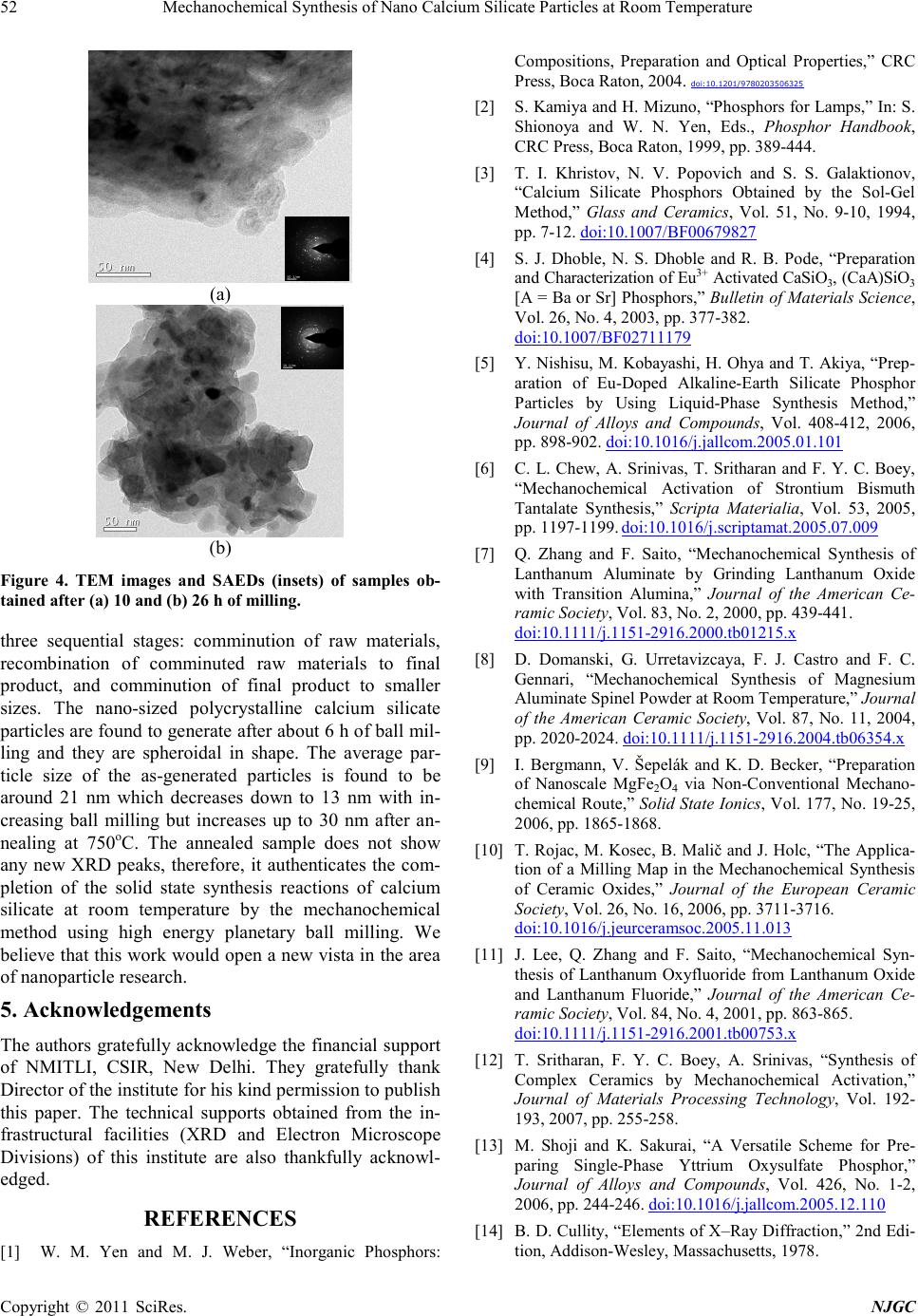
Mechan ochemical Synthesis of Nano Calcium Silicate P ar ticles at Room Temperature
Copyright © 2011 SciRes. NJGC
(a)
(b)
Figure 4. TEM images and SAEDs (insets) of samples ob-
tained after (a) 10 and (b) 26 h of milling .
three sequential stages: comminution of raw materials,
recombination of comminuted raw materials to final
product, and comminution of final product to smaller
sizes. The nano-sized polycrystalline calcium silicate
particles are found to generate after about 6 h of ball mil-
ling and they are spheroidal in shape. The average par-
ticle size of the as-generated particles is found to be
around 21 nm which decreases down to 13 nm with in-
creasing ball milling but increases up to 30 nm after an-
nealing at 750oC. The annealed sample does not show
any new XRD peaks, therefore, it authenticates the com-
pletion of the solid state synthesis reactions of calcium
silicate at room temperature by the mechanochemical
method using high energy planetary ball milling. We
beli eve tha t thi s work would op en a ne w vista in the area
of nanopar tic le research.
5. Acknowledg ements
The authors grateful ly ack nowledge the fina ncial suppo rt
of NMITLI, CSIR, New Delhi. They gratefully thank
Director o f the institute for his kind per missio n to p ublish
this paper. The technical supports obtained from the in-
frastructural facilities (XRD and Electron Microscope
Divisions) of this institute are also thankfully acknowl-
edged.
REFERENCES
[1] W. M. Yen and M. J. Weber, “Inorganic Phosphors:
Compositions, Preparation and Optical Properties,” CRC
Press, Boca Raton, 2004. doi:10.1201/9780203506325
[2] S. Kamiya and H. Mizuno, “Phosphors for Lamps,” I n: S.
Shionoya and W. N. Yen, Eds., Phosphor Handbook,
CRC Press, Boca Raton, 1999, pp. 389-444.
[3] T. I. Khristov, N. V. Popovich and S. S. Galaktionov,
“Calcium Silicate Phosphors Obtained by the Sol-Gel
Method,” Glass and Ceramics, Vol. 51, No. 9-10, 1994,
pp. 7-12. doi:10.1007/BF00679827
[4] S. J. Dhoble, N. S. Dhoble and R. B. Pode, “Preparation
and Ch aracterizat ion of Eu 3+ Activat ed CaSiO3, (C aA)SiO3
[A = Ba or Sr] Phosphors,” Bulletin of Materials Scien ce,
Vol. 26, N o. 4, 20 03, pp. 37 7-382.
doi:10.1007/BF02711179
[5] Y. Nish isu, M. Kobayashi, H. Ohya and T. Akiya, “Prep-
aration of Eu-Doped Alkaline-Earth Silicate Phosphor
Particles by Using Liquid-Phase Synthesis Method,”
Journal of Alloys and Compounds, Vol. 408-412, 2006,
pp. 898-902. doi:10.1016/j.jallcom.2005.01.101
[6] C. L. Chew, A. Srinivas, T. Sritharan and F. Y. C. Boey,
“Mechanochemical Activation of Strontium Bismuth
Tantalate Synthesis,” Scripta Materialia, Vol. 53, 2005,
pp. 1197 -1199. doi:10.1016/j.scriptamat.2005.07.009
[7] Q. Zhang and F. Saito, “Mechanochemical Synthesis of
Lanthanum Aluminate by Grinding Lanthanum Oxide
with Transition Alumina,” Journal of the American Ce-
ramic Society, Vol. 83, No. 2, 2000, pp . 439-441.
doi:10.1111/j.1151-2916.2000.tb01215.x
[8] D. Domanski, G. Urretavizcaya, F. J. Castro and F. C.
Gennari, “Mechanochemical Synthesis of Magnesium
Aluminate Spinel Powder at Room Temperature,” Journal
of the American Ceramic Society, Vol. 87, No. 11, 2004,
pp. 2020 -2024. doi:10.1111/j.1151-2916.2004.tb06354.x
[9] I. Bergmann, V. Šepelák and K. D. Becker, “Preparation
of Nanoscale MgFe2O4 via Non-Conventional Mechano-
chemical Ro ute,” Solid State Ionics, Vol. 177, No. 19-25,
2006, pp . 1865-1868.
[10] T. Rojac, M. Kosec, B. Malič and J. Holc, “The Applica-
tion of a Milling Map in the Mechanochemical Synthesis
of Ceramic Oxides,” Journal of the European Ceramic
Soci ety, Vol. 26, No. 16, 2006, pp. 3711-3716.
doi:10.1016/j.jeurceramsoc.2005.11.013
[11] J. Lee, Q. Zhang and F. Saito, “Mechanochemical Syn-
thesis of Lanthanum Oxyfluoride from Lanthanum Oxide
and Lanthanum Fluoride,” Journal of the American Ce-
ramic Society, Vol . 84, No. 4, 20 01, pp . 863-865.
doi:10.1111/j.1151-2916.2001.tb00753.x
[12] T. Sritharan, F. Y. C. Boey, A. Srinivas, “Synthesis of
Complex Ceramics by Mechanochemical Activation,”
Journal of Materials Processing Technology, Vol. 192-
193, 2007, pp. 255-258.
[13] M. Shoji and K. Sakurai, “A Versatile Scheme for Pre-
paring Single-Phase Yttrium Oxysulfate Phosphor,”
Journal of Alloys and Compounds, Vol. 426, No. 1-2,
2006, pp . 244-246. doi:10.1016/j.jallcom.2005.12.110
[14] B. D. Cullity, “Elements of X–Ray Diffraction,” 2nd Edi-
tion, Addison-Wesley, Massachusetts, 1978.