 Materials Sciences and Applications, 2015, 6, 953-962 Published Online November 2015 in SciRes. http://www.scirp.org/journal/msa http://dx.doi.org/10.4236/msa.2015.611096 How to cite this paper: Afrough, M., Pandya, T.S., Daryadel, S.S. and Mantena, P.R. (2015) Dynamic Response of Pultruded Glass-Graphite/Epoxy Hybrid Composites Subjected to Transverse High Strain-Rate Compression Loading. Materials Sciences and Applications, 6, 953-962. http://dx.doi.org/10.4236/msa.2015.611096 Dynamic Response of Pultruded Glass-Graphite/Epoxy Hybrid Composites Subjected to Transverse High Strain-Rate Compression Loading Mohammad Afrough*, Tejas S. Pandya, Seyed Soheil Daryadel, Prabhakar Raju Mantena Composite Structures and Nano-Engineering Research, Department of Mechanical Engineering, University of Mississippi, Oxford, USA Received 18 September 2015; accepted 14 November 2015; published 17 Nove mber 2015 Copyright © 2015 by authors and Scientific Research Publishing Inc. This work is licensed under the Creative Commons Attribution International License (CC BY). http://creativecommons.org/licenses/by/4.0/ Abstract In a previous study, the energy absorption and dynamic response of different combinations of cy- lindrical fiber-reinforced pultruded hybrid composite samples made of unidirectional glass and graphite fiber/epoxy, were investigated under longitudinal compression loading. It was found that placing glass fibers in the inner core of composites resulted in a higher ultimate compressive strength and specific energy absorption. In this study, the dynamic responses of pultruded glass- graphite/epoxy hybrid specimens with rectangular cross-section subjected to transverse com- pression loading are reported. Crack initiation and propagation was monitored using a high-speed video camera, and the effects of hybridization were analyzed. It was found that the location of glass or graphite fibers inside the pultruded composites has no significant effect on the ultimate compressive strength under such transverse compression loading. The energy absorption in all the hybrid specimens was almost identical. Graphite/epoxy composite showed higher specific energy absorption due to its lower density, and glass/epoxy composite had the lowest specific energy absorption. Keywords Pultruded Composites, High Strain-Rate Compression Loading, SHPB, Energy Absorption, Transverse Loadings Corresponding author.
 M. Afrough et al. 1. Introduction Composites are utilized because they have desired properties which cannot be attained by other types of consti- tuent materials. Fibrous composites, including reinforcing fibers embedded in a matrix material, are commonly used in different applications. Fiber-reinforced composite materials present different features in terms of stiff- ness, specific strength, deformation etc. Their usage encompasses a wide range of applications in automotive, aerospace and marine. The two most common reinforcing fibers are glass and graphite. Glass fibers have high tensile strength and lo w tens ile mod ulus. O n the othe r hand, graphit e fiber s possess very hi gh tensi le modulus, lo w wei ght and low impact resistance. A number of investigations have been carried out on pultruded composites [1]-[13]. The ir re- sults illustrate that hybridization with different percentages of glass and graphite within the same epoxy matrix has a significant effect on the mechanical properties. In many applications, composite materials are subjected to dynamic loading, which is produced by vibration or wave propagation [14]. Therefore, it is necessary to investigate the strain rate sensitivity, failure modes and other be havio rs und er dyna mic loa ding. Sever al s tudie s ha ve been performed on strain rate sensitivity [15]-[17]. Ochola et a l. [18] tested a glass fiber reinforced polymer at different strain rates. The results show that the value of mean ultimate compressive stress for this composite increased by 20.9% as the strain rate is increased from 10−3 to 450 s−1. Vari ous set -ups li ke d ro p -weight and Sp lit H o pki nson P re ssur e B a r (S HP B ) have b ee n u se d [19] [20]. Furthermore, direction of loading and fiber orientation play an important role in dynamic behavior of compo- site materials. Yokoyama et al. [21] investigated cubic specimens of carbon/epoxy laminates behavior in all three principal material directions under high strain rate compression test and discussed the failure mechanism of co mposit es. T hey sho w that b y increa sing strain rate, while the compression is alo ng the fiber d irection, ulti- mate strength increases, and energy absorbed up to failure strain decreases. In a previous investigation by the authors [22], the energy absorption and dynamic response of different combinations of glass and graphite pul- truded composites under longitudinal compression loading was studied. It was found that locating glass fibers in the inner core raises the ultimate compressive strength and results in better dynamic behavior of the composite. Since there are many applications where loads are applied in transverse direction, optimization of pultruded composite materials from thi s point of view is necessitated . The purpose of this study is to analyze the dynamic behavior and energy absorption of pultruded glass-graph- ite/epoxy hybrid composites by applying transverse compression load at high strain-rate. A modified SHPB test system has been used for producing dynamic load. Additionally, a high-speed camera was deployed to record the event s and monito r crack i nitiati on, propaga tion a nd fai lure of samples during the tests. 2. Specimen Details Samples for SHPB test were cut from long rectangular cross-section composite beams manufactured by the pul- trusion process (Pulstar 804 machine). Glass and graphite fibers were used for reinforcement, while volume fraction of epoxy was maintained at 40%. The graphite fibers were AS4W-12K (Hercules), the glass fibers were E-Glass (PPG 2001, #12), and the epoxy was EPON 862/W/537 (Shell Chemical Company, USA). All the SHPB test samples were cut precisely in 6.6 mm × 6.6 mm section from 3.3 mm thick long rectangular beams (Figure 1). The six fiber combinations with layup sequence for hybrid composite test samples are shown in Table 1. Reference [23] describes more details of the manufacturing process for these pultruded beam samples. The measured bulk densities of the specimens are shown in Table 2. 3. Experimental Technique The high strain-rate testing was carried out using a modified SHPB located at the Blast and Impact Dynamics Laborator y, University o f Mississippi. A schematic of SHP B set-up is s ho wn in Figure 2. It co nsists of a str iker bar, a strain gaged incide nt/input bar and a transmitter/output bar. The bars are made of maraging steel. Speci- mens are sandwiched between the incident and transmission bars. A copper pulse shaper was applied to attain a triangular i ncident pulse. Petroleum jelly was used to place specimen between the incident and transmitter bars, and also to avoid friction and shear effects on the samples during testing.
 M. Afrough et al. Figure 1 . Dimensions of test samples cut from rectangular cross-secti on pultru de d be a m s . Figure 2 . Schematic of a Split Hopkinson Pressure bar [20]. Table 1. Fiber combinations with layup sequence for hybrid composites [23]. Specimen ID Fiber Volume Fraction (%) Resin S ystem (%) Cross Section C F MIX0 1 60 0 40 C F MIX0 2 0 60 40 C F MIX0 4 30 15 + 15 40 C F MIX0 5 20 + 20 20 40 C F MIX0 6 15 + 15 30 40 Table 2. Measured average bulk densities of the pultruded hybrid specimens. Density (kg/m3) CF M IX0 1 C FMIX0 2 C F MIX03 1569 1924 1807 CF M IX0 4 C FMIX0 5 C F MIX06 1809 1749 1784 A hi gh-speed video camera (HyperVision HPV-2, Shimadzu Corporation, Japan) was employed to record the event s and mo nitor the c rack ini tiatio n, propagation and failure of samples during the SHPB compression load- ing tests. One hundred and two frames of each event were recorded at a frame rate of 500,000 fps. Two 1000W strobes were used to provide the required lighting. 4. Results and Discussion Six combinations of pultruded glass-graphite/epoxy hybrid samples were tested under transverse compression
 M. Afrough et al. loading. Figure 3 shows typical str ess wave pulse s includi ng incident, reflected and tr ansmitted pulses. For va- lidating the dynamic equilibrium condition, a test specimen must be in force equilibrium [22]. The stresses in both interfaces of each specimen were calculated to ensure that equilibrium was attained (Figure 4). Five samples were tested for each combination at an average strain rate of 800 s−1. Figure 5 shows typical stress-strain behavior for all the combinations. All curves are plotted until shattering point of each specimen, with hi gh-speed photography utilized for capturing this moment. They are essentially coincident with each other until ultimate compressive strength with all of them possessing equal initial stiffness. This synchronization ap- pears to be from matrix dominant response for these pultruded composites, with compression load applied in the transverse direction. Figure 6 illustrates the average ultimate compressive strength for six specimens of each combination. CFMIX01 with 60% graphite possess the highest ultimate compressive strength and CFMIX02 with 60% glass has the lowest. The compressive strength of hybrid with 40% graphite in the outer layer and 20% glass in the Figure 3. Typical incident, reflected and transmitted pulses from SHPB test of a pultruded hybrid composite sample. Figure 4 . V alidation of stress equilibrium for tested pultruded hybrid composite sample.
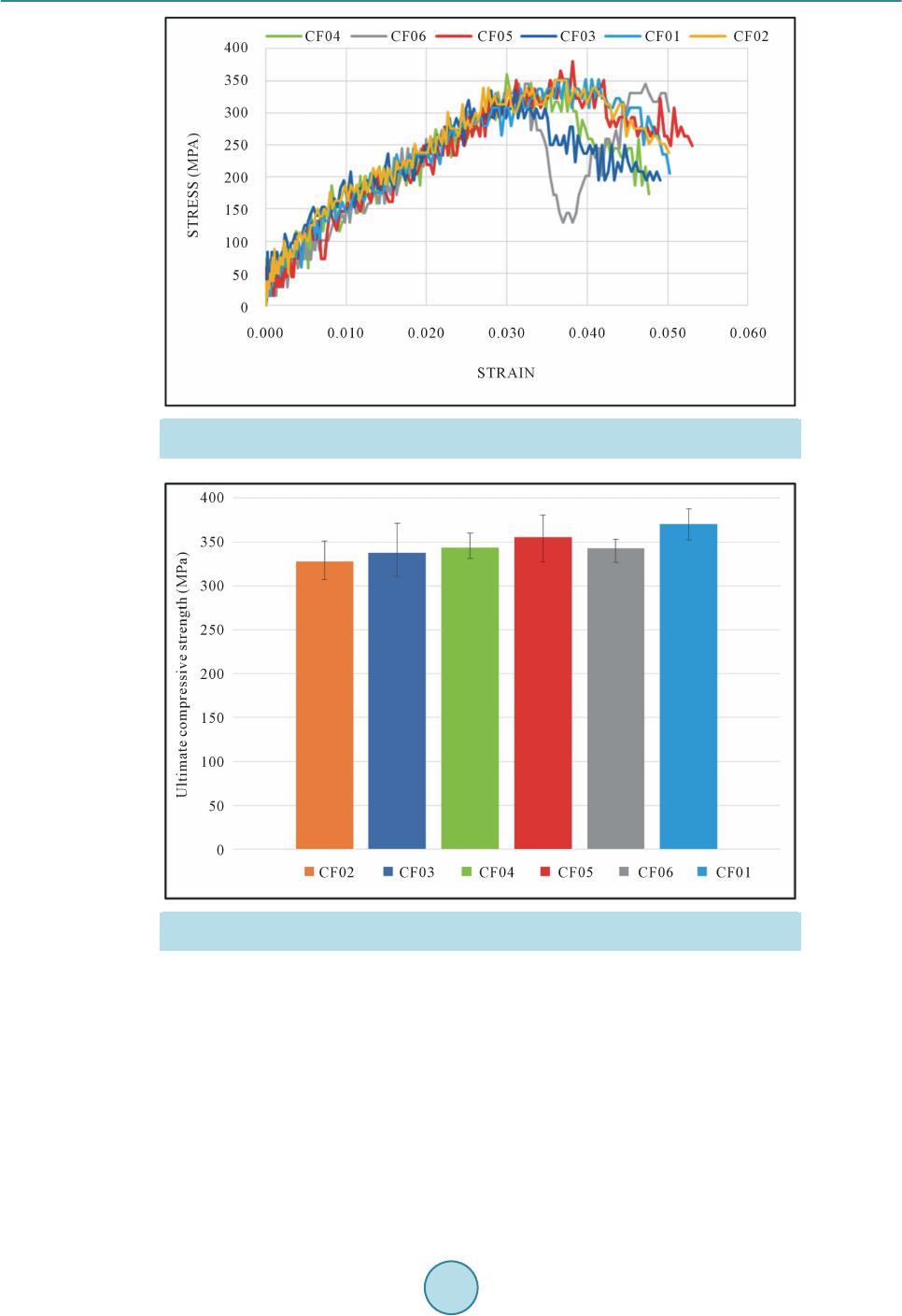 M. Afrough et al. Figure 5 . Typical stress-strain curve for all hybrids tested by SHPB transverse compression loading. Figure 6. Ultimate compressive strength (MPa) for all hybrids tested by SHPB transverse compression loading. core, CFMIX05, is slightly nearer to graphite/epoxy. The small difference between the highest and lowest ulti- mate compressive strength demonstrates that using different portions of glass and graphite fibers does not dra- matically change the compressive strength under transverse compression dynamic loading. This small difference results from the stronger bond between graphite fiber and epoxy matrix compared to the bond between glass fi- ber and epoxy matrix, perhaps due to the sizing of graphite fibers for improving adhesion during pultrusion manufacturing. As can be seen in Figure 7, graphite fibers (Figure 7(a)), have mostly disintegrated after the compression event while gla ss fibers (Figure 7(b)), remained almost intact. As can be seen in Figure 5, all the specimens absorbed almost equivalent amounts of energy (integration of area under stress-strain curve) during the dynamic compression tests, with specimens having greater volume fraction of graphite fibers absorbing marginally more energy. CF06 samples showed a distinct drop towards the
 M. Afrough et al. (a) (b) Figure 7 . Micrograph vi ew of speci mens after trans ver se compression event (500×). (a) Graphite fib e rs ; and (b) Glass fibe rs . Figure 8 . Sp ecific energy absorption for all hybrid specimens tested by SHPB under transverse compression loading. end of the compressive event, perhaps due to the larger portion of glass fibers located in the central region. However, the specific energy absorption (energy absorbed per unit mass) for each specimen is distinct due to their different densi ties (Figure 8). As shown i n Table 2, specimens with more graphite fibers have lower den- sity, which resulted in higher specific energy absorption. Specimens with 60% graphite (CFMIX01) and 40% graphite (CFMIX05) show the highest specific energy absorption; while 60% glass (CFMIX02) exhibits the lowest specific energy absorption. As men tioned, a high -speed video camera was deployed to monitor the crack initiation, prop agation and fail- ure of samples during the SHPB compression loading tests. For these samples, the event (from time zero to crack initiation of specimen) takes 55 μs -75 μs. Four stages of specimen CFMIX05 deformation are shown in Figure 9. The stress versus time histor y of this specimen is dep icted in Figure 10. Three red dot s show starting point, ultimate strength point and crack initiation point. The maximum load (ultimate strength) occurs at 51 μs and crack initiates at 66 μs. The image at 79 μs shows that the fiber glass in the specimen core detaches from epoxy matrix. The results indicate that the entire sample has not been completely damaged at the peak stress. It should be noted that the compression load is applied transverse to the fiber direction, i.e., load is perpendicular to the fiber orientation for each of the tested hybrid composites.
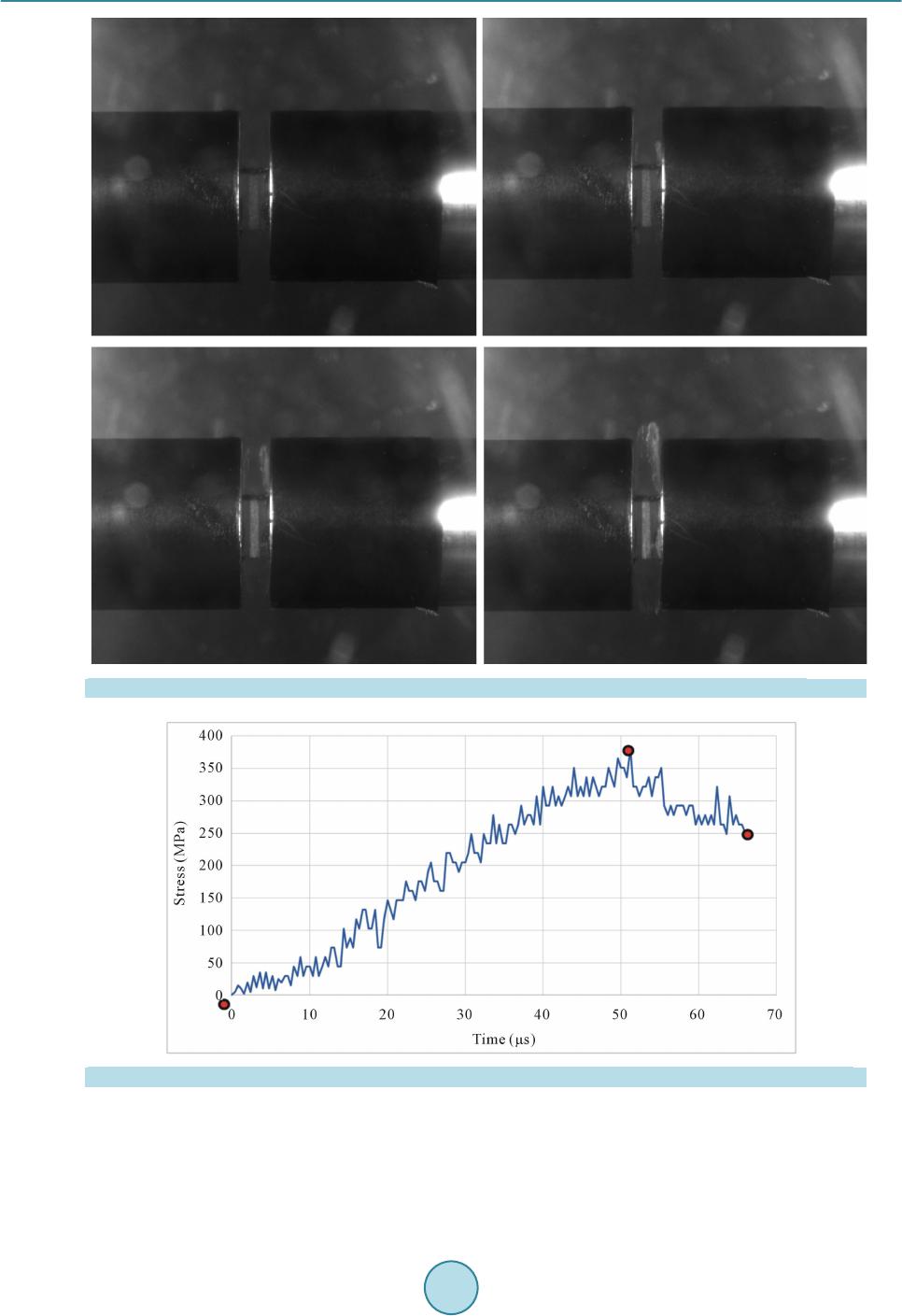 M. Afrough et al. Figure 9 . Four stages of specimen CFMIX05 deformation under transverse compression at different times. Figure 10. Stress versus time history of CFMIX05 under transverse compression loading. Three red dots show st. A comparison between this study and previous investigation conducted by the authors on longitudinal com- pre ssive load ing [22] shows dramatic difference in the dynamic response of graphite-glass/epoxy pultruded hy- brids under transverse and longitudinal loading conditions. Figure 11 and Figure 12 show the ultimate com- pressive strength and average specific energy of cylindrical hybrid combinations where GL60, GR60, GL30 and
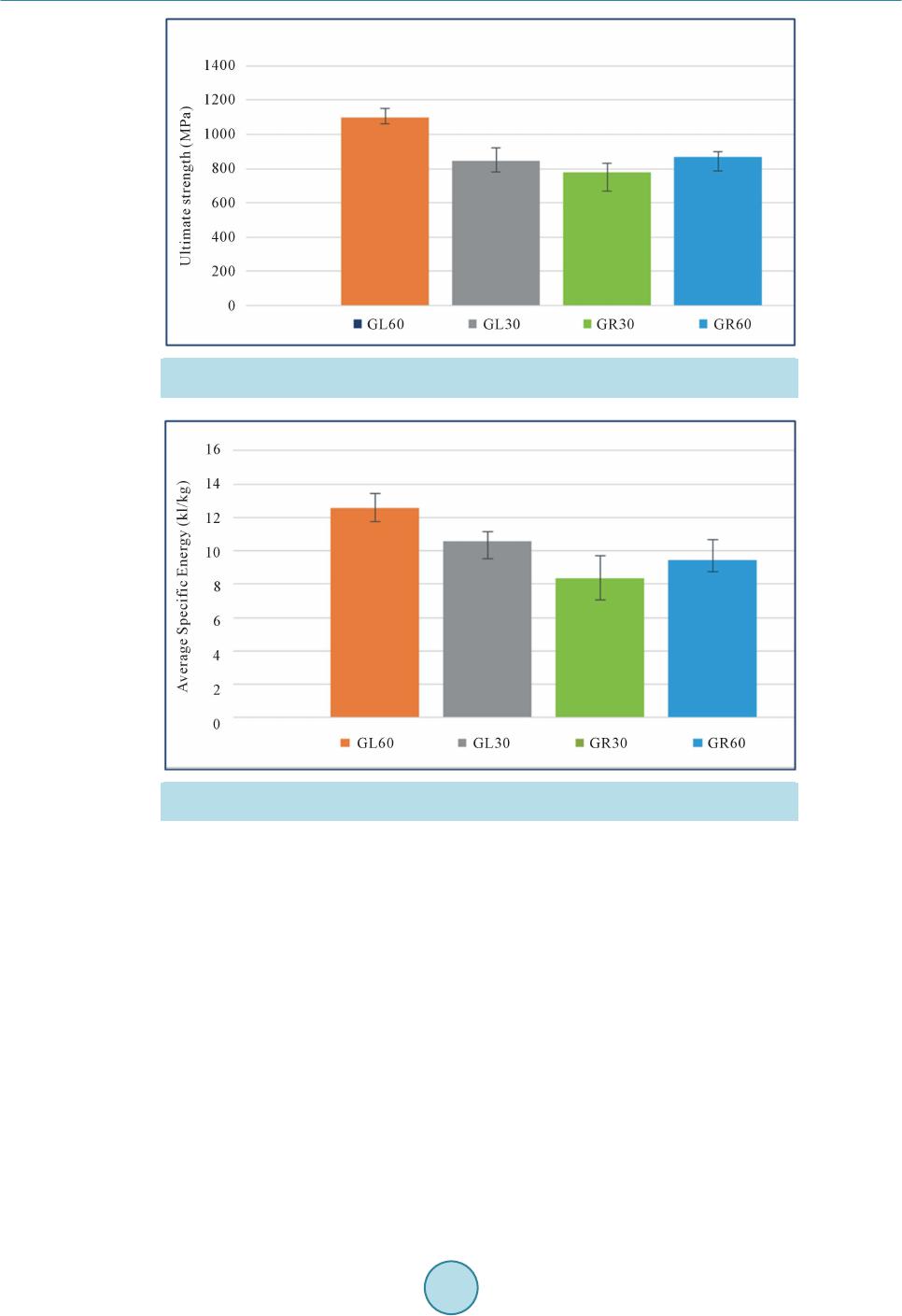 M. Afrough et al. Figure 11. Ultimate compressive strength for different combinations of pultruded hybrids under longitudinal compression loading [22]. Figure 12. Ave r age specific energy absorption for different combinations of pultruded hy- brids un de r lo ng it u di na l c ompre s sion l oa ding [22]. GR30 have respectively similar hybrid combinations of CF02, CF01, CF06 and CF04 used in this study. It is apparent that under transverse compression dynamic loading, ultimate compressive strength of graphite-glass/ epoxy pultruded hybrids is about one-third o f tha t und er lo ngi tud inal c ompr e ssion d yna mi c lo ading. On t he ot h- er hand, specific energy absorption under longitudinal compression loading shows an opposite trend to the re- sponse under transverse compression loading. 5. Conclusion In this i nvestigation, six combinations of pultruded glas s-graphite/epoxy hybrids have been experimentally stu- died und er t rans ver se hi gh s tr ain -rate compression loading, using a modified SHPB. It was observed that failure of specimens loaded along transverse direction was do minated by matrix failure. It was also observed that 60% grap hite (CF MIX01 ) has t he highe st ultimate co mpressive strength. The ultimate compressive strength was also marginally greater with higher percentage of graphite. This marginal difference results from the stronger bond between graphite fiber and epoxy compared to that between glass fiber and epoxy, perhaps due to the sizing of graphite fibers for better adhesion. Location of glass or graphite fibers inside the pultruded composites had no significant effect on the ultimate compressive strength under transverse compression dynamic loading. While all
 M. Afrough et al. specimens absorbed almost equivalent amount of energy, the graphite/epoxy samples demonstrated significantly higher specific energy absorption compared to the other combinations. This was due to its lo wer density, while glass/epox y sho wed the lowest specific energy absorption. Moreover, comparison between this study and a pre- vious investigation conducted by the authors showed significant differences in the response under longitudinal and trans verse dynamic l oad i ng. Acknowledgements The authors wish to acknowledge funding received from US Army Research Office-DURIP Grant # W911NF- 13-1-0248 for the high-speed digital cameras used in this research. The authors would also like to thank Mr. P. Matthew Lowe, for his help w ith sample prep a ration. References [1] Swaminathan, R. and Raju Mantena, P. (2003) Axial Loading and Buckling Response Characteristics of Pultruded Hy- brid Glass-Graphite/Epoxy Composites. Journal of Reinforced Plastics and Composites, 22. [2] Nori , C.V., R aju Man tena, P. and McCarty, T.A. (1996) Experimental and Finite Element Analysis of Pultruded Glass- Graphite/Epoxy Hybrids in the Axial and Flexural Modes of Vibration. Journal of Composite Materials, 30. http://dx.doi.org/10.1177/002199839603001803 [3] Shokrieh, M.M. and Omid i, M.J. (2011) Investigating the Transverse Behavior o f Glass-Epoxy Composites under In- termediate Strain Rates. Journal of Com pos i te St r uc tures, 93, 690-696. http://dx.doi.org/10.1016/j.compstruct.2010.08.010 [4] Hosu r , M.V., Adya , M., Jeelani, S., Vaidya, U.K. and Dutta, P.K. (2004) Experimental Studies on the High Strain Rate Compression Response of Woven Graphite/Epoxy Composites at Room and Elevated Temperatures. Journal of Rein- forced Plastics and Composites, 23, 491-514. http://dx.doi.org/10.1177/0731684404032019 [5] Vaughan, J.G., Roux, J.A. and Mantena, P.R. (1992) Characterization of Mechanical and Thermal Properties of Ad- vanced Composite Pultrusion. Proceedings of the 1992 NSF Design and Manufacturing Systems Conference, 1141- 1145. [6] Mantena, P.R., Vaughan, J.G., Donti, R.P. and Kowsika, M.V. (1992) Influence of Process Variables on the Dynamic Characteristics of Pultruded Graphite-Epoxy Composites. Vibro -Aco ustic Characterization of Materials and Structures, ASME, 14, 147-154. [7] Almagab leh, A., Gupta, S., Raju Mant ena, P. an d Al-Ost az, A. (2008) Dynamic Mechanical Analysis of Graphite Pla- telets an d Nanoclay Rein forced V inyl Ester, and MWCNT Reinforced Nylon 6.6 Nanocomposites. Proceedings of the 2008 SAMPE Fall Technical Conference, (Paper # 034 on CD) ROM, Memphis, 8-11 September 2008. [8] Jadhav, P., Raju Mantena, P. and Gibson, R.F. (2006) Energy Absorption and Impact Damage Evaluation of Grid- Stiffened Composite Panels under Transverse Loading. Compos i te s E ngineer i ng, Part B , 37, 191-199. http://dx.doi.org/10.1016/j.compositesb.2005.05.017 [9] Kowsika, M., Raju Manten a, P. and Balasu bramniam, K. (2002) Energy Absorption and Dissipation Characteristics of Pultruded Glass-Graphite/Epoxy Hybrid Composite Beams. Journal of Thermoplastic Composite Materials, 15. http://dx.doi.org/10.1177/0892705702015003453 [10] Raju Mantena, P., Mann, R. and Nori, C.V. (2003) Low Velocity Impact Response and Dynamic Characteristics of Gla s s -Resin Composites. Journal of Reinforced Plastics and Composites, 20, 513-534. [11] Barpanda, D. and Mantena, P.R. (1998 ) Effects of H ybridizat ion on the Cr eep and Stress Relaxati on Ch aracterist ics of Pultruded Composites. Journal of Reinforced Plastics and Composites, 17, 234-249. [12] Barpanda, D. and Mantena, P.R. (1996) Dynamic Mechanical Analysis of Pultruded Glass-Graphite/Epoxy Hybrid Composi tes at Elevated Temperatures. Journal of Reinforced Plastics and Composites, 15, 497-532. [13] Ku ma r, S. and Mantena, P.R. (1996) Dynamic and Static Torsional Characterization of Pultruded Hybrid Cylindrical Composite Rods. Journal of Composite Materials, 30, 918-932. http://dx.doi.org/10.1177/002199839603000804 [14] Gibson, R.F. (2007) Principles of Composite Material Mechanics. 2nd Edition, CR C Press, Boca Rat on. [15] Sierakowski, R.L. (1997) Strain Rate Effects in Composites. Applied Mecha nics Reviews , 50, 741-761. [16] Hamouda, A.M.S. and Hashmi, M.S.J. (1998) Testing of Composite Materials at High Rates of Strain, Advances and Challenges. Journal of Mate r i a l s Pr oc e s s i ng Tec h nology, 77, 327-336. http://dx.doi.org/10.1016/S0924-0136(97)00436-6 [17] Al-Hassani, S.T.S. and Kaddour, A.S. (1998) Strain Rate Effects on GRP, KRP and CFRP Composite Laminates. Key Engineering Materials, 141-143, 427-452. http://dx.doi.org/10.4028/www.scientific.net/KEM.141-143.427
 M. Afrough et al. [18] Ochola, R.O. , Marcus, K., Nu ri ck, G.N . and Franz, T. (2004) Mechanical Behaviour of Glass and Carbon Fiber Rein- forced Composites at Varying Str ain Rates. Journal of Composite Structures, 63, 455-467. http://dx.doi.org/10.1016/S0263-8223(03)00194-6 [19] Hangai, Y., Kubota, N., Utsunomiya, T., Kawashi ma, H., Kuwazuru, O. and Yoshikawa, N. (2015) Drop Weight Im- pact Behavior of Functionally Graded Aluminum Foam Consisting of A1050 and A6061 Aluminum Alloys. Journal of Materials Science & Engineering A, 639, 597-603. http://dx.doi.org/10.1016/j.msea.2015.05.007 [20] Gama, B.A., Lopatnikov, S.L. and Gillespie Jr., J.W. (2004) Hopkinson Bar Experimental Technique, a Critical Re- view. Applied Mecha nics Reviews , 57, 223-250. [21] Yokoyama, T., Nakai, K. and Odamura, T. (2007) High Strain-Rate Compressive Characteristics of a Unidirectional Carbon/Epoxy Composite: Effect of Loading Directions. In: Gdoutos, E.E., Ed., Experimental Analysis of Nano and Engineering Materials and Structures, Springer, Dordrecht, 681 -682. http://dx.doi.org/10.1007/978-1-4020-6239-1_338 [22] Daryadel, S.S., Ray, C., Pa n dya , T. and Mantena, P.R. (2015) Energy Absorption of Pultruded Hybrid Glass/Graphite Epoxy Composites under High Strain-Rate SHPB Compression Loading. Materials Sciences and Applications, 6, 511- 518. http://dx.doi.org/10.4236/msa.2015.66054 [23] Mantena, P.R., Vangipuram, R. and Vaughan, J.G. (1994) Dynamic Flexural Properties of Pultruded Glass/Graphite Hybrid Composites. Pr oceedi ngs of t he 39th International SAMPE Symposium, Anaheim, 11-14 Apri l 1994, 174-182.
|