global Angu-
lar Gage Momentum of the Atmosphere,” Geophysical
Research Letters, Vol. 11, No. 9, 1984, pp. 840-842.
doi:10.1029/GL011i009p00840
[13] V. Noël and M. Haeffelin “Midlatitude Cirrus Clouds and
Multiple Tropopauses from a 2002-2006 Climatology
over the SIRTA Observatory,” Journal of Geophysical
Research, Vol. 112, No. D13, 2007.
doi:10.1029/2006JD007753
[14] McFarquhar, M. Greg, J. Andrew, et al., “Thin and Sub-
visual Tropopause Tropical Cirrus: Observations and Ra-
diative Impacts,” Journal of Atmospheric Sciences, Vol.
57, No. 12, 2000, pp. 1841-1853.
doi:10.1175/1520-0469(2000)057<1841:TASTTC>2.0.C
O;2
[15] T. J. Garrett, B. C. Navarro, C. H. Twohy, et al., “Evolu-
tion of a Florida Cirrus Anvil,” Journal of Atmospheric
Sciences, Vol. 62, No. 7, 2005, pp. 2352-2372.
doi:10.1175/JAS3495.1
[16] W. B. Rossow and L. C. Garder, “Cloud Detection Using
Satellite Measurements of Infrared and Visible Radiances
Copyright © 2011 SciRes. ACS