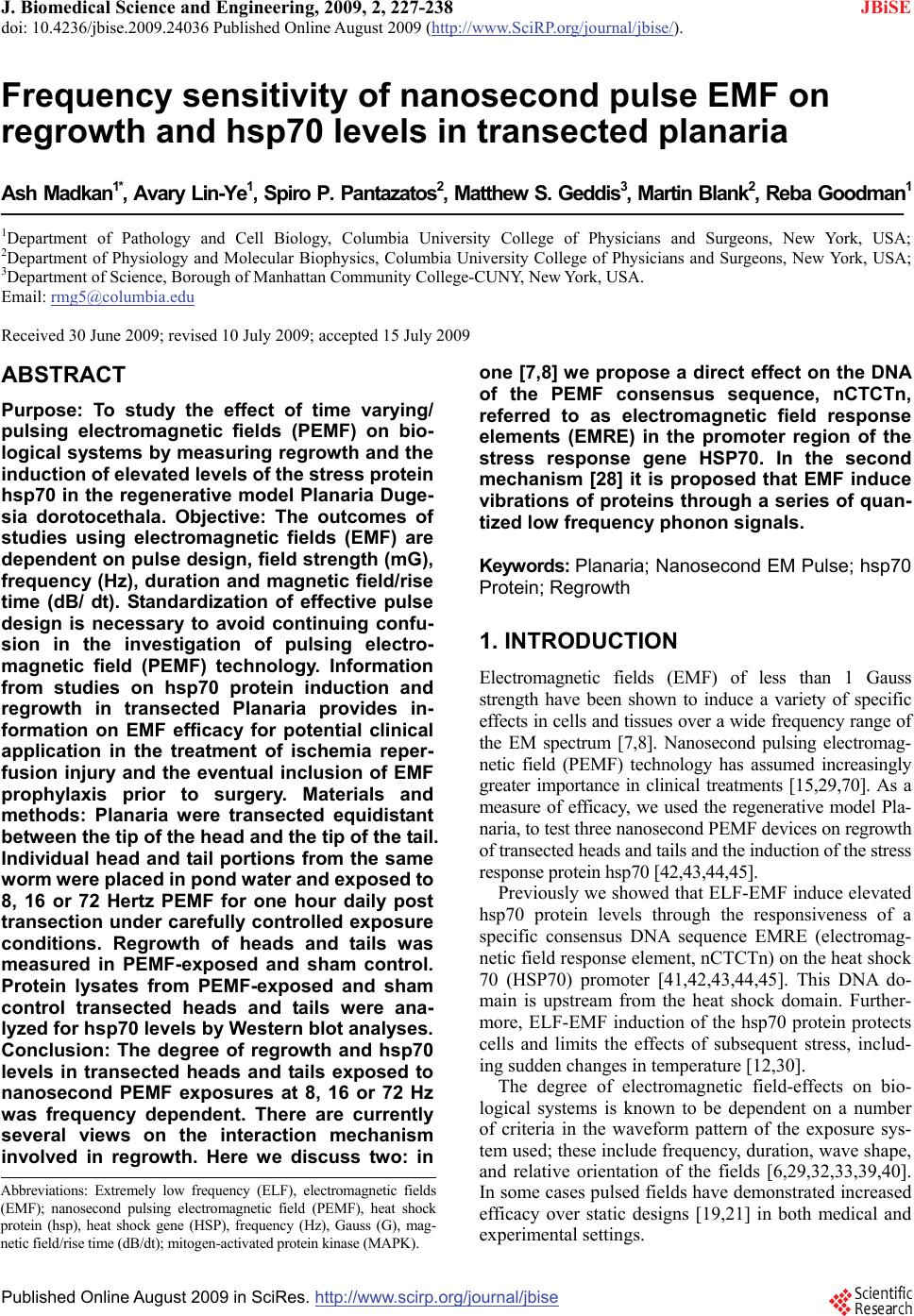 J. Biomedical Science and Engineering, 2009, 2, 227-238 doi: 10.4236/jbise.2009.24036 Published Online August 2009 (http://www.SciRP.org/journal/jbise/ JBiSE ). Published Online August 2009 in SciRes. http://www.scirp.org/journal/jbise Frequency sensitivity of nanosecond pulse EMF on regrowth and hsp70 levels in transected planaria Ash Madkan1*, Avary Lin-Ye1, Spiro P. Pantazatos2, Matthew S. Geddis3, Martin Blank2, Reba Goodman1 1Department of Pathology and Cell Biology, Columbia University College of Physicians and Surgeons, New York, USA; 2Department of Physiology and Molecular Biophysics, Columbia University College of Physicians and Surgeons, New York, USA; 3Department of Science, Borough of Manhattan Community College-CUNY, New York, USA. Email: rmg5@columbia.edu Received 30 June 2009; revised 10 July 2009; accepted 15 July 2009 ABSTRACT Purpose: To study the effect of time varying/ pulsing electromagnetic fields (PEMF) on bio- logical systems by measuring regrowth and the induction of elevated levels of the stress protein hsp70 in the regenerative model Planaria Duge- sia dorotocethala. Objective: The outcomes of studies using electromagnetic fields (EMF) are dependent on pulse design, field strength (mG), frequency (Hz), duration and magnetic field/rise time (dB/ dt). Standardization of effective pulse design is necessary to avoid continuing confu- sion in the investigation of pulsing electro- magnetic field (PEMF) technology. Information from studies on hsp70 protein induction and regrowth in transected Planaria provides in- formation on EMF efficacy for potential clinical application in the treatment of ischemia reper- fusion injury and the eventual inclusion of EMF prophylaxis prior to surgery. Materials and methods: Planaria were transected equidistant between the tip of the head and the tip of the tail. Individual head and tail portions from the same worm were placed in pond water and exposed to 8, 16 or 72 Hertz PEMF for one hour daily post transection under carefully controlled exposure conditions. Regrowth of heads and tails was measured in PEMF-exposed and sham control. Protein lysates from PEMF-exposed and sham control transected heads and tails were ana- lyzed for hsp70 levels by Western blot analyses. Conclusion: The degree of regrowth and hsp70 levels in transected heads and tails exposed to nanosecond PEMF exposures at 8, 16 or 72 Hz was frequency dependent. There are currently several views on the interaction mechanism involved in regrowth. Here we discuss two: in one [7,8] we propose a direct effect on the DNA of the PEMF consensus sequence, nCTCTn, referred to as electromagnetic field response elements (EMRE) in the promoter region of the stress response gene HSP70. In the second mechanism [28] it is proposed that EMF induce vibrations of proteins through a series of quan- tized low frequency phonon signals. Keywords: Planaria; Nanosecond EM Pulse; hsp70 Protein; Regrowth 1. INTRODUCTION Electromagnetic fields (EMF) of less than 1 Gauss strength have been shown to induce a variety of specific effects in cells and tissues over a wide frequency range of the EM spectrum [7,8]. Nanosecond pulsing electromag- netic field (PEMF) technology has assumed increasingly greater importance in clinical treatments [15,29,70]. As a measure of efficacy, we used the regenerative model Pla- naria, to test three nanosecond PEMF devices on regrowth of transected heads and tails and the induction of the stress response protein hsp70 [42,43,44,45]. Previously we showed that ELF-EMF induce elevated hsp70 protein levels through the responsiveness of a specific consensus DNA sequence EMRE (electromag- netic field response element, nCTCTn) on the heat shock 70 (HSP70) promoter [41,42,43,44,45]. This DNA do- main is upstream from the heat shock domain. Further- more, ELF-EMF induction of the hsp70 protein protects cells and limits the effects of subsequent stress, includ- ing sudden changes in temperature [12,30]. The degree of electromagnetic field-effects on bio- logical systems is known to be dependent on a number of criteria in the waveform pattern of the exposure sys- tem used; these include frequency, duration, wave shape, and relative orientation of the fields [6,29,32,33,39,40]. In some cases pulsed fields have demonstrated increased efficacy over static designs [19,21] in both medical and experimental settings. Abbreviations: Extremely low frequency (ELF), electromagnetic fields (EMF); nanosecond pulsing electromagnetic field (PEMF), heat shoc rotein (hsp), heat shock gene (HSP), frequency (Hz), Gauss (G), mag- netic field/rise time (dB/dt); mitogen-activated protein kinase (MAPK).
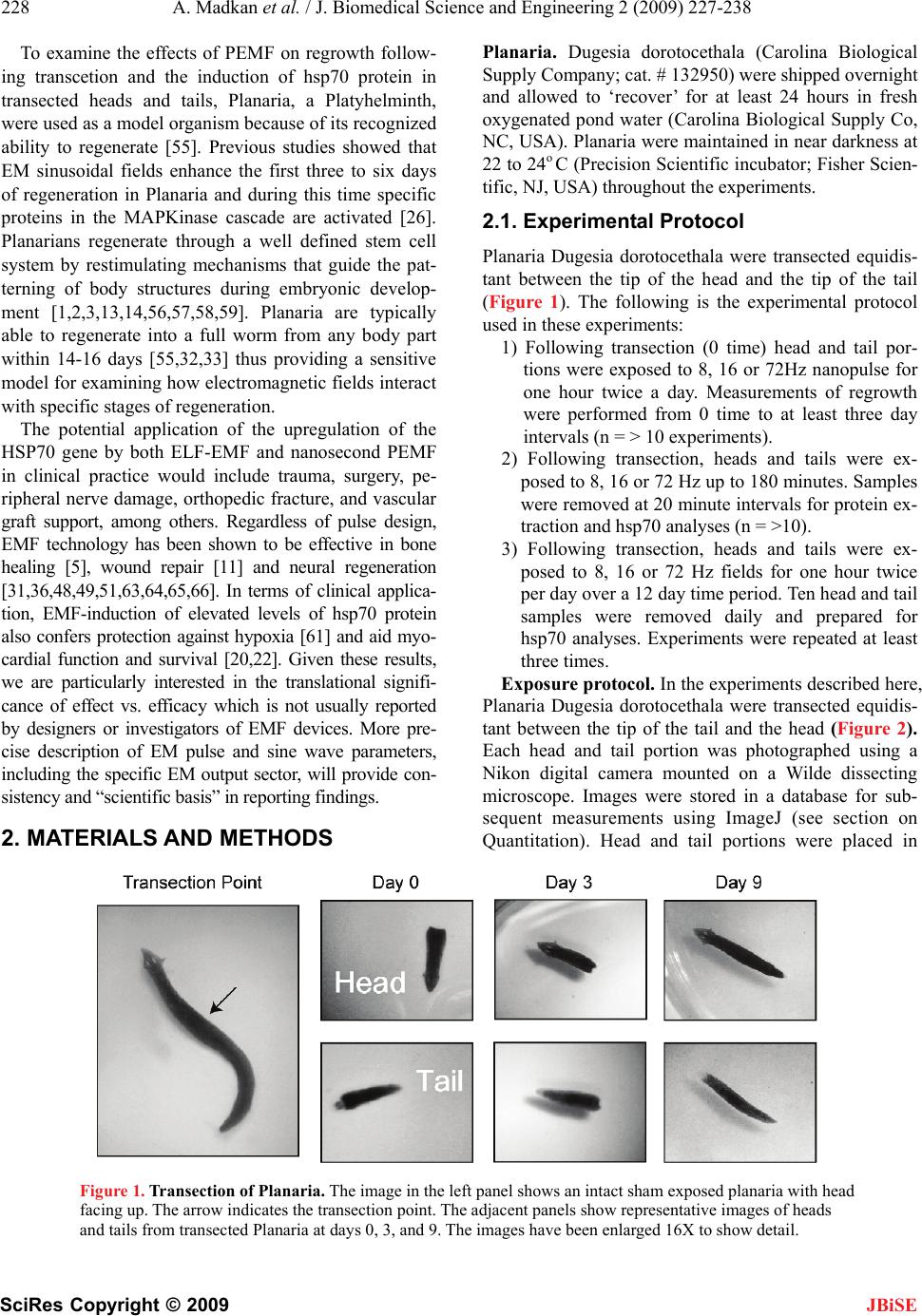 228 A. Madkan et al. / J. Biomedical Science and Engineering 2 (2009) 227-238 SciRes Copyright © 2009 JBiSE To examine the effects of PEMF on regrowth follow- ing transcetion and the induction of hsp70 protein in transected heads and tails, Planaria, a Platyhelminth, were used as a model organism because of its recognized ability to regenerate [55]. Previous studies showed that EM sinusoidal fields enhance the first three to six days of regeneration in Planaria and during this time specific proteins in the MAPKinase cascade are activated [26]. Planarians regenerate through a well defined stem cell system by restimulating mechanisms that guide the pat- terning of body structures during embryonic develop- ment [1,2,3,13,14,56,57,58,59]. Planaria are typically able to regenerate into a full worm from any body part within 14-16 days [55,32,33] thus providing a sensitive model for examining how electromagnetic fields interact with specific stages of regeneration. The potential application of the upregulation of the HSP70 gene by both ELF-EMF and nanosecond PEMF in clinical practice would include trauma, surgery, pe- ripheral nerve damage, orthopedic fracture, and vascular graft support, among others. Regardless of pulse design, EMF technology has been shown to be effective in bone healing [5], wound repair [11] and neural regeneration [31,36,48,49,51,63,64,65,66]. In terms of clinical applica- tion, EMF-induction of elevated levels of hsp70 protein also confers protection against hypoxia [61] and aid myo- cardial function and survival [20,22]. Given these results, we are particularly interested in the translational signifi- cance of effect vs. efficacy which is not usually reported by designers or investigators of EMF devices. More pre- cise description of EM pulse and sine wave parameters, including the specific EM output sector, will provide con- sistency and “scientific basis” in reporting findings. 2 . MATERIALS AND METHODS Planaria. Dugesia dorotocethala (Carolina Biological Supply Company; cat. # 132950) were shipped overnight and allowed to ‘recover’ for at least 24 hours in fresh oxygenated pond water (Carolina Biological Supply Co, NC, USA). Planaria were maintained in near darkness at 22 to 24o C (Precision Scientific incubator; Fisher Scien- tific, NJ, USA) throughout the experiments. 2.1. Experimental Protocol Planaria Dugesia dorotocethala were transected equidis- tant between the tip of the head and the tip of the tail (Figure 1). The following is the experimental protocol used in these experiments: 1) Following transection (0 time) head and tail por- tions were exposed to 8, 16 or 72Hz nanopulse for one hour twice a day. Measurements of regrowth were performed from 0 time to at least three day intervals (n = > 10 experiments). 2) Following transection, heads and tails were ex- posed to 8, 16 or 72 Hz up to 180 minutes. Samples were removed at 20 minute intervals for protein ex- traction and hsp70 analyses (n = >10). 3) Following transection, heads and tails were ex- posed to 8, 16 or 72 Hz fields for one hour twice per day over a 12 day time period. Ten head and tail samples were removed daily and prepared for hsp70 analyses. Experiments were repeated at least three times. Exposure protocol. In the experiments described here, Planaria Dugesia dorotocethala were transected equidis- tant between the tip of the tail and the head (Figure 2). Each head and tail portion was photographed using a Nikon digital camera mounted on a Wilde dissecting microscope. Images were stored in a database for sub- sequent measurements using ImageJ (see section on Quantitation). Head and tail portions were placed in Figure 1. Transection of Planaria. The image in the left panel shows an intact sham exposed planaria with head facing up. The arrow indicates the transection point. The adjacent panels show representative images of heads and tails from transected Planaria at days 0, 3, and 9. The images have been enlarged 16X to show detail.
 A. Madkan et al. / J. Biomedical Science and Engineering 2 (2009) 227-238 229 SciRes Copyright © 2009 JBiSE 250ns width @ 72 Hz 8 V 555 TIMER Figure 2. EM Probe Device. This device is designed to generate fast magnetic field pulses of low duty cycle in the near field region. The pulse length is about 250ns driven by a storage capacitor and a MOFSET switch that produces a 10A current in a field generating circuit element. The circuit element is just the single loop of the capacitor and switch circuit and does not contain any additional coil or turns of a conductor to enhance the magnetic field strength. The compact single loop design minimizes inductance and increases the speed of the circuit allowing a fast rise pulse pro- ducing a maximum magnetic field of the order of one Gauss at about 1cm from the device. The field is concentrated in a region of a few cm3 and falls off rapidly outside that region. The fast rise and fall of the current and magnetic field pulse implies a broad spectrum of Fourier components contain within the repeated pulse of the waveform. For the pulse rise time of about 10ns and a pulse frequency of 72Hz, those components extend from the fundamental fre- quency at 72Hz at the low end to at least 20MHz at the high frequency end. The particular distribution of frequen- cies is determined by the usual methods of Fourier analysis from the exact pulse shape. This wide range of fre- quency offer resonant interactions with the biological mechanisms of the organism being treated over a very wide range and could include molecular, cellular and multicellular level interactions. separate Petri dishes (Falcon 351007 60 x 15mm; Fisher Scientific, NJ, USA) containing pond water approxi- mately 0.6cm deep. Dishes were numbered so that heads and tails of the same worm could be identified for meas- urements. Dishes were placed on a firm base and the PEMF devices were attached to the dishes so that the entire area of the dish was exposed to a uniform field for one hour a day. All exposures took place within an incu- bator with the temperature maintained at 22-24o C and monitored with a thermocouple probe (sensitivity +/- 0.01oC; Physitemp, model BAT12, Hackensack, NJ, USA). Growth was assessed at three-day intervals from day 0 (immediately following transection and the onset of PEMF exposure) to day 12 post-transection. In a se- ries of separate experiments, pigmented eye spot devel- opment was monitored at 12 hour intervals in transected tails exposed to PEMF and sham control immediately following transection. The nanosecond PEMF device (EM-PROBE Technologies) in Figure 1 generates a near field fast magnetic pulse of 250 nanosecond (ns) duration, which is driven by a circuit containing a storage capacitor and a switch that produces a 10 A current in a field-generating circuit element. The single loop circuit does not contain any additional coils of a conductor to enhance the mag- netic field strength, and allows a rapid dB/dt pulse design producing a magnetic field of 1 Gauss approximately 1 cm from the device. The pulse begins with a peak strength of 1.4-1.7 Gauss that deteriorates to zero in about 200ns. At 72Hz this means that there is an active field for 0.00072% of the time. At 16 Hz there is an active field for 0.00016% of the time. At 7.8Hz there is an active field for 0.000078% of the time. The particular distribution of frequencies is determined by Fourier analysis from the exact pulse shape. This wide range of frequencies offers resonant interactions with the biological mechanisms of the organism being treated. The entire output circuit is optimized for rapid response by minimizing inductance. The wide range of frequencies offers resonant interactions with the biological mechanisms of the organism being treated over a wide range and might include molecular, cellular and multi- cellular interactions. Shielding: Both active (experimental) and sham- ex- posed (control) samples were enclosed in 30cm high, 15cm diameter cylindrical Mu metal containers (0.040" thickness) (Amuneal Corp. Philadelphia, PA). Detailed measurements of background magnetic fields in the in- cubator, harmonic distortion, DC magnetic fields and mean static magnetic fields in the incubators were pre- viously determined [35]. Protein lysates. In a separate series of experiments, protein was extracted at defined time periods from the transected heads and tails, both experimental and sham, to determine effect of PEMF on hsp70 levels. Lysates were prepared from the heads and the tails of exposed and sham exposed Planaria for analyses of hsp70 [41, 42,
 230 A. Madkan et al. / J. Biomedical Science and Engineering 2 (2009) 227-238 SciRes Copyright © 2009 JBiSE 45]. Protein concentrations were determined by the Bradford assay (Bio-Rad Redmond, WA, USA). Western blot. Lysed samples containing 30µg of pro- tein were subjected to sodium dodecyl sulfate gel elec- trophoresis (Fisher Scientific, NJ, USA) on 10% poly- acrylamide gels using appropriate molecular weight markers (Santa Cruz Biotechnology Inc, Santa Cruz, CA, USA) and the polypeptides were transferred to polyvi- nylidene fluoride membrane for immunoblotting. Blots were probed with anti-hsp70 antibody (1:10,000). The blots were then stripped using SuperSignal West Pico Sta- ble Peroxide Solution (Pierce, prod # 1859674, Fisher Scientific, NJ, USA) and SuperSignal West Pico Luminol Enhancer Solution (Pierce prod # 1859675, from Fisher Scientific, NJ, USA) and reprobed with anti-actin (1:1000) to confirm equivalent loading. Visualization was by the enhanced chemiluminescent detection system (Fisher Scientific, NJ, USA) as previously described [43]. Antibodies. The antibody to hsp70 was kindly provided by Dr. Richard Morimoto, Northwestern University. Statistical analysis: For determination of regrowth, multiple high-resolution digital pictures of Planaria heads and tails were taken at three day intervals and the rate of regrowth quantified using ImageJ v1.38 (http:// rsb.info.nih.gov/ij). Length measurements were cali- brated in millimeters and transposed to an Excel spread- sheet for statistical analysis. Lengths were normalized to the 0-time. Length differences over each 3-day interval were then subjected to 2-sample t-tests to assess signifi- cant differences in mean growth between the control and exposed conditions for both heads and tails. An addi- tional analysis was conducted in SAS v9.1 (www. sas.com) to assess the significance of an interaction ef- fect, i.e., whether the exposure had greater effect on ei- ther heads or tails. For this a ‘mixed effects’ analysis was performed which modeled the average length at 3-day intervals as a linear sum of the fixed effects of exposure (experimental vs. control), worm portion (heads vs. tails) and their interaction. For quantification of the hsp70 and beta-actin levels the films from Western blot were scanned and saved as digital images (Figure 3). The densities of the bands were quantified using the histogram function in ImageJ and values transposed to Excel for statistical analysis (2 sample t-tests). A minimum of three replications of each assay were conducted. 2.2 Western Blots Quantitation of Hsp70 and β-actin Bands Images from films were scanned into a computer and analyzed with Image J v1.37 (NIH). The analyze func- tion for gels was used to plot the spatial signal density for each lane of hsp70 (see Figure 2). The same was done for the β-actin controls (not shown). Figure 2 plots the mean hsp70/-actin ratios for PEMF and Control con- ditions. These values were imported into a Microsoft Excel spreadsheet where the signal value of hsp70 was divided by the value of the-actin signal in the same lane in order to normalize against variable loading volumes. -actin is a housekeeping gene and unaffected by EMF or PEMF. Statistical analyses were also conducted in Excel using the Data Analysis toolbox. 3. RESULTS The extraordinary ability of Planaria to regenerate after injury is attributed to the presence of totipotent neoblasts capable of differentiating into all of the tissue types [1, 4]. Perhaps most impressive is their ability to regenerate the nervous system. When transected the tail region forms a new head complete with bilateral optic nerves and eyes, a two-lobed brain, and a pair of ventral nerve cords (VNC) [2,13,14]. 3.1. PEMF Accelerates Rate of Regrowth of Transected Planaria To assess the efficacy of the nanosecond PEMFs on Planaria regeneration, the length of ELF-EMF-exposed and sham control heads and tails were measured at three- day intervals starting immediately following transection (Figure 4 A,B,C). The heads and tails of all transected Planaria (experimental and control) regrew to normal viable worms. The initial experiments used PEMF at 72Hz.to measure regrowth of transected heads and tails. As previously demonstrated using ELF-EMF [26], ac- celerated tail regrowth was significant at days 3 and 6 post transaction (p=0.05). In contrast to the effect of exposure to72 Hz PEMF, 8Hz PEMF exposure on the tail portion showed the greatest response to any of the Figure 3. Images from films were scanned into computer and analyzed with Image J v1.37 (NIH). The ‘analyze function for gels’ was used to plot the spatial signal density for each lane of hsp70. The same was done for the β-actin controls (not shown). These values were imported into a Microsoft Excel spreadsheet where the signal value of hsp70 was divided by the value of the β-actin signal in the same lane in order to normalize against vari- able loading volumes. β-actin is a so-called ‘housekeeping gene’ and unaffected by EMF or EMP. Statistical analyses were also conducted in Excel using the Data Analysis toolbox.
 A. Madkan et al. / J. Biomedical Science and Engineering 2 (2009) 227-238 231 SciRes Copyright © 2009 frequencies to which the transected heads and tails were exposed. The sham control samples in general showed very little change in length over this time period (Figure 3B). A reduced response to the 16Hz PEMF (Figure 3C) was measured in both transected heads and tails when compared with 72 and 8Hz (Figure 3A, B). EM Probe Regeneration - 72 Hz TAILS (normalized) 0.4 0.6 0.8 1 1.2 1.4 1.6 1.8 0346 Days Ratio to Day EM Probe 72 Hz Control Figure 4A. Regeneration of heads and tails following transection of Planaria. Histograms show- ing the mean length of control and PEMF-exposed heads and tails immediately post-transection (0 time), and 3 to 9 days post-transection. (A) Mean length ± 2 standard error of the mean (SEM) of ex- posed heads (HE, n=48) and sham exposed heads (HC, n=27) at each time. (B) Mean length ± 2SEM of exposed tails (TE, n=20) and sham exposed tails (TC, n=12) at each time. The asterisks indicate a significant statistical difference in the rate of regeneration between exposed and sham exposed heads and tails assessed by 2-sample t-tests of mean difference in length. A. Exposure to 72Hz accelerated tail regrowth was significant at days 3 and 6. post-transection [data normalized against day zero (n=3)]. The effect of the 72Hz EM probe is `statistically significant (p=0.05) at three and six days on the tail portion. The heads and tails of all transected Planaria (ex- perimental and control) regrew to normal viable worms by 20 days. There was little or no measure- able effect on regrowth in head portion. EM Probe Regeneration - 8 Hz TAILS (normalized) 0.4 0.6 0.8 1 1.2 1.4 1.6 1.8 0234610 Days Ratio to Day EM Probe 8 Hz Control Figure 4B. In contrast to the effect of exposure to 72 Hz PEMF, transected tails exposed to the 8Hz EM probe show a steady increase in length. The effect of 8Hz PEMF on the tail portion showed the greatest response to any of the frequencies to which the transected heads and tails were exposed. The sham con- trol samples and the head exposed samples showed very little change in length over this time period. JBiSE
 232 A. Madkan et al. / J. Biomedical Science and Engineering 2 (2009) 227-238 SciRes Copyright © 2009 JBiSE EM Probe Regeneration - 16 Hz HEADS (normalized) 0.4 0.6 0.8 1 1.2 1.4 1.6 1.8 03467912 Days Ratio to Day 0 EM Probe 16 Hz Control Figure 4C. A reduced response to the 16Hz PEMF was measured in both transected heads and tails when compared with 72 and 8Hz. Of particular interest was the general trend of the re- sponsiveness of the tail to the three frequencies tested as compared to the head portions (Figure 4 A, B, C). The third day after transection has been shown to be the time of maximal ELF-EMF-stimulated growth of the tail [26]. During normal regeneration this is the developmental stage when the neural networks are actively forming [13, 14] and the ventral nerve cords (VNC) are extending from the head into the newly formed tail. 3.2. PEMF Activation of Hsp70 Associated with Injury and Repair A significant elevation in hsp70 levels was evident in tail portions that were exposed to the nanosecond PEMF using all three frequencies at 0, 20, 40, 60 and 80 min- utes (Figure 5). We also looked at potential differences in induction of hsp70 protein, comparing all separate PEMF exposures to the sham control. Tails that were exposed to 16Hz EM-Probe fields showed the highest hsp70 production (p= .000007.91). In terms regrowth, the 8Hz tails shows the quickest response to PEMF (Figure 4B). The 72Hz also shows a noted difference between exposed and unexposed worms (Figure 4A). The 16Hz was too inconsistent in order to draw any conclusions (data not shown). We believe the accelerated regrowth above is consis- tent with the role of hsp70 as a chaperone that monitors the folding of proteins during repair. PEMF induction of the HSP70 gene may result from events triggered by the activation of ERK that utilize a unique consensus se- quence (electromagnetic field response element; EMRE) upstream from the heat shock consensus sequence [8, 9,10]. The increase paralleled the accelerated regeneration noted above and is consistent with the role of hsp70 as a chaperone that monitors the folding of proteins during repair. Induction of the HSP70 gene may result from events triggered by the activation of ERK that utilize a unique consensus sequence (electromagnetic field re- sponse element; EMRE) upstream from the heat shock consensus sequence [42,47]. Stress response protein hsp70 levels are elevated after exposure to PEMF [43, 44,45]. We next examined the effect of nanosecond PEMF at 8, 16 and 72 Hz exposures on levels of this protein. Earlier studied had shown that hsp70 levels (Figure 5A,B) depicts mean hsp70/-actin ratios and 95% CI for both 16Hz and 72Hz exposure conditions on heads and tails respectively vs. their control counterparts. With both frequencies we observed a spike in hsp70 lev- els at 40 minutes which concurs with our previous data [41,45] and as previously noted the hsp70 levels return to normal levels by 120 minutes. [Mean hsp70/-actin ratios for 16Hz EMP Heads (n=14), 16Hz EMP Tails (n=14), 72Hz EMP Tails (n=17) and 72Hz EMP Heads (n=13).] As expected, measurements of hsp70 during regrowth showed little to no effect of exposures. 4. DISCUSSION In the experiments reported here we examined nanosec- ond PEMF, rapid dB/dt using 8, 16 and 72Hz EM probe devices on induction of hsp70 levels and Planarian head and tail regrowth post-transection. Our data show that the induction of elevated hsp70 levels and regrowth fol- lowing transection are frequency specific; tail portions that regenerate nervous system, brain and eyes, are more responsive to the EM probe device than head portions. The effectiveness of time varying electromagnetic fields on biological systems has been shown to be dependent on pulse design; frequency, duration, magnetic field/rise time (dB/dt) [29,30]. Measurements of EMF induced
 A. Madkan et al. / J. Biomedical Science and Engineering 2 (2009) 227-238 233 SciRes Copyright © 2009 JBiSE Planaria EM Pulse Solo - 16 Hz and Control 0 2 4 6 8 10 12 0 20406080 Exposure Time (minutes) Mean Hsp70 / beta-actin Protein Levels (arbitary units) Control Heads Control Tails Exposed Heads Exposed Tails Figure 5A. Histogram showing a quantitative analysis of hsp70 protein levels from regenerating heads, tails, and sham controls. The relative protein levels are expressed in arbitrary units based on the intensity of the hsp70 protein band from the Western blots. The PEMF exposed tails show an obvious spike between 20 and 40 minutes. Mean hsp70/β-actin ratios for 16 Hz PEMF heads (n=14), 16 Hz PEMF tails (n=14), 72 Hz PEMF tails (n=17) and 72 Hz PEMF heads (n=13). The control groups for each sample had a sample size equal to that of its corresponding PEMF group. Error bars represent 95% confidence intervals ( 2*Standard Error).] Planaria TAILS Hsp70 - Days 0 5000 10000 15000 20000 25000 30000 036 Days Mean Hsp70 Levels (arbitrary units) 8 Hz 16 Hz 72 Hz Control Figure 5B. Histogram showing a quantitative analysis of hsp70 protein levels from regenerating heads, tails, and sham controls.[Mean hsp70/β-actin ratios for 16 Hz EMP heads (n=14), 16 Hz PEMF tails (n=14), 72 Hz PEMF tails (n=17) and 72 Hz PEMF heads (n=13)]. The control groups for each sample had a sample size equal to that of its corresponding EMP group. Error bars represent 95% confidence intervals ( 2*Standard Error).] hsp70 levels and induction of post-transected re-growth were used as markers for repair efficacy in this study. Standardization of effective pulse design is necessary to avoid continuing confusion in the investigation of puls-
 234 A. Madkan et al. / J. Biomedical Science and Engineering 2 (2009) 227-238 SciRes Copyright © 2009 JBiSE ing electromagnetic field (PEMF) technology. The data from hsp70 protein induction and regrowth in Planaria, a regeneration model, as a measure of EMF efficacy, sug- gest excellent opportunity for clinical use in treatment of ischemia reperfusion injury and the inclusion of EMF prophylaxis prior to surgery. Our focus in these experi- ments was on: (a) regrowth of head and tail portions following transection, (b) immediacy of hsp70 induction during regeneration of heads and tails, (c) levels of hsp70 elevation during regeneration of heads and tails and (d) duration of hsp70 elevation. We have shown that the tail portion of the transected Planaria is more sensi- tive to different frequencies both in the induction of hsp70 levels and in regrowth. Furthermore, these changes in tail metabolism occur at different times de- pending upon the frequency used in the exposure. 4.1. Interaction Mechanisms How weak electromagnetic (EM) fields interact with DNA to stimulate protein synthesis remains unknown; EMF interaction with cells and tissues has been exam- ined in a variety of in vivo and in vitro systems including enzyme reactions [10], Drosophila [25,27,72], yeast [71] cultured cells [23,24,53] and on regeneration in Planaria [26]. Currently no definitive mechanism exists to explain how EMF interact with DNA and proteins in cells and tissues, although several theories have been proposed including cyclotron resonance [40], and a modification of this idea [6,37]. There is some evidence that EM fields can affect DNA, both directly and indirectly [9]. We have shown a unique DNA sequence in a specific domain on the HSP70 promoter that is sensitive to EM field exposures and this observation has contributed to the understanding, in part, of how ELF-EMF affects biological systems. There are specific regions on the promoters of some genes, HSP70 and c-myc for example, that contain this consensus sequence and when this sequence is trans- fected into promoter of reporter genes, these genes be- come sensitive to EMF, whereas before they were not. [41,42,43,44,45]. EM fields initiate up regulation of the HSP70 gene, increase mRNA transcripts and hsp70 pro- tein levels. The responsiveness is dependent on the number of copies of the nCTCTn consensus sequence. [42]. Electromagnetically, responsive elements act as ‘sensors’. This is an HSF-1 dependent process as shown by electrophoretic mobility shift assays (EMSA) of pro- tein extracts from HL60 cells exposed to EMF. HSF1- DNA binding activity was demonstrated by a super- shifted band. The magnetic field-inducible heat shock element-binding activity is HSE sequence-specific and contains HSF1 [45]. Because of the demonstrated effect of EMF on elec- tron transfer reactions, it has been proposed that dis- placement of electrons in the H-bonds that hold DNA together can lead to DNA chain separation, thus initiat- ing transcription [7,8]. The resultant charging due to electron displacement on the dynamics of DNA chain separation suggests that electron transfer could favor separation of base pairs e.g. nCTCTn, with the EM field sensitive DNA sequence acting as the first order iterative mechanism. In the case of Planaria the DNA in the toti- potent stem cells created by the injury may be respond- ing directly to the EM fields. It has been shown that DNA-mediated charge transport and the oxidative dam- age that results are extremely sensitive to variations in the sequence and conformational-adaptive response leading to stacking of the intervening bases [18,62]. Protein-binding to DNA, for example, through one or more of the MAPKinase cascades, modulates long-range charge transport negatively and positively depending upon the specific protein DNA interactions in play [52,54,68,69]. A possible mechanism for EMF initiation of protein synthesis is by acting on the de-localized π electrons in the base pairs that hold the DNA strands together. This is one way in which the effect of EMF in ELF range can be due to the resulting current. The charging of molecular complexes has been shown to lead to their disaggrega- tion [10], so local charging would be expected to cause the DNA strands to disaggregate. A possible sequence of steps may start with EMF displacing electrons and charging DNA segments, followed by disaggregation of DNA strands as a result of the charging. The properties of the CTCT bases suggest that they may be involved in the first step in a molecular mecha- nism for EMF activation of protein synthesis. They have low electron affinities, so electrons would be more easily displaced by the EMF. Also, the CTCT are pyrimidines, and when the H-bonds split between CTCT and the GAGA (purines) bases on the complementary chain, the smaller area that results would require less energy, and make the disaggregation more favorable [7]. Two recent studies of molecular properties of DNA add support to this proposed mechanism of DNA disag- gregation. One paper compared the lifetimes of excita- tion induced by ultraviolet (UV) light in different DNA structures [60]. Apparently, the induced fluorescence had significantly longer lifetimes in DNA chains composed of A and G bases than in chains with C and T bases. The critical non-thermal stress protein sequences, the CTCT pyrimidines, were found to have the shortest UV stimu- lated fluorescence lifetimes. Their conjugate GAGA purines were found to have lifetimes that are approxi- mately an order of magnitude longer. Fluorescence lifetimes are measured in the picosecond time range, a relevant time scale for molecular rear- rangements. Since the fluorescence lifetimes relate to the properties of the excited molecule and not to what caused the excitation, the measured UV excitation life-
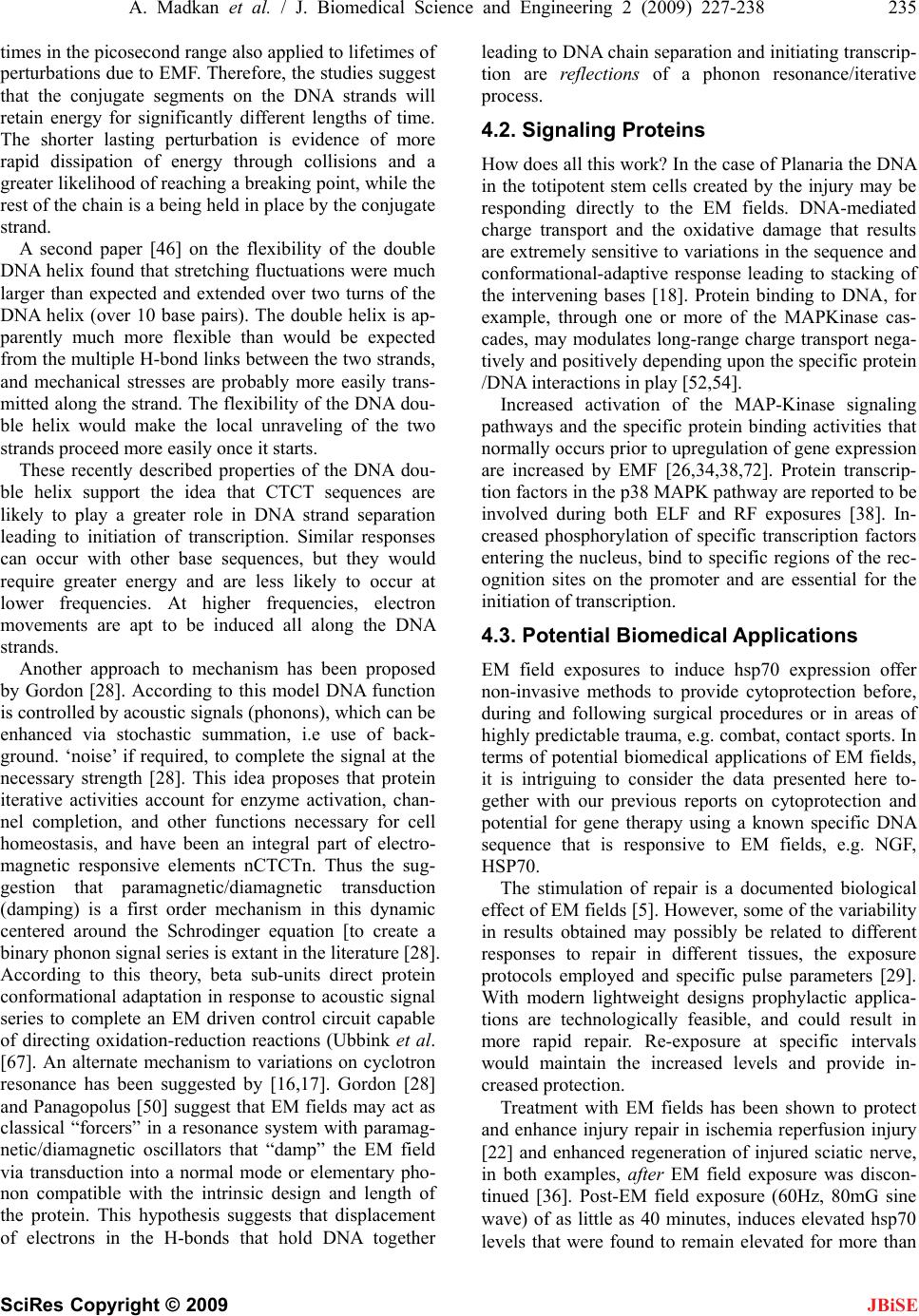 A. Madkan et al. / J. Biomedical Science and Engineering 2 (2009) 227-238 235 SciRes Copyright © 2009 JBiSE times in the picosecond range also applied to lifetimes of perturbations due to EMF. Therefore, the studies suggest that the conjugate segments on the DNA strands will retain energy for significantly different lengths of time. The shorter lasting perturbation is evidence of more rapid dissipation of energy through collisions and a greater likelihood of reaching a breaking point, while the rest of the chain is a being held in place by the conjugate strand. A second paper [46] on the flexibility of the double DNA helix found that stretching fluctuations were much larger than expected and extended over two turns of the DNA helix (over 10 base pairs). The double helix is ap- parently much more flexible than would be expected from the multiple H-bond links between the two strands, and mechanical stresses are probably more easily trans- mitted along the strand. The flexibility of the DNA dou- ble helix would make the local unraveling of the two strands proceed more easily once it starts. These recently described properties of the DNA dou- ble helix support the idea that CTCT sequences are likely to play a greater role in DNA strand separation leading to initiation of transcription. Similar responses can occur with other base sequences, but they would require greater energy and are less likely to occur at lower frequencies. At higher frequencies, electron movements are apt to be induced all along the DNA strands. Another approach to mechanism has been proposed by Gordon [28]. According to this model DNA function is controlled by acoustic signals (phonons), which can be enhanced via stochastic summation, i.e use of back- ground. ‘noise’ if required, to complete the signal at the necessary strength [28]. This idea proposes that protein iterative activities account for enzyme activation, chan- nel completion, and other functions necessary for cell homeostasis, and have been an integral part of electro- magnetic responsive elements nCTCTn. Thus the sug- gestion that paramagnetic/diamagnetic transduction (damping) is a first order mechanism in this dynamic centered around the Schrodinger equation [to create a binary phonon signal series is extant in the literature [28]. According to this theory, beta sub-units direct protein conformational adaptation in response to acoustic signal series to complete an EM driven control circuit capable of directing oxidation-reduction reactions (Ubbink et al. [67]. An alternate mechanism to variations on cyclotron resonance has been suggested by [16,17]. Gordon [28] and Panagopolus [50] suggest that EM fields may act as classical “forcers” in a resonance system with paramag- netic/diamagnetic oscillators that “damp” the EM field via transduction into a normal mode or elementary pho- non compatible with the intrinsic design and length of the protein. This hypothesis suggests that displacement of electrons in the H-bonds that hold DNA together leading to DNA chain separation and initiating transcrip- tion are reflections of a phonon resonance/iterative process. 4.2. Signaling Proteins How does all this work? In the case of Planaria the DNA in the totipotent stem cells created by the injury may be responding directly to the EM fields. DNA-mediated charge transport and the oxidative damage that results are extremely sensitive to variations in the sequence and conformational-adaptive response leading to stacking of the intervening bases [18]. Protein binding to DNA, for example, through one or more of the MAPKinase cas- cades, may modulates long-range charge transport nega- tively and positively depending upon the specific protein /DNA interactions in play [52,54]. Increased activation of the MAP-Kinase signaling pathways and the specific protein binding activities that normally occurs prior to upregulation of gene expression are increased by EMF [26,34,38,72]. Protein transcrip- tion factors in the p38 MAPK pathway are reported to be involved during both ELF and RF exposures [38]. In- creased phosphorylation of specific transcription factors entering the nucleus, bind to specific regions of the rec- ognition sites on the promoter and are essential for the initiation of transcription. 4.3. Potential Biomedical Applications EM field exposures to induce hsp70 expression offer non-invasive methods to provide cytoprotection before, during and following surgical procedures or in areas of highly predictable trauma, e.g. combat, contact sports. In terms of potential biomedical applications of EM fields, it is intriguing to consider the data presented here to- gether with our previous reports on cytoprotection and potential for gene therapy using a known specific DNA sequence that is responsive to EM fields, e.g. NGF, HSP70. The stimulation of repair is a documented biological effect of EM fields [5]. However, some of the variability in results obtained may possibly be related to different responses to repair in different tissues, the exposure protocols employed and specific pulse parameters [29]. With modern lightweight designs prophylactic applica- tions are technologically feasible, and could result in more rapid repair. Re-exposure at specific intervals would maintain the increased levels and provide in- creased protection. Treatment with EM fields has been shown to protect and enhance injury repair in ischemia reperfusion injury [22] and enhanced regeneration of injured sciatic nerve, in both examples, after EM field exposure was discon- tinued [36]. Post-EM field exposure (60Hz, 80mG sine wave) of as little as 40 minutes, induces elevated hsp70 levels that were found to remain elevated for more than
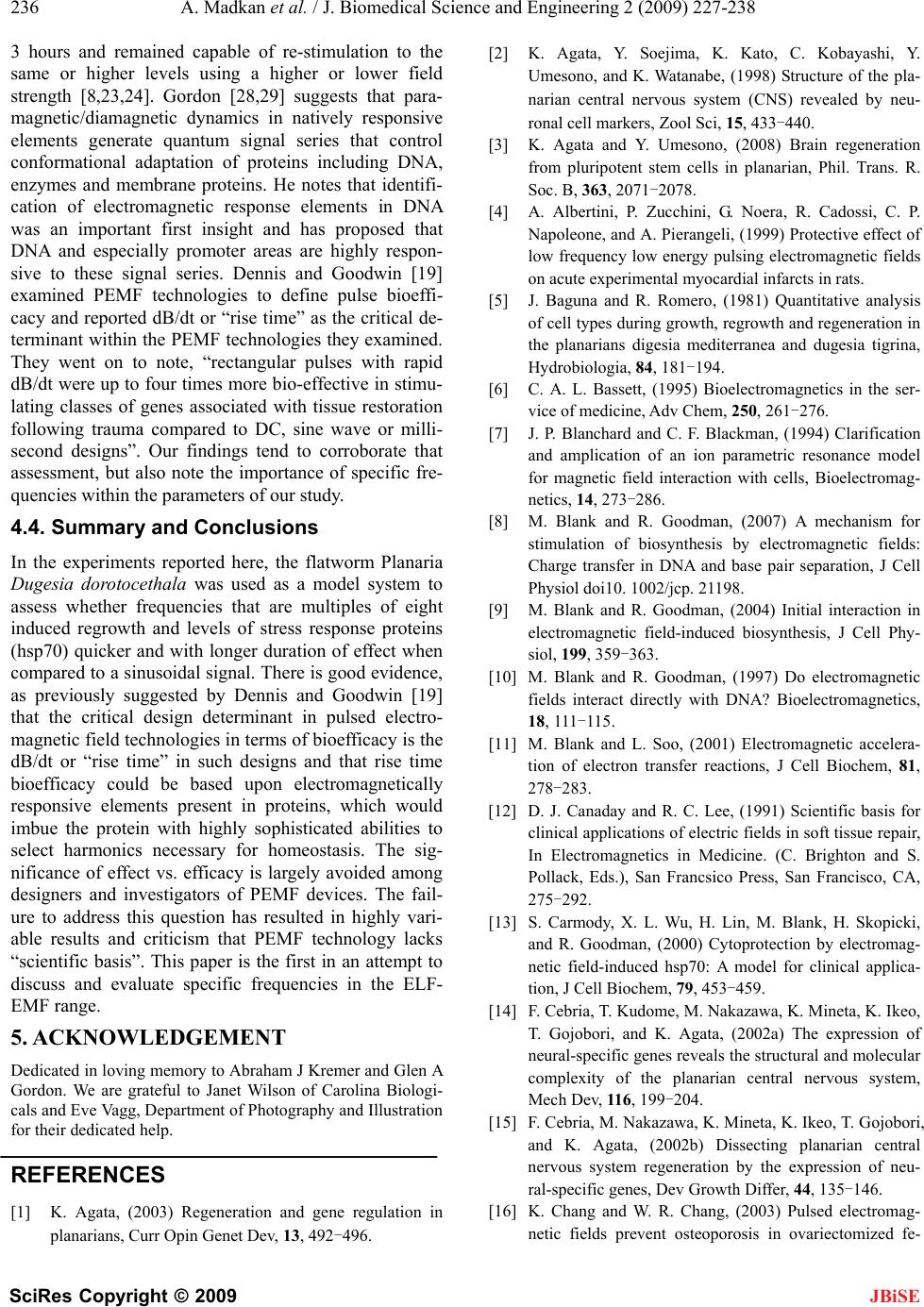 236 A. Madkan et al. / J. Biomedical Science and Engineering 2 (2009) 227-238 SciRes Copyright © 2009 JBiSE 3 hours and remained capable of re-stimulation to the same or higher levels using a higher or lower field strength [8,23,24]. Gordon [28,29] suggests that para- magnetic/diamagnetic dynamics in natively responsive elements generate quantum signal series that control conformational adaptation of proteins including DNA, enzymes and membrane proteins. He notes that identifi- cation of electromagnetic response elements in DNA was an important first insight and has proposed that DNA and especially promoter areas are highly respon- sive to these signal series. Dennis and Goodwin [19] examined PEMF technologies to define pulse bioeffi- cacy and reported dB/dt or “rise time” as the critical de- terminant within the PEMF technologies they examined. They went on to note, “rectangular pulses with rapid dB/dt were up to four times more bio-effective in stimu- lating classes of genes associated with tissue restoration following trauma compared to DC, sine wave or milli- second designs”. Our findings tend to corroborate that assessment, but also note the importance of specific fre- quencies within the parameters of our study. 4.4. Summary and Conclusions In the experiments reported here, the flatworm Planaria Dugesia dorotocethala was used as a model system to assess whether frequencies that are multiples of eight induced regrowth and levels of stress response proteins (hsp70) quicker and with longer duration of effect when compared to a sinusoidal signal. There is good evidence, as previously suggested by Dennis and Goodwin [19] that the critical design determinant in pulsed electro- magnetic field technologies in terms of bioefficacy is the dB/dt or “rise time” in such designs and that rise time bioefficacy could be based upon electromagnetically responsive elements present in proteins, which would imbue the protein with highly sophisticated abilities to select harmonics necessary for homeostasis. The sig- nificance of effect vs. efficacy is largely avoided among designers and investigators of PEMF devices. The fail- ure to address this question has resulted in highly vari- able results and criticism that PEMF technology lacks “scientific basis”. This paper is the first in an attempt to discuss and evaluate specific frequencies in the ELF- EMF range. 5. ACKNOWLEDGEMENT Dedicated in loving memory to Abraham J Kremer and Glen A Gordon. We are grateful to Janet Wilson of Carolina Biologi- cals and Eve Vagg, Department of Photography and Illustration for their dedicated help. REFERENCES [1] K. Agata, (2003) Regeneration and gene regulation in planarians, Curr Opin Genet Dev, 13, 492-496. [2] K. Agata, Y. Soejima, K. Kato, C. Kobayashi, Y. Umesono, and K. Watanabe, (1998) Structure of the pla- narian central nervous system (CNS) revealed by neu- ronal cell markers, Zool Sci, 15, 433-440. [3] K. Agata and Y. Umesono, (2008) Brain regeneration from pluripotent stem cells in planarian, Phil. Trans. R. Soc. B, 363, 2071-2078. [4] A. Albertini, P. Zucchini, G. Noera, R. Cadossi, C. P. Napoleone, and A. Pierangeli, (1999) Protective effect of low frequency low energy pulsing electromagnetic fields on acute experimental myocardial infarcts in rats. [5] J. Baguna and R. Romero, (1981) Quantitative analysis of cell types during growth, regrowth and regeneration in the planarians digesia mediterranea and dugesia tigrina, Hydrobiologia, 84, 181-194. [6] C. A. L. Bassett, (1995) Bioelectromagnetics in the ser- vice of medicine, Adv Chem, 250, 261-276. [7] J. P. Blanchard and C. F. Blackman, (1994) Clarification and amplication of an ion parametric resonance model for magnetic field interaction with cells, Bioelectromag- netics, 14, 273-286. [8] M. Blank and R. Goodman, (2007) A mechanism for stimulation of biosynthesis by electromagnetic fields: Charge transfer in DNA and base pair separation, J Cell Physiol doi10. 1002/jcp. 21198. [9] M. Blank and R. Goodman, (2004) Initial interaction in electromagnetic field-induced biosynthesis, J Cell Phy- siol, 199, 359-363. [10] M. Blank and R. Goodman, (1997) Do electromagnetic fields interact directly with DNA? Bioelectromagnetics, 18, 111-115. [11] M. Blank and L. Soo, (2001) Electromagnetic accelera- tion of electron transfer reactions, J Cell Biochem, 81, 278-283. [12] D. J. Canaday and R. C. Lee, (1991) Scientific basis for clinical applications of electric fields in soft tissue repair, In Electromagnetics in Medicine. (C. Brighton and S. Pollack, Eds.), San Francsico Press, San Francisco, CA, 275-292. [13] S. Carmody, X. L. Wu, H. Lin, M. Blank, H. Skopicki, and R. Goodman, (2000) Cytoprotection by electromag- netic field-induced hsp70: A model for clinical applica- tion, J Cell Biochem, 79, 453-459. [14] F. Cebria, T. Kudome, M. Nakazawa, K. Mineta, K. Ikeo, T. Gojobori, and K. Agata, (2002a) The expression of neural-specific genes reveals the structural and molecular complexity of the planarian central nervous system, Mech Dev, 116, 199-204. [15] F. Cebria, M. Nakazawa, K. Mineta, K. Ikeo, T. Gojobori, and K. Agata, (2002b) Dissecting planarian central nervous system regeneration by the expression of neu- ral-specific genes, Dev Growth Differ, 44, 135-146. [16] K. Chang and W. R. Chang, (2003) Pulsed electromag- netic fields prevent osteoporosis in ovariectomized fe-
 A. Madkan et al. / J. Biomedical Science and Engineering 2 (2009) 227-238 237 SciRes Copyright © 2009 JBiSE male rat model: A prostaglandin E2 associated process, Bioelectromagnetics, 24, 189-198. [17] K. C. Chou, (1988) Review: Low frequency collective motion in biomacromolecules and its biological functions, Biophysical Chemistry, 30, 3-48. [18] K. C. Chou, (1984) Low frequency vibration of DNA molecules, Biochemical Journal, 221, 27-31. [19] P. J. Dandliker, R. E. Holmlin, and J. K. Barton, (1997) Oxidative thymine repair in the DNA helix, Science, 275, 1465-1468. [20] R. Dennis and T. Goodwin, (2003) Physiological and molecular genetics effects of time-varying electromag- netic fields on human neuronal cells, NASA Technical Paper TP-2003-212054. [21] A. L. DiCarlo, J. M. Farrell, and T. A Litovitz, (1999) Myocardial protection conferred by electromagnetic fields, Circulation, 99, 813-816. [22] C. Eichwald and J. Wallaczek, (1996) Activation de- pendent and biphasic electromagneetic field effects: Model based on cooperative enzyme kinetics in cellular signaling, Bioelectromagnetics, 17(6), 427-35. [23] I. George, M. S. Geddis, Z. Lill, H. Lin, T. Gomez, M. Blank, M. C. Oz, and R. Goodman, (2008) Myocardial function improved by electromagnetic field induction of stress protein hsp70. J Cell Physiol DOI: 10. 1002/jcp 21461. [24] R. Goodman and M. Blank, (2002) Insights into electro- magnetic interaction mechanisms, J Cell Physiol, 192, 16-22. [25] R. Goodman and M. Blank, (1998) Magnetic field stress induces expression of hsp70, Cell Stress/Chaperones 3, 79-88. [26] R. Goodman and A. S. Henderson, (1988) Exposure of salivary gland cells to low-frequency electromagnetic fields alters polypeptide synthesis, Proc Natl Acad Sci U S A, 85, 3928-3932. [27] R. Goodman, Y . A. Lin, M. Geddis, S. Hodge, S. Panta- zatos, M. Blank, and R. Ambron, (2009) Electromagnetic fields activate the ERK cascade, increase hsp70 protein levels and promote regeneration in Planaria, International Journal of Radiation Biology, In Press. [28] R. Goodman, D. Weisbrot, A. Uluc, and A. Henderson, (1992) Transcription in Drosophila melanogaster salivary gland cells is altered following exposure to low fre- quency electromagnetic fields: Analysis of chromosome 3R, Bioelectromagnetics, 13, 111-118. [29] G. Gordon, (2008) Extrinsic electromagnetic fields, low frequency (phonon) vibrations and control of cell func- tion: A non-linear resonance system, J Biomed Sci and Engineering, 1, 152-156. [30] G. Gordon, (2007) Designed electromagnetic pulsed therapy: Clinical applications, J Cell Physiol, 212, 579- 582. [31] L. Han, H. Lin, M. Head, M. Jin, M. Blank, and R. Goodman, (1998) Application of magnetic field-induced heat shock protein 70 for presurgical cytoprotection, J Cell Biochem, 71, 577-583. [32] H. Ito and C. A. Bassett, (1983) Effect of weak, pulsing electromagnetic fields on neural regeneration in the rat, Clin Orthop Relat Res, 181, 283-290. [33] K. A. Jenrow, C. H. Smith, and A. R. Liboff, (1996) Weak extremely low frequency magnetic field-induced regeneration anomalies in the planarian dugesia tigrina, Bioelectromagnetics, 17, 467-474. [34] K. A. Jenrow, C. H. Smith, and A. R. Liboff, (1995) Weak extremely low frequency magnetic fields and regeneration in the planarian dugesia tigrina, Bioelectro- magnetics, 16, 106-112. [35] M. Jin, M. Blank, and R. Goodman, (2000) ERK1/2 phosphorylation, induced by electromagnetic fields, di- minishes during neoplastic transformation, J Cell Bio- chem, 78, 371-379. [36] M. Jin, H. Lin, L. Han, M. Opler, S. Maurer, M. Blank, and R. Goodman, (1997) Biological and technical vari- ables in c-myc expression in HL60 cells exposed to 60Hz electromagnetic fields, Bioelectrochem Bioenerg, 44, 111 - 120. [37] M. Kanje, A. Rusovan, B. Sisken, and G. Lundborg, (1993) Pretreatment of rats with pulsed electromagnetic fields enhances regeneration of the sciatic nerve, Bio- electromagnetics, 14, 353-359. [38] V. V. Lednev, (1991) Possible mechanism for influence of magnetic fields on biological systems, Bioelectro- magnetics, 12, 71-75. [39] D. Leszczynski, S. Joenvaara, J. Reivinen, and R. Kuokka, (2002) Non-thermal activation of the hsp27/ p38MAPK stress pathway by mobile phone radiation in human endothelial cells: molecular mechanism for can- cer-and blood-brain barrier-related effects, Differentia- tion, 70, 120-129. [40] A. Liboff, (2004) Toward and electromagnetic paradigm for biology and medicine, Journal of Alternative and Complementary Medicine, 1, 41-47. [41] A. Liboff, (1985) Geomagnetic cyclotron resonance in membrane transport, J Biol Physics, 13, 99-102. [42] H. Lin, M. Blank, M. Head, and R. Goodman, (1999) A magnetic field-responsive domain in the human HSP70 promoter, J Cell Biochem, 75, 170-176. [43] H. Lin, M. Blank, K. R. Haseroth, and R. Goodman, (2001) Regulating genes with electromagnetic response elements, J Cell Biochem, 81, 143-148. [44] H. Lin, L. Han, M. Blank, M. Head, and R. Goodman, (1998a) Magnetic field activation of protein-DNA bind- ing, J Cell Biochem, 70, 297-303. [45] H. Lin, M. Head, M. Blank, L. Han, M. Jin, R. Goodman, (1998b) Myc-mediated transactivation of HSP70 expres- sion following exposure to magnetic fields, J Cell Bio- chem, 69, 181-188.
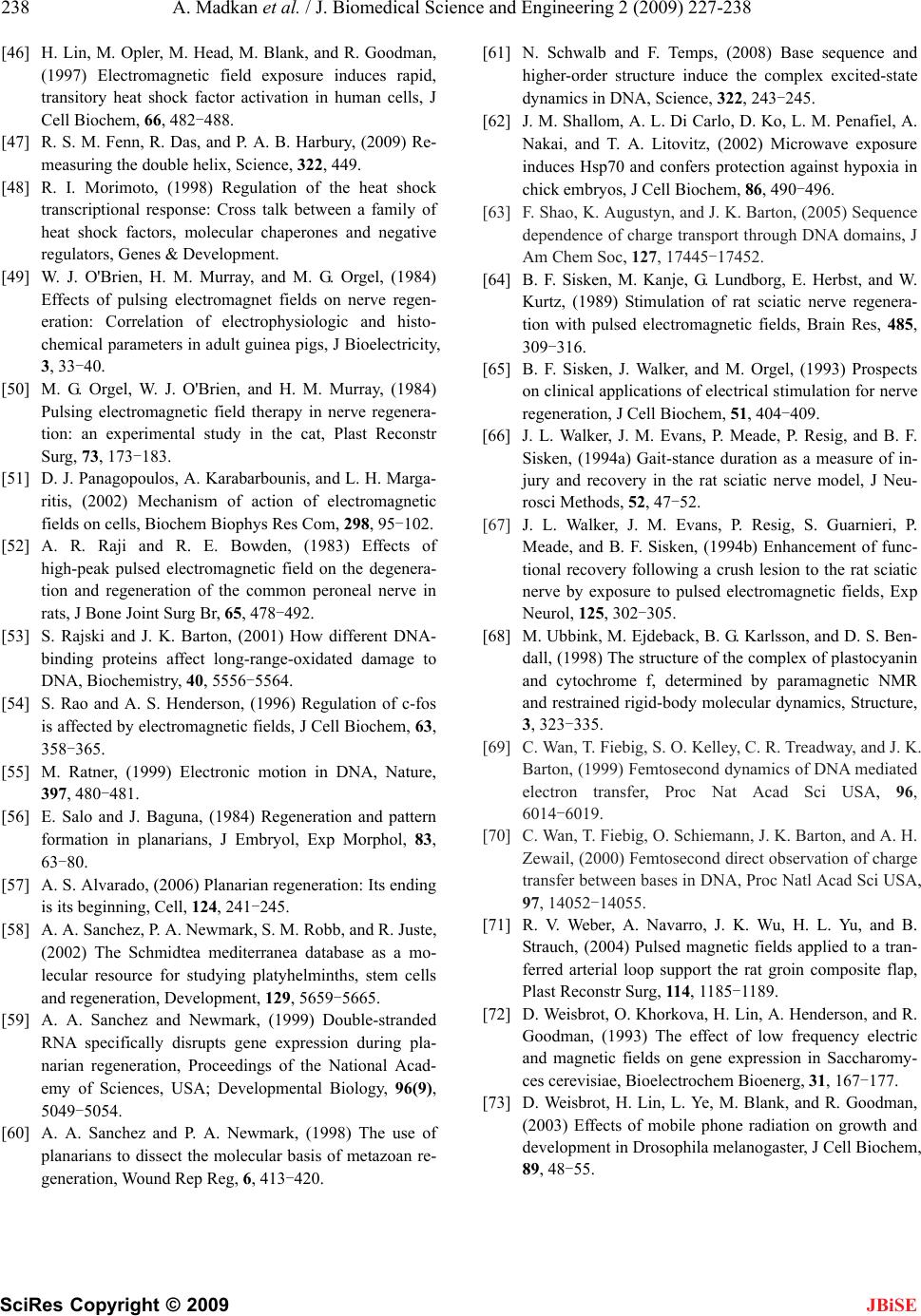 238 A. Madkan et al. / J. Biomedical Science and Engineering 2 (2009) 227-238 SciRes Copyright © 2009 JBiSE [46] H. Lin, M. Opler, M. Head, M. Blank, and R. Goodman, (1997) Electromagnetic field exposure induces rapid, transitory heat shock factor activation in human cells, J Cell Biochem, 66, 482-488. [47] R. S. M. Fenn, R. Das, and P. A. B. Harbury, (2009) Re- measuring the double helix, Science, 322, 449. [48] R. I. Morimoto, (1998) Regulation of the heat shock transcriptional response: Cross talk between a family of heat shock factors, molecular chaperones and negative regulators, Genes & Development. [49] W. J. O'Brien, H. M. Murray, and M. G. Orgel, (1984) Effects of pulsing electromagnet fields on nerve regen- eration: Correlation of electrophysiologic and histo- chemical parameters in adult guinea pigs, J Bioelectricity, 3, 33-40. [50] M. G. Orgel, W. J. O'Brien, and H. M. Murray, (1984) Pulsing electromagnetic field therapy in nerve regenera- tion: an experimental study in the cat, Plast Reconstr Surg, 73, 173-183. [51] D. J. Panagopoulos, A. Karabarbounis, and L. H. Marga- ritis, (2002) Mechanism of action of electromagnetic fields on cells, Biochem Biophys Res Com, 298, 95-102. [52] A. R. Raji and R. E. Bowden, (1983) Effects of high-peak pulsed electromagnetic field on the degenera- tion and regeneration of the common peroneal nerve in rats, J Bone Joint Surg Br, 65, 478-492. [53] S. Rajski and J. K. Barton, (2001) How different DNA- binding proteins affect long-range-oxidated damage to DNA, Biochemistry, 40, 5556-5564. [54] S. Rao and A. S. Henderson, (1996) Regulation of c-fos is affected by electromagnetic fields, J Cell Biochem, 63, 358-365. [55] M. Ratner, (1999) Electronic motion in DNA, Nature, 397, 480-481. [56] E. Salo and J. Baguna, (1984) Regeneration and pattern formation in planarians, J Embryol, Exp Morphol, 83, 63-80. [57] A. S. Alvarado, (2006) Planarian regeneration: Its ending is its beginning, Cell, 124, 241-245. [58] A. A. Sanchez, P. A. Newmark, S. M. Robb, and R. Juste, (2002) The Schmidtea mediterranea database as a mo- lecular resource for studying platyhelminths, stem cells and regeneration, Development, 129, 5659-5665. [59] A. A. Sanchez and Newmark, (1999) Double-stranded RNA specifically disrupts gene expression during pla- narian regeneration, Proceedings of the National Acad- emy of Sciences, USA; Developmental Biology, 96(9), 5049-5054. [60] A. A. Sanchez and P. A. Newmark, (1998) The use of planarians to dissect the molecular basis of metazoan re- generation, Wound Rep Reg, 6, 413-420. [61] N. Schwalb and F. Temps, (2008) Base sequence and higher-order structure induce the complex excited-state dynamics in DNA, Science, 322, 243-245. [62] J. M. Shallom, A. L. Di Carlo, D. Ko, L. M. Penafiel, A. Nakai, and T. A. Litovitz, (2002) Microwave exposure induces Hsp70 and confers protection against hypoxia in chick embryos, J Cell Biochem, 86, 490-496. [63] F. Shao, K. Augustyn, and J. K. Barton, (2005) Sequence dependence of charge transport through DNA domains, J Am Chem Soc, 127, 17445-17452. [64] B. F. Sisken, M. Kanje, G. Lundborg, E. Herbst, and W. Kurtz, (1989) Stimulation of rat sciatic nerve regenera- tion with pulsed electromagnetic fields, Brain Res, 485, 309-316. [65] B. F. Sisken, J. Walker, and M. Orgel, (1993) Prospects on clinical applications of electrical stimulation for nerve regeneration, J Cell Biochem, 51, 404-409. [66] J. L. Walker, J. M. Evans, P. Meade, P. Resig, and B. F. Sisken, (1994a) Gait-stance duration as a measure of in- jury and recovery in the rat sciatic nerve model, J Neu- rosci Methods, 52, 47-52. [67] J. L. Walker, J. M. Evans, P. Resig, S. Guarnieri, P. Meade, and B. F. Sisken, (1994b) Enhancement of func- tional recovery following a crush lesion to the rat sciatic nerve by exposure to pulsed electromagnetic fields, Exp Neurol, 125, 302-305. [68] M. Ubbink, M. Ejdeback, B. G. Karlsson, and D. S. Ben- dall, (1998) The structure of the complex of plastocyanin and cytochrome f, determined by paramagnetic NMR and restrained rigid-body molecular dynamics, Structure, 3, 323-335. [69] C. Wan, T. Fiebig, S. O. Kelley, C. R. Treadway, and J. K. Barton, (1999) Femtosecond dynamics of DNA mediated electron transfer, Proc Nat Acad Sci USA, 96, 6014-6019. [70] C. Wan, T. Fiebig, O. Schiemann, J. K. Barton, and A. H. Zewail, (2000) Femtosecond direct observation of charge transfer between bases in DNA, Proc Natl Acad Sci USA, 97, 14052-14055. [71] R. V. Weber, A. Navarro, J. K. Wu, H. L. Yu, and B. Strauch, (2004) Pulsed magnetic fields applied to a tran- ferred arterial loop support the rat groin composite flap, Plast Reconstr Surg, 114, 1185-1189. [72] D. Weisbrot, O. Khorkova, H. Lin, A. Henderson, and R. Goodman, (1993) The effect of low frequency electric and magnetic fields on gene expression in Saccharomy- ces cerevisiae, Bioelectrochem Bioenerg, 31, 167-177. [73] D. Weisbrot, H. Lin, L. Ye, M. Blank, and R. Goodman, (2003) Effects of mobile phone radiation on growth and development in Drosophila melanogaster, J Cell Biochem, 89, 48-55.
|