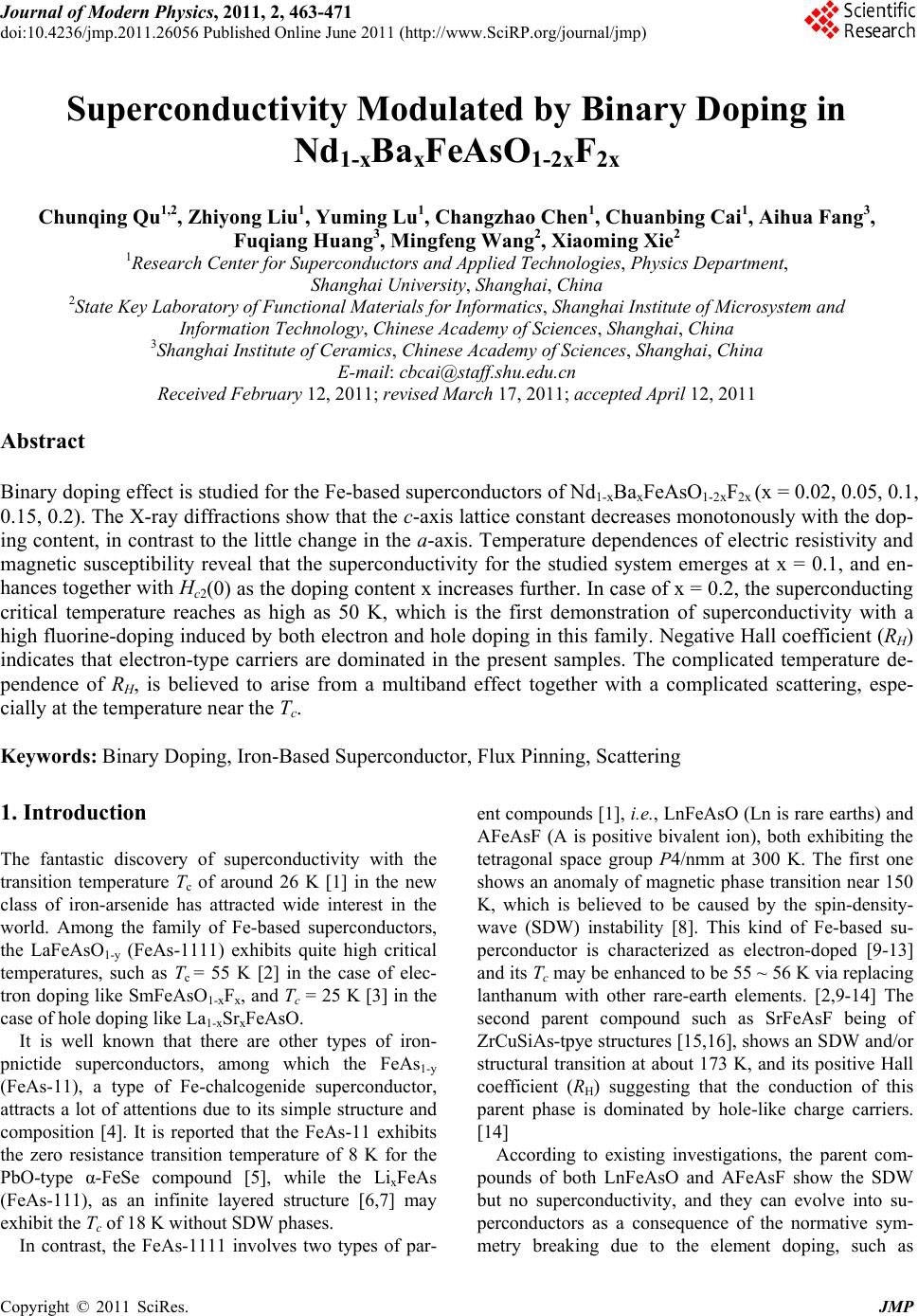 Journal of Modern Physics, 2011, 2, 463-471 doi:10.4236/jmp.2011.26056 Published Online June 2011 (http://www.SciRP.org/journal/jmp) Copyright © 2011 SciRes. JMP Superconductivity Modulated by Binary Doping in Nd1-xBaxFeAsO1-2xF2x Chunqing Qu1,2, Zhiyong Liu1, Yuming Lu1, Changzhao Chen1, Chuanbing Cai1, Aihua Fang3, Fuqiang Huang3, Mingfeng Wang2, Xiaoming Xie2 1Research Center for Superc ond ucto rs an d A ppl i ed Tec hnologies, Physics Department, Shanghai University , Shanghai, China 2State Key Laboratory of Functional Materials for Informatics, Shanghai Institute of Microsystem and Information Technology, Chinese Academy of Sciences, Shanghai, China 3Shanghai Institu te of Ceramics, Chinese Academy of Sciences, Shanghai, China E-mail: cbcai@staff.shu.edu.cn Received February 12, 2011; revised March 17, 2011; accepted April 12, 2011 Abstract Binary doping effect is studied for the Fe-based superconductors of Nd1-xBaxFeAsO1-2xF2x (x = 0.02, 0.05, 0.1, 0.15, 0.2). The X-ray diffractions show that the c-axis lattice constant decreases monotonously with the dop- ing content, in contrast to the little change in the a-axis. Temperature dependences of electric resistivity and magnetic susceptibility reveal that the superconductivity for the studied system emerges at x = 0.1, and en- hances together with Hc2(0) as the doping content x increases further. In case of x = 0.2, the superconducting critical temperature reaches as high as 50 K, which is the first demonstration of superconductivity with a high fluorine-doping induced by both electron and hole doping in this family. Negative Hall coefficient (RH) indicates that electron-type carriers are dominated in the present samples. The complicated temperature de- pendence of RH, is believed to arise from a multiband effect together with a complicated scattering, espe- cially at the temperature near the Tc. Keywords: Binary Doping, Iron-Based Superconductor, Flux Pinning, Scattering 1. Introduction The fantastic discovery of superconductivity with the transition temperature Tc of around 26 K [1] in the new class of iron-arsenide has attracted wide interest in the world. Among the family of Fe-based superconductors, the LaFeAsO1-y (FeAs-1111) exhibits quite high critical temperatures, such as Tc = 55 K [2] in the case of elec- tron doping like SmFeAsO1-xFx, and Tc = 25 K [3] in the case of hole doping like La1-xSrxFeAsO. It is well known that there are other types of iron- pnictide superconductors, among which the FeAs1-y (FeAs-11), a type of Fe-chalcogenide superconductor, attracts a lot of attentions due to its simple structure and composition [4]. It is reported that the FeAs-11 exhibits the zero resistance transition temperature of 8 K for the PbO-type α-FeSe compound [5], while the LixFeAs (FeAs-111), as an infinite layered structure [6,7] may exhibit the Tc of 18 K without SDW phases. In contrast, the FeAs-1111 involves two types of par- ent compounds [1], i.e., LnFeAsO (Ln is rare earths) and AFeAsF (A is positive bivalent ion), both exhibiting the tetragonal space group P4/nmm at 300 K. The first one shows an anomaly of magnetic phase transition near 150 K, which is believed to be caused by the spin-density- wave (SDW) instability [8]. This kind of Fe-based su- perconductor is characterized as electron-doped [9-13] and its Tc may be enhanced to be 55 ~ 56 K via replacing lanthanum with other rare-earth elements. [2,9-14] The second parent compound such as SrFeAsF being of ZrCuSiAs-tpye structures [15,16], shows an SDW and/or structural transition at about 173 K, and its positive Hall coefficient (RH) suggesting that the conduction of this parent phase is dominated by hole-like charge carriers. [14] According to existing investigations, the parent com- pounds of both LnFeAsO and AFeAsF show the SDW but no superconductivity, and they can evolve into su- perconductors as a consequence of the normative sym- metry breaking due to the element doping, such as
 C. Q. QU ET AL. 464 . Gd1-xThxFeAsO [13] SmFe0.9Co0.1AsO [17], CeFeAs1-x PxO [18], and LaFeAsO1-xFx [1]. Similar phenomena also exists in FeAs-122 system, such as Ca1-xNaxFe2As2 [19], Ba(Fe0.961Ru0.039)2As2 [20], EuFe2(As0.7P0.3)2 [21] and so on. With respect to the FeAs-1111 system, the fluorine doping or oxygen deficiency related to the oxygen-site is frequently studied [22,23], disclosing that they are effec- tive to produce the superconductivity. For FeAs-11 sys- tem, it is reported that the clean superconducting phase exists only in those samples with intentional Se defi- ciency [24,25], and single crystal samples are hardly ob- tained with a stoichiometric composition of 1:1. All of these suggest that the superconductivity may emerge or be enhanced by element substitution due to normative symmetry breaking in electronic structure, charge or stress etc. Apart from one element or one site substitution, it is also reported that the second element doping at other site is applicable to modify the normative symmetry and then the superconductivity [26-28], such as Ce1−xGdx FeAsO0.84F0.16 [26], and SmFe1−xRuxAsO0.85F0.15 [27]. Compared with the case of one site doping, two ele- ment substitutions or doping may exhibit more unique phenomenon. In the present paper, we focus on the FeAs-1111 system and study its doping effect using bi- nary substitution, namely Nd1-xBaxFeAsO1-2xF2x. This spe- cial system with both electron-doping and hole-doping allow more breaking of original symmetry, and this may give rise to complicate modification for superconducting and magnetic behaviors. In the flowing sections, the structural and electromagnetic characteristics regarding this system are systemically investigated, to understand its distinct performances and underlying mechanism due to binary doping. 2. Experimental Polycrystalline bulks with nominal compositions of Nd1-xBaxFeAsO1-2xF2x (x = 0.02, 0.05, 0.1, 0.15, and 0.2) were synthesized by conventional solid-state reaction. Firstly, the intermediate phases of Fe2As, NdAs and FeO were prepared by the reactions of the mixed commercial powders of Nd (99.5%), Fe (99.9%), As (99.999%) and Fe2O3 (99.9%), sealed in a vacuum quartz tube, annealed at 600˚C for 6 h and then 850˚C for 12 h. Subsequently, the resultant precursors together with BaF2 powder in stoichiometry of Nd1-xBaxFeAsO1-2xF2x were ground for 15 min. All the weighing and mixing procedures were performed in a glove box filled with high purity Ar gas. After that, the mixtures pressed to into pellets in diameter of 8mm, were sealed in a vacuum quartz tube, and then heated up to 1160˚C by a rate of 230˚C/h. For the reference, the pellets are divided into two sorts, with and without surface wrapping by Ta pieces [29] to test the effect of preventing fluorine-vola- tilizing during the solid-state reaction. Note that the present precursors with and without Ta wrapping were maintained at the temperature of 1160˚C for 10 h only, rather than several ten hours reported by others. This is purposely selected as we believe that it is critical to shorten the heating time to avoid the fluo- rine-volatilizing at high temperatures, and to increase the synthesis efficiency as well. The crystal structure and phase purity of all studied samples were characterized by X-ray diffraction (XRD). The superconducting properties were measured by using a physical property measurement system (Quantum De- sign PPMS) in magnetic fields up to 9 T. Magnetic and transport performances are evaluated by a series of char- acteristic curves including the temperature dependences of resistivity, dc susceptibility, and the Hall coefficient etc. For the magnetization measurements, rectangular specimens were prepared nearly in the same dimension of about 6 × 2 × 1 mm3, and the applied magnetic fields were normal to the long axis direction of the specimen. 3. Result and Discussion 3.1. Microstructure, Lattice Constants and Their Doping Dependences The XRD patterns for the samples with various doping contents of 0.02 - 0.2 are shown in Figure 1. It is clear that the main peaks for all studied samples can be in- dexed to the FeAs-1111 phase with the tetragonal ZrCuSiAs-type structure, regardless of the slight shift of main peaks and several weak peaks corresponding with the impurity phase of NdOF The doping dependence of the lattice constants calcu- lated from the XRD data are presented in Figure 2. There is little change in the lattice constants of a-axis as the doping content increases. This may result from the contrary contributions to the in-plane lattice from two elements doping, since the ionic radius of Ba2+ is larger than that of Nd3+, while the ionic radius of F– is smaller than that of O2–. In contrast, the c-axis lattice constants decrease monotonously with the doping content. Such a reduction probably arises from the decrease in layer dis- tance between FeAs and NdO which may take places due to chemical pressure variation along the c-axis [30]. Note that this is unlike the case of hole-like system such as Pr1-xSrxFeAsO [31] and Nd1-xSrxFeAsO [32], where the c-axis lattices increase monotonously with increasing the doping content of Sr. It is thus observed that the c-axis lattice increase with the doping content is mostly present Copyright © 2011 SciRes. JMP
 C. Q. QU ET AL. 465 Figure 1. (Colour online) X-ray diffraction patterns for the Nd1-xBaxFeAsO1-2xF2x compounds with various doping con- tents from x = 0.02 (bottom) to x = 0.2 (top). Figure 2. (Colour online) Doping content dependence of a-axis and c-axis lattice constants. in hole-like doping FeAs-1111 system, while c-axis de- crease with the doping mostly in electron-like doping. With respect to the chemical pressure change, it is sup- posed that a further increase in Tc may emerge with more BaF2 chemically doped into the FeAs-1111 system [33]. 3.2. Phase Diagram Regarding Superconductivity and SDW Transition Characteristic transition temperatures are evaluated by both R-T curve and dc susceptibility measurements. Figure 3(a) shows the R-T curves for the samples with various doping contents prepared by solid-state reaction without Ta wrapping. To test the stability of the samples, the R-T curves for all samples are measured twice, i.e., immediately after growing and after five months in air, which show a similar transport behavior, suggesting no obvious performance degradation with time going. The inset of Figure 3(a), shows two R-T curves for the same sample of x = 0.15, measured before and after five months to give a direct comparison. Figure 3(b) shows the superconducting critical tem- perature as well as its transition width, identified from the R-T curves in Figure 3(a). It is revealed that the pre- sent samples exhibit the superconductivity as x is more than 0.1, being as high as 50 K at x = 0.2. Moreover, the superconducting transition width ΔT, determined by the temperatures corresponding to 90% and 10% of the nor- mal-state resistivity, decreases with increasing doping content, being as low as 1.86 K at x = 0.2. (a) (b) Figure 3. (colour online) (a) Temperature dependence of resistivity for the studied samples with x = 0.05, 0.1, 0.15, and 0.2. Inset shows two R-T curves for the same sample of x = 0.15, measured before and after 5 months, respectively; (b) The doping content dependence of Tonset and ΔT (deter- mined by the difference of and . 90% c T10% c T Copyright © 2011 SciRes. JMP
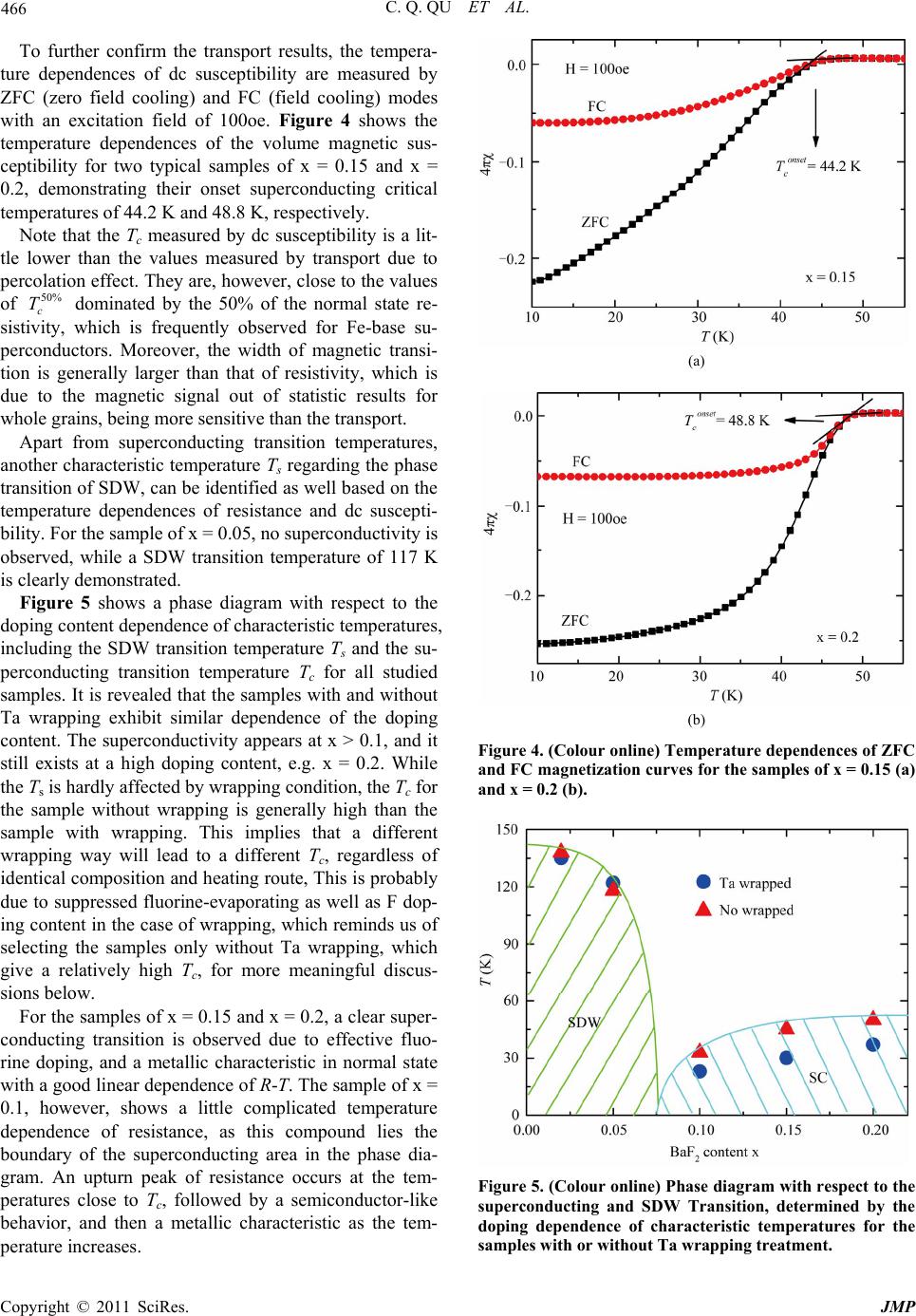 466 C. Q. QU ET AL. To further confirm the transport results, the tempera- ture dependences of dc susceptibility are measured by ZFC (zero field cooling) and FC (field cooling) modes with an excitation field of 100oe. Figure 4 shows the temperature dependences of the volume magnetic sus- ceptibility for two typical samples of x = 0.15 and x = 0.2, demonstrating their onset superconducting critical temperatures of 44.2 K and 48.8 K, respectively. Note that the Tc measured by dc susceptibility is a lit- tle lower than the values measured by transport due to percolation effect. They are, however, close to the values of dominated by the 50% of the normal state re- sistivity, which is frequently observed for Fe-base su- perconductors. Moreover, the width of magnetic transi- tion is generally larger than that of resistivity, which is due to the magnetic signal out of statistic results for whole grains, being more sensitive than the transport. 50% c T Apart from superconducting transition temperatures, another characteristic temperature Ts regarding the phase transition of SDW, can be identified as well based on the temperature dependences of resistance and dc suscepti- bility. For the sample of x = 0.05, no superconductivity is observed, while a SDW transition temperature of 117 K is clearly demonstrated. Figure 5 shows a phase diagram with respect to the doping content dependence of characteristic temperatures, including the SDW transition temperature Ts and the su- perconducting transition temperature Tc for all studied samples. It is revealed that the samples with and without Ta wrapping exhibit similar dependence of the doping content. The superconductivity appears at x > 0.1, and it still exists at a high doping content, e.g. x = 0.2. While the Ts is hardly affected by wrapping condition, the Tc for the sample without wrapping is generally high than the sample with wrapping. This implies that a different wrapping way will lead to a different Tc, regardless of identical composition and heating route, This is probably due to suppressed fluorine-evaporating as well as F dop- ing content in the case of wrapping, which reminds us of selecting the samples only without Ta wrapping, which give a relatively high Tc, for more meaningful discus- sions below. For the samples of x = 0.15 and x = 0.2, a clear super- conducting transition is observed due to effective fluo- rine doping, and a metallic characteristic in normal state with a good linear dependence of R-T. The sample of x = 0.1, however, shows a little complicated temperature dependence of resistance, as this compound lies the boundary of the superconducting area in the phase dia- gram. An upturn peak of resistance occurs at the tem- peratures close to Tc, followed by a semiconductor-like behavior, and then a metallic characteristic as the tem- perature increases. (a) (b) Figure 4. (Colour online) Temperature dependences of ZFC and FC magnetization curves for the samples of x = 0.15 (a) and x = 0.2 (b). Figure 5. (Colour online) Phase diagram with respect to the superconducting and SDW Transition, determined by the doping dependence of characteristic temperatures for the samples with or without Ta wrapping treatment. Copyright © 2011 SciRes. JMP
 C. Q. QU ET AL. 467 Similar behavior was also observed in NdFe1-xRhxAsO [34] and it was assumed that such a semiconductor-like behavior actually was one of natures of this family, which was unambiguously proved by band structure cal- culations as well as photoemission spectroscopy experi- ments [35]. For the present case, as x increases, both the Tc and ΔT are improved due to the increase of BaF2 dop- ing content, and then a more rigid metallic characteristic that eventually hides the semiconductor-like nature at all. This assumption is in consistent with the observation of the residual resistance ratio (RRR = R(300 K)/R(Tc), which is enhanced from 1.93 to 5.5 as the BaF2 doping content of x increases from 0.1 to 0.2. 3.3. Characteristic Magnetic Fields Figure 6 shows the temperature dependences of resistiv- ity under different magnetic fields for the sample of x = 0.15 and 0.2. Based on the magnetotransport measure- ments, two types of characteristic magnetic fields, irre- versibility fields (Hirr) and upper critical fields (Hc2) are (a) (b) Figure 6. (Colour online) Temperature dependences of re- sistance under various magnetic fields for two samples of x = 0.15 (a) and x = 0.2 (b). determined by the criterion of 10% and 90% of nor- mal-state resistivity, respectively. Note that similar evaluation are widely applied to YBaCuO, MgB2, the F-doped LaFeAsO and Sr-doped PrFeAsO polycrystal- line samples [31,36,37]. Figure 7(a) shows the relations between the reduced temperature and characteristic magnetic fields. It is re- vealed that both Hirr and Hc2 are enhanced with increas- ing x from 0.1 to 0.2. In case of x = 0.2, the Hirr(T) curve is closer to Hc2(T) curve, which implies that the stronger flux pinning effect hinders the dissipating resistance due to flux jumping. Figure 7(b) shows the slopes of Hc2(T) near Tc for several superconducting samples with higher doping content of x. By using the Werthamer-Helfand Hohen- berg (WHH) theory, the zero temperature upper critical field Hc2(0) can be evaluated by the formula below, 2 2 d 00.693 dc c cc TT H HT T Taking Tc = 50 K for x = 0.2 sample, one can get the Hc2(0) of 163 T. As well, Hc2(0) is calculated to be 110 T, and 43 T for x = 0.15 and x = 0.1, respectively. Note that these Hc2(0) values are actually lower than that of SmFeAs(OF) [38], and FeAs-42622 family [16]. How- ever, the slope of 2 dd c cT T for the present sample of x = 0.2 is much larger than that of hole doped Pr1-xSrxFeAsO [31], and also than that of electron doped LaFeASO1-xFx) [36,37]. Obviously, the values of upper critical field in the case of the binary doping are much more sensitive than that of single element doping. This suggests that the present system with both electron-doping and hole-doping gives rise to more breaking of original symmetry, and then more modification for supercon- ducting behaviors. A direct mechanism may arise from the increased quasiparticle density of states (DOS) near the Fermi level due to both electron and hole doping. 3.4. Hall Effect and Dominant Carriers To get more information about the conducting carriers, the Hall coefficient (RH) of all samples are measured using the five-probe technique. The deliberated binary doping probably allow more symmetry breaking of the electronic structure, compared with the cases of sole hole doping or electron doping, and this will give rise to the more disorders of internal structure of the compound. Figure 8 shows the temperature dependences of RH for the samples of x = 0.05, 0.10, and 0.20. The negative Hall coefficient RH indicates that the electron-type carri- ers dominate the conduction in the present samples, al- though the binary doping is purposely designed. This is Copyright © 2011 SciRes. JMP
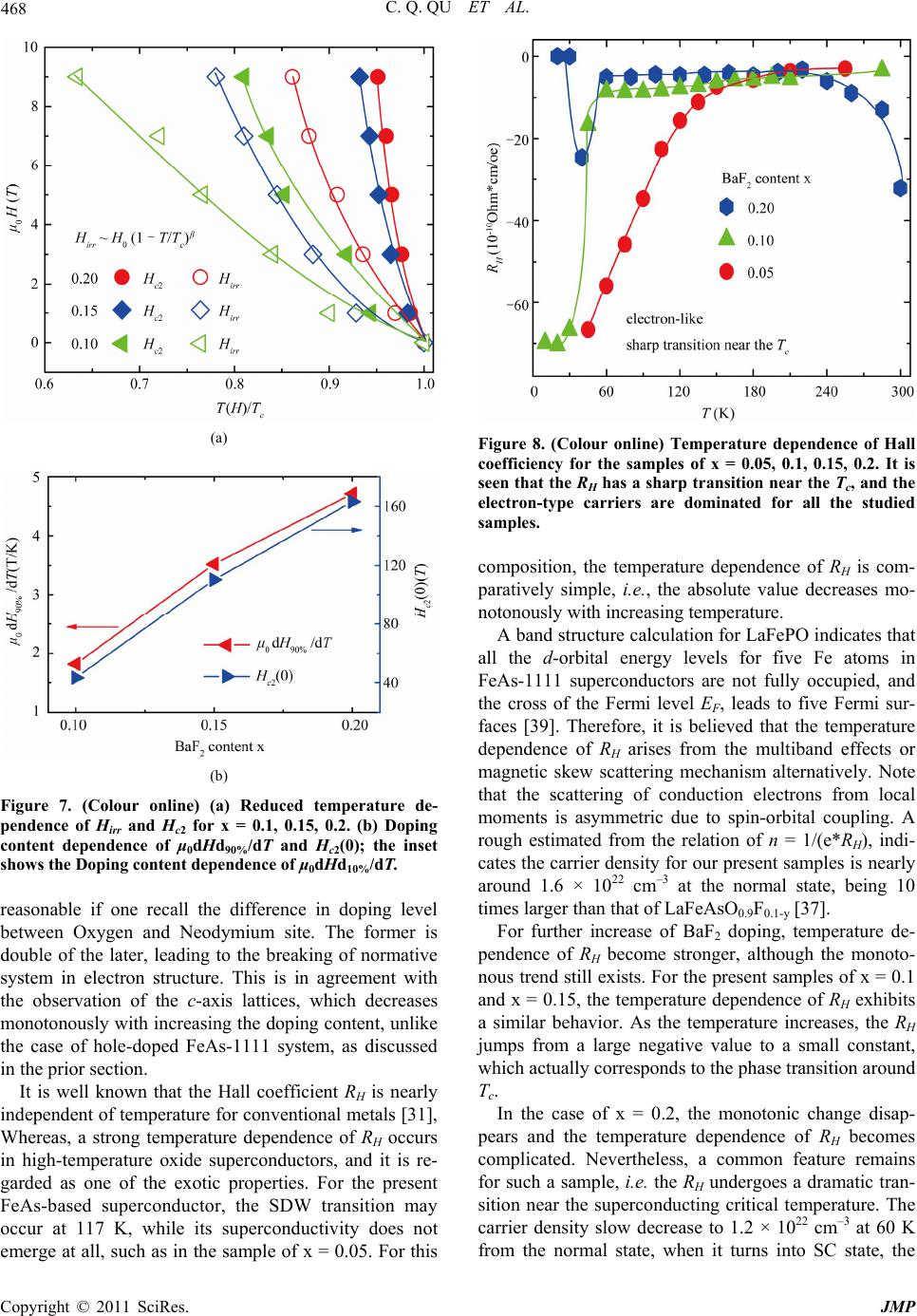 468 C. Q. QU ET AL. (a) (b) Figure 7. (Colour online) (a) Reduced temperature de- pendence of Hirr and Hc2 for x = 0.1, 0.15, 0.2. (b) Doping content dependence of μ0dHd90%/dT and Hc2(0); the inset shows the Doping content dependence of μ0dHd10%/dT. reasonable if one recall the difference in doping level between Oxygen and Neodymium site. The former is double of the later, leading to the breaking of normative system in electron structure. This is in agreement with the observation of the c-axis lattices, which decreases monotonously with increasing the doping content, unlike the case of hole-doped FeAs-1111 system, as discussed in the prior section. It is well known that the Hall coefficient RH is nearly independent of temperature for conventional metals [31], Whereas, a strong temperature dependence of RH occurs in high-temperature oxide superconductors, and it is re- garded as one of the exotic properties. For the present FeAs-based superconductor, the SDW transition may occur at 117 K, while its superconductivity does not emerge at all, such as in the sample of x = 0.05. For this Figure 8. (Colour online) Temperature dependence of Hall coefficiency for the samples of x = 0.05, 0.1, 0.15, 0.2. It is seen that the RH has a sharp transition near the Tc, and the electron-type carriers are dominated for all the studied samples. composition, the temperature dependence of RH is com- paratively simple, i.e., the absolute value decreases mo- notonously with increasing temperature. A band structure calculation for LaFePO indicates that all the d-orbital energy levels for five Fe atoms in FeAs-1111 superconductors are not fully occupied, and the cross of the Fermi level EF, leads to five Fermi sur- faces [39]. Therefore, it is believed that the temperature dependence of RH arises from the multiband effects or magnetic skew scattering mechanism alternatively. Note that the scattering of conduction electrons from local moments is asymmetric due to spin-orbital coupling. A rough estimated from the relation of n = 1/(e*RH), indi- cates the carrier density for our present samples is nearly around 1.6 × 1022 cm–3 at the normal state, being 10 times larger than that of LaFeAsO0.9F0.1-y [37]. For further increase of BaF2 doping, temperature de- pendence of RH become stronger, although the monoto- nous trend still exists. For the present samples of x = 0.1 and x = 0.15, the temperature dependence of RH exhibits a similar behavior. As the temperature increases, the RH jumps from a large negative value to a small constant, which actually corresponds to the phase transition around Tc. In the case of x = 0.2, the monotonic change disap- pears and the temperature dependence of RH becomes complicated. Nevertheless, a common feature remains for such a sample, i.e. the RH undergoes a dramatic tran- sition near the superconducting critical temperature. The carrier density slow decrease to 1.2 × 1022 cm–3 at 60 K from the normal state, when it turns into SC state, the Copyright © 2011 SciRes. JMP
 C. Q. QU ET AL. 469 is system. . Summary mperature near the Tc for such a binary doping stem. . Acknowledgements demy of Sciences are gratefully knowledged as well. . References No. 11, 2008, pp. 3296- value rapid decline to 0.255 × 1022 cm–3at 40 K, while the further low temperature makes the value dramatically shot up to 6.25 × 1024 cm–3. At the temperature below 30 K, the value of RH is close to zero. As the temperature increases, it becomes a large negative value firstly, and then upturns to be a small constant up to 240 K, and fi- nally goes to increase until 300 K. The above temperature dependence appears unusual. It, however, is not hard to understand if one recalls the multi-band effect together with a complicated scattering [3,14,40-42], by which a similar scenario in the hole-like Pr1-xSrxFeAsO [31] can be explained well. It is believed that the present samples follow a strong multi-band effect with a large variation of charge carrier densities as well, especially at the high doping content where the nominal composition of F reaches as high as 40%. These factors suggest that the resultant RH is probably weighted by the sum of the contributions from each band. Assuming that the scattering rate of each band has different temperature dependence, it is reason- able to see the weighted sum change with temperature. In reality, both the effects are able to make collective con- tributions to the Hall signal in the present Nd1-xBax FeAsO1-2xF2x since the electrons are dominant carries in th 4 Effect of binary doping on the compound of Nd1-xBax FeAsO1-2xF2x has been investigated with respect to dis- tinct structural and superconducting properties. It is re- vealed that the c-axis lattice constants decrease monoto- nously with increasing doping content. In case of x > 0.1, the magnetic order of SWD may be destroyed and su- perconductivity takes places, which are confirmed by the measurements of electrical resistivity, and dc susceptibil- ity, and Hall coefficient. The onset superconducting transition temperature can reach 50 K at x = 0.2. The negative Hall coefficient at the studied temperature range suggests that the electron-type charge carriers are domi- nated, regardless of both electron and hole doping pur- posely designed. The complicated temperature depend- ence of RH, is believed to be attributed to a multiband effect together with a complicated scattering, especially at low te sy 5 This work is partly sponsored by the Ministry of Science and Technology of China (973 Projects, No. 2011CBA 00105 and 863 Projects, No. 2009AA03Z204), the Sci- ence and Technology Commission of Shanghai Munici- pality (No. 10dz1203500), and Shanghai Leading Aca- demic Discipline Project (No. S30105). And the supports from the Knowledge Innovation Program of the Chinese Academy of Sciences (Physical Properties and Mecha- nism of Iron based Superconductors), the Open Project of State Key Laboratory of Functional Materials for In- formatics, Chinese Aca ac 6 [1] Y. Kamihara, T. Watanabe, M. Hirano and H. Hosono, “Iron-Based Layered Superconductor La[O1-xFx]FeAs (x = 0.05 - 0.12) with Tc 26 K,” Journal of the American Chemical Society, Vol. 130, 3297. doi:10.1021/ja800073m [2] Z. A. Ren, W. Lu, J. Yang, W. Yi, X. L. Shen, Z. C. Li, G. C. Che, X. L. Dong, L. L. Sun, F. Zhou and Z. X. Zhao, “Superconductivity at 55 K in Iron-Based F-Doped Lay- ered Quaternary Compound Sm[O1-xFx]FeAs, Physics Letters, Vol. 25, No. 6, 200 ” Chinese 8, p. 2215. doi:10.1088/0256-307X/25/6/080 [3] H. H. Wen, G. Mu, L. Fang, H. Yang and X. Y. Zhu, “Superconductivity at 25K in Hole-Doped (La1-xSrx) OFeAs,” Europhysical Letters, Vol. 82, No. 1, 2008, p. 17009. doi:10.1209/0295-5075/82/17009 [4] Y. Mizuguchi, Y. Takano, “A Review of Fe-Chalcogenide Superconductors: The Simplest Fe-Based Superconduc- tor,” Journal of the physical society of Japan, Vol. 79, , 2008, pp. 14262- 2010, p. 102001. [5] F.-C. Hsu, J.-Y. Luo, K.-W. Ye, T.-K. Chen, T.-W. Huang, P. M. Wu, Y.-C. Lee, Y.-L. Huang, Y.-Y. Chu, D.-C. Yan and M.-K. Wu, “Superconductivity in the PbO-Type Structure-FeSe,” Proceedings of the National Academy of Sciences, Vol. 105, No. 38 14264. doi:10.1073/pnas.0807325105 [6] X. C. Wang, Q. Q. Liu, Y. X. Lv, W. B. Gao, L. X. Yang, R. C. Yu, F. Y. Li and C. Q. Jin, “The Superconductivity at 18 K in LiFeAs System,” Solid Sate C Vol. 148, No. 11-12, 2008, pp. ommunications, 538-540. doi:10.1016/j.ssc.2008.09.057 [7] J. H. Tapp, Z. J. Tang, B. Lv, K. Sasmal, B. Lorenz, P. C. W. Chu and A. M. Guloy, “LiFeAs: An Intrinsic FeAs- Based Superconductor with T B, Vol. 78, No. 6, 2008, p. 4. = 18 K,” Physical Review doi:10.1103/PhysRevB.78.060505 [8] J. Dong, H. J. Zhang, G. Xu, Z. Li, W. Z. Hu, D. Wu, G. F. Chen, X. Dai, J. L. Luo, Z. Feng, N. Fang and N. L. Wang, “Competing Orders and Spin-Density-Wave In- stability in La(O1-xFx)FeAs,” Europhysics Letters, Vol. 83, No. 2, 2008, p. 27006. doi:10.1209/0295-5075/83/27006 [9] X. H. Chen, T. Wu, G. Wu, R. H. Liu, H. Chen and D. F. Fang, “Superconductivity at 43K in SmFeA Nature, Vol. 453, No. 7196 sO1-xFx,” , 2008, pp. 761-762. doi:10.1038/nature07045 [10] G. F. Chen, Z. Li, D. Wu, G. Li, W. Z. Hu, J. Dong, P. Copyright © 2011 SciRes. JMP
 C. Q. QU ET AL. 470 ical Review , Vol. 12, Zheng, J. L. Luo, and N. L. Wang, “Superconductivity at 41K and Its Competition with Spin-Density-Wave Instability in Layered CeO1-xFxFeAs,” Phys Letters, Vol. 100, No. 24, 2008, Id: 247002. [11] Z. A. Ren, J. Yang, W. Lu, W. Yi, G.-C. Che, X.-L. Dong, L.-L. Sun and Z.-X. Zhao, “Superconductivity at 52 K in Iron Based F Doped Layered Quaternary Compound PO1-xFxFeAs,” Materials. Research Innovations 2008, p. 105. doi:10.1179/143307508X333686 [12] P. Cheng, L. Fang, H. Yang, X. Y. Zhu, G. Mu, H. Q. Luo, Z. S. Wang and H. H. Wen, “Superconductivity at 36 K in Gadolinium-Arsenide Oxides GdO1- Science China Series G, Vol. 51, 2 xFxFeAs,” 008, p. 719. doi:10.1179/143307508X333686 [13] C. Wang, L. J. Li, S. Chi, Z. W. Zhu, Z. Ren, Y. K. Li, Y. T. Wang, X. Lin, Y. K. Luo, S. Jiang, X. F. Xu, G. H. Cao and Z. A. Xu, “Thorium-Doping–Induced Super- conductivity up to 56K in Gd1-xThxFe Letters, Vol. 83, No. 6, 2008, p. 67 AsO,” Europhys. 006. doi:10.1209/0295-5075/83/67006 [14] F. Han, X. Y. Zhu, G. Mu, P. Cheng and H. H. Wen, “SrFeAsF as a Parent Compound for Iron Pnictide Su- perconductors,” Physical Review B, Vol. 78, No. 18, e- ,” Physical Review B e ysical Re of Physics Physical Re- ical Review Le 2008, Id: 180503(R). [15] H. Kabbour, L. Cario and F. Boucher, “Rational Design Of New Inorganic Compounds with the Zrsicuas Struc- ture Type Using 2D Building Blocks,” Journal of Mat rials Chemistry, Vol. 15, No. 34, 2005, pp. 3525-3531. [16] X. Y. Zhu, F. Han, G. Mu, P. Cheng, B. Zeng and H. H. Wen, “Transition of Stoichiometric Sr VO FeAs to a Su- perconducting State at 37.2 K, Vol. 79, No. 22, 2009, Id: 220512. [17] P. S. Awana, A.Vajpayee, A. Pal, M. Mudgel, R. S. Meena and H. Kishan, “Superconductivity at 14K in th Co-Doped SmFe0.9Co0.1AsO,” arXiv: 0902.3894, 2009. [18] C. de la Cruz, W. Z. Hu, S. L. Li, Q. Huang, J. W. Lynn, M. A. Green, G. F. Chen, N. L. Wang, H. A. Mook, Q. M. Si and P. C. Dai, “Lattice Distortion and Magnetic Quan- tum Phase Transition in CeFeAs1-xPxO,” Phview Letters, Vol. 104, No. 1, 2009, Id: 017204. [19] G. Wu, H. Chen, T. Wu, Y. L. Xie, Y. J. Yan, R. H. Liu, X. F. Wang, J. J. Ying and X. H. Chen, “Differentresis- tivityresponse to Spin-Density Wave and Superconduc- tivity at 20 Kin Ca1-xNaxFe2As2,” Journal Condensed Matter, Vol. 20, 2008, Id: 422201. [20] A. Kreyssig, M. G. Kim, S. Nandi, D. K. Pratt, W. Tian, J. L. Zarestky, N. Ni, A. Thaler, S. L. Bud’ko, P. C. Can- field, R. J. McQueeney and A. I. Goldman, “Suppression of Antiferromagnetic Order and Orthorhombic Distortion in Superconducting Ba(Fe0.961Rh0.039)2As2,” view B, Vol. 81, No. 13, 2010, Id: 134512. [21] Z. Ren, Q. Tao, S. Jiang, C. M. Feng, C. Wang, J. H. Dai, G. H. Cao and Z. A. Xu, “Superconductivity Induced by Phosphorus Doping and Its Coexistence with Ferromag- netism in EuFe2(As0.7P0.3)2,” Phys Vol. 102, No. 13, 2008, p. 137002. tters, doi:10.1103/PhysRevLett.102.137002 [22] J. Yang, X.-L. Shen, W. Lu, W. Yi, Z.-C. Li, Z.-A. Ren, G.-C. Che, X.-L. Dong, L.-L. Sun, F. Zhou and Z.-X. Zhao, “High-Tc Superconductivity in Some Heavy Rare-Earth Iron-Asenide REFeAsO1-y (RE = Ho, Y, Dy and Tb) Compounds,” Group, Vol. 11, No. 2, 2008, p. he Vol. 109, No. 3, 1978, pp. 025005. [23] K. Miyazawa, K. Kihou, P. M. Shirage, C.-H. Lee, H. Kito, H. Eisaki and A. Iyo, “Superconductivity above 50 K in LnFeAsO1y (Ln 1/4 Nd, Sm, Gd, Tb, and Dy) Syn- thesized by High-Pressure Technique,” Journal of t Physical Society of Japan, Vol. 78, 2009, Id: 034712. [24] P. Terzieff and K. L .Komarek, “The Paramagnetic Prop- erties of Iron Selenides with NiAs-Type Structure,” Monatshefte für Chemie, 651-659. doi:10.1007/BF00912781 [25] W. Schuster, H. MiMer and K. L .Komarek, “Transition Metal-Chalcogen Systems, VII.: The Iron-Elenium Phase Diagram,” Monatshefte für Chemie, Vol. 110, No. 5, 1979, pp. 1153-1170. doi:10.1007/BF00910963 [26] J. L. Yang, W. J. Ren, D. Li, W. J. Hu, B. Li and Z. D. Zhang, “Superconductivity Modulated by Internal Pres- sureinCe1-xGdxFeAs O0.84F0.16 Compounds,” Supercon- ductor Science and Technology, Vol. 23, 2010, Id: ,” Physical r Science and Technol- Physical Review B, Vol. 78, No. 5, 2008, Id: ciety of Ja- 06-123706-4. 025003. [27] M. Tropeano, M. R. Cimberle, C. Ferdeghini, G. Lamura, A. Martinelli, A. Palenzona, I. Pallecchi, A. Sala, I. Sheikin, F. Bernardini, M. Monni, S. Massidda and M. Putti, “Isoelectronic Ru Substitution at the Iron Site in SmFe1-xRuxAsO0.85F0.15 and Its Effects on Structural, Superconducting and Normal-State Properties Review B, Vol. 81, No. 18, 2010, Id: 184054. [28] L. Wang, Y. P. Qi, Z. S. Gao, D. L. Wang, X. P. Zhang and Y. W. Ma, “The Roleof Silveraddition on the Struc- tural and Superconducting Properties of Polycrystalline Sr0.6K0.4Fe2As2,” Superconducto ogy, Vol. 23, 2010, Id: 025027. [29] C. Senatore and R. Flukiger, “Upper Critical Fields Well above 100 T for the Superconductor SmFeAsO F with T = 46 K,” 054514. [30] L. E. Klintberg, S. K. Goh, S. Kasahara, Y. Nakai, K. Ishida, M. Sutherland, T. Shibauchi, Y. Matsuda and T. Terashima, “Chemical Pressure and Physical Pressure in BaFe2(As1-xPx)2,” Journal of the Physical So pan, Vol. 79, No. 12, pp. 1237 doi:10.1143/JPSJ.79.123706 [31] G. Mu, B. Zeng, X. Y. Zhu, F. Han, P. Cheng, B. Shen, and H. H. Wen, “Synthesis, Structural, and Transport Proper- ties of the Hole-Doped Superconductor Pr1−xSrxFeAsO,” Chemical Commu- p. 707-709. Physical Review B, Vol. 79, No. 10, 2008. [32] K. Kasperkiewicz, J.-W. G. Bos, A. N. Firch, K. Pras- sides and S. Margadonna, “Structural and Electronic Re- sponse upon Hole Doping of Rare-Earth Iron Oxyarse- nides Nd1−xSrxFeAsO (0 < x ≤ 0.2),” nications, No. 6, 2009, p doi:10.1039/b815830d [33] Y. Mizuguhci, Y. Hara, K. Deguchi, S. Tsuda1, T. Ya- maguchi, K. Takeda, H. Kotegawa, H. Tou and Y. Ta- Copyright © 2011 SciRes. JMP
 C. Q. QU ET AL. Copyright © 2011 SciRes. JMP 471 cience and Technology RhxAsO,” Physical Review B, Vol. 78, No. 18, 2008, nce and Technolog, Vol. 21, No. 10, eview letters, Vol. 101, No. 5, 2008, Id: 8] A. H. Fang, F. Q. Wang and X. M. Xie, “Low-Temperature kano, “Anion Height Dependence of Tc for the Fe-Based Superconductor,” Superco nductor S, 05 Vol. 23, No. 5, 2010, Id: 054013. [34] D. Berardan, L. D. Zhao, L. Pinsard-Gaudart and N. Dragoe, “Electronic Phase Diagram of Fe1-x Physical Review B, Vol. 81, 2010, Id: 094506. [35] A. Koitzsch, D. Inosov, J. Fink, M. Knupfer, H. Eschrig, S. V. Borisenko, G. Behr, A. Köhler, J. Werner, B. Büchner, R. Follath and H. A. Dürr, “Valence-Band and Core-Level Photoemission Spectroscopy of La- FeAsO1-xFx,” Id: 180506. [36] X. Y. zhu, H. Yang, L. Fang, G. Mu and H. H. Wen, “Up- per Criticalfield, Hall Effectand Magnetoresistance Inthe Iron-Based Layeredsuperconductor LaFeAsO0.9F0.1-δ,” Superconductor Scie 2008, ID: 105001. [37] G. F. Chen, Z. Li, G. Li, J. zhou, D. Wu, J. Dong, W. Z. Hu, P. Zheng, Z. J. Chen, H. Q. Yuan, J. Singleton, J. L. Luo and N. L. Wang, “Superconducting Properties of the Fe-Based Layered Superconductor LaFeAsO0.9F0.1-δ,” Physical R 7007. Rapid Synthesis and Superconductivity of Fe-Based Oxypnictide Superconductors,” Journal of the American Chemical Society, Vol. 132, No. 10, 2010. pp. 3260-3261. [3 doi:10.1021/ja100055k [39] S. Lebgue, “Electronic Structure and Properties of the Fermi Surface of the Superconductor LaOFeP,” Physical Review B, Vol. 75, No. 3, 2007, Id: 035110. [40] G. F. Chen, Z. Li, G. Li, W. Z. Hu, J. Dong, J. Zhou, X. D. Zhang, P. Zheng, N. L. Wang and J. L. Luo, “Super- conductivity in Hole-Doped (Sr1-xKx)Fe2As2,” Chinese Physical Letters, Vol. 25, No. 9, 2008, p. 3403. doi:10.1088/0256-307X/25/9/083 [41] J. Q. Yan, A. Kreyssig, S. Nandi, N. Ni, S. L. Bud’ko, A. Kracher, R. J. McQueeney, R. W. McCallum, T. A. Lo- grasso, A. I. Goldman and P. C. Canfield, “Structural Transition and Anisotropic Properties of Single-Crystalline SrFe2As2,” Physical Review B, Vol. 78, No. 2, 2008, Id: 024516. [42] G. F. Chen, Z. Li, J. Dong, G. Li, W. Z. Hu, X. D. Zhang, X. H. Song, P. Zheng, N. L. Wang and J. L. Luo, “Transport and Anisotropy in Single-Crystalline SrFe2As2 and A0.6K0.4Fe2As2 (A = Sr, Ba) Superconductors,” Physical Review B, Vol. 78, No. 22, 2008, Id: 224512.
|