 Journal of Environmental Protection, 2011, 2, 445-453 doi: 10.4236/jep.2011.24051 Published Online June 2011 (http://www.SciRP.org/journal/jep) Copyright © 2011 SciRes. JEP 445 High-Precision GC-MS Analysis of Atmospheric Polycyclic Aromatic Hydrocarbons (PAHs) and Isomer Ratios from Biomass Burning Emissions Prashant Rajput, Manmohan Sarin*, Ramabadran Rengarajan Geosciences-Division, Physical Research Laboratory, Ahmedabad, India. Email: sarin@prl.res.in Received February 19th, 2011; revised March 25th, 2011; accepted April 27th, 2011. ABSTRACT This manuscript describ es an analytical method for the quantita tive determination of 16-polycyclic aromatic hydrocar- bons (PAHs) using accelerated solvent extraction (ASE), followed by purification on a silica cartridge, and sub sequent measurement by gas chromatograph coupled to a mass spectrometer (GC-MS). The solvent extraction parameters (T = 100 ˚C, P = 1500 psi, t = 30 min, V = 30 ml) are optimized with dichloromethan e (DCM) in order to avoid fractiona- tion effect, thereby achieving quantitative mass recovery of PAHs. The purification of PAHs on silica cartridge elimi- nates the matrix effect, facilitates their enrichment from extracted solution and quantitative determination in presence of an internal-standard (Pyrene-D10). The analytical protocol has been successfully used for the quantification of 16-PAHs and their isomer ratios in atmospheric aerosols collected from northern India dominated by agricultural- waste (post-harvest paddy and wheat residue) burning emissions. Based on the analysis of ambient aerosols, collected from different sites, the overall recovery efficiency for 2- to 3-ring PAHs is 85% and near 100% recovery for 4- to 6-ring compounds. Keywords: Agricultural-Waste Burning, PAHs, Accelerated Solvent Extraction, GC-MS 1. Introduction Atmospheric aerosols are composed of mineral dust, in- organic constituents (sulphate and nitrate), carbonaceous matter (organic carbon and elemental carbon) and sea-salts [1-5]. Among the various components, physical adsorp- tion characteristics of mineral dust, sea-salt (polar), and graphitic carbon (non-polar) are well understood [6-8]. These characteristics affect the high precision measure- ments of organic compounds and compromise their ap- plication as proxies to trace the aerosol sources and to understand their chemical reactivity with the atmospheric oxidants (O3, OH and NOx) [9-12]. It is, thus, essential to establish an analytical protocol for the measurements of organic compounds in atmospheric aerosols with varying mass concentration and matrix. Analytical schemes for the quantitative determination of PAHs in environmental samples and standard refer- ence materials are available in the literature e.g. [12-18]. However, suitability of many of these is limited to low aerosol loading. More importantly, these analytical meth- ods have not adequately investigated the matrix effect of tarry matter (emitted from agricultural-waste burning) on mass recovery of PAHs. Therefore, development of an analytical protocol is required for the quantitative mass recovery of PAHs, by eliminating the matrix from high atmospheric loading of aerosols. We report here a quan- titative method for the determination of PAHs by suitable combination of accelerated solvent extraction (ASE), followed by purification on a silica cartridge and subse- quent determination on a gas chromatograph coupled to a mass spectrometer (GC-MS). The suitability of the ana- lytical method has been ascertained from the field-based samples collected from different geographical locations in India. The analyses of organic compounds by the conven- tional extraction techniques such as Soxhlet extraction and ultrasonication [14,19,20], though provide their quantitative recovery, require large volume of solvents (>100 mL) and are often labour intensive. In spite of this, the Soxhlet extraction technique has been successfully used for the extraction of PAHs from standard reference materials (SRM-National Institute of Standards and Tech-
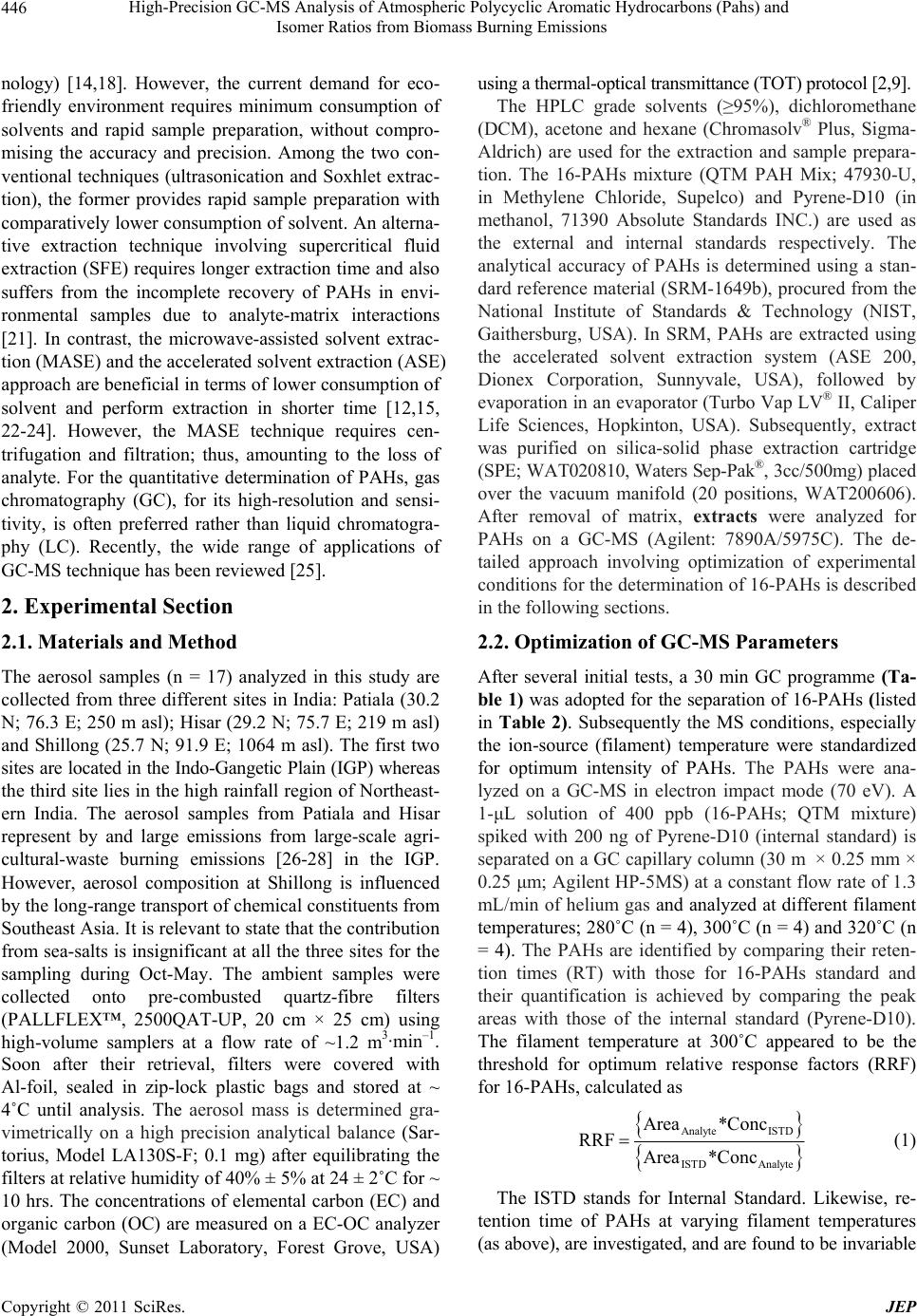 High-Precision GC-MS Analysis of Atmospheric Polycyclic Aromatic Hydrocarbons (Pahs) and 446 Isomer Ratios from Biomass Burning Emissions nology) [14,18]. However, the current demand for eco- friendly environment requires minimum consumption of solvents and rapid sample preparation, without compro- mising the accuracy and precision. Among the two con- ventional techniques (ultrasonication and Soxhlet extrac- tion), the former provides rapid sample preparation with comparatively lower consumption of solvent. An alterna- tive extraction technique involving supercritical fluid extraction (SFE) requires longer extraction time and also suffers from the incomplete recovery of PAHs in envi- ronmental samples due to analyte-matrix interactions [21]. In contrast, the microwave-assisted solvent extrac- tion (MASE) and the accelerated solvent extraction (ASE) approach are beneficial in terms of lower consumption of solvent and perform extraction in shorter time [12,15, 22-24]. However, the MASE technique requires cen- trifugation and filtration; thus, amounting to the loss of analyte. For the quantitative determination of PAHs, gas chromatography (GC), for its high-resolution and sensi- tivity, is often preferred rather than liquid chromatogra- phy (LC). Recently, the wide range of applications of GC-MS technique has been reviewed [25]. 2. Experimental Section 2.1. Materials and Method The aerosol samples (n = 17) analyzed in this study are collected from three different sites in India: Patiala (30.2 N; 76.3 E; 250 m asl); Hisar (29.2 N; 75.7 E; 219 m asl) and Shillong (25.7 N; 91.9 E; 1064 m asl). The first two sites are located in the Indo-Gangetic Plain (IGP) whereas the third site lies in the high rainfall region of Northeast- ern India. The aerosol samples from Patiala and Hisar represent by and large emissions from large-scale agri- cultural-waste burning emissions [26-28] in the IGP. However, aerosol composition at Shillong is influenced by the long-range transport of chemical constituents from Southeast Asia. It is relevant to state that the contribution from sea-salts is insignificant at all the three sites for the sampling during Oct-May. The ambient samples were collected onto pre-combusted quartz-fibre filters (PALLFLEX™, 2500QAT-UP, 20 cm × 25 cm) using high-volume samplers at a flow rate of ~1.2 m3·min–1. Soon after their retrieval, filters were covered with Al-foil, sealed in zip-lock plastic bags and stored at ~ 4˚C until analysis. The aerosol mass is determined gra- vimetrically on a high precision analytical balance (Sar- torius, Model LA130S-F; 0.1 mg) after equilibrating the filters at relative humidity of 40% ± 5% at 24 ± 2˚C for ~ 10 hrs. The concentrations of elemental carbon (EC) and organic carbon (OC) are measured on a EC-OC analyzer (Model 2000, Sunset Laboratory, Forest Grove, USA) using a thermal-optical transmittance (TOT) protocol [2,9]. The HPLC grade solvents (≥95%), dichloromethane (DCM), acetone and hexane (Chromasolv® Plus, Sigma- Aldrich) are used for the extraction and sample prepara- tion. The 16-PAHs mixture (QTM PAH Mix; 47930-U, in Methylene Chloride, Supelco) and Pyrene-D10 (in methanol, 71390 Absolute Standards INC.) are used as the external and internal standards respectively. The analytical accuracy of PAHs is determined using a stan- dard reference material (SRM-1649b), procured from the National Institute of Standards & Technology (NIST, Gaithersburg, USA). In SRM, PAHs are extracted using the accelerated solvent extraction system (ASE 200, Dionex Corporation, Sunnyvale, USA), followed by evaporation in an evaporator (Turbo Vap LV® II, Caliper Life Sciences, Hopkinton, USA). Subsequently, extract was purified on silica-solid phase extraction cartridge (SPE; WAT020810, Waters Sep-Pak®, 3cc/500mg) placed over the vacuum manifold (20 positions, WAT200606). After removal of matrix, extracts were analyzed for PAHs on a GC-MS (Agilent: 7890A/5975C). The de- tailed approach involving optimization of experimental conditions for the determination of 16-PAHs is described in the following sections. 2.2. Optimization of GC-MS Parameters After several initial tests, a 30 min GC programme (Ta- ble 1) was adopted for the separation of 16-PAHs (listed in Table 2). Subsequently the MS conditions, especially the ion-source (filament) temperature were standardized for optimum intensity of PAHs. The PAHs were ana- lyzed on a GC-MS in electron impact mode (70 eV). A 1-μL solution of 400 ppb (16-PAHs; QTM mixture) spiked with 200 ng of Pyrene-D10 (internal standard) is separated on a GC capillary column (30 m × 0.25 mm × 0.25 μm; Agilent HP-5MS) at a constant flow rate of 1.3 mL/min of helium gas and analyzed at different filament temperatures; 280˚C (n = 4), 300˚C (n = 4) and 320˚C (n = 4). The PAHs are identified by comparing their reten- tion times (RT) with those for 16-PAHs standard and their quantification is achieved by comparing the peak areas with those of the internal standard (Pyrene-D10). The filament temperature at 300˚C appeared to be the threshold for optimum relative response factors (RRF) for 16-PAHs, calculated as Analyte ISTD ISTD Analyte Area *Conc RRF Area *Conc (1) The ISTD stands for Internal Standard. Likewise, re- tention time of PAHs at varying filament temperatures (as above), are investigated, and are found to be invariable Copyright © 2011 SciRes. JEP
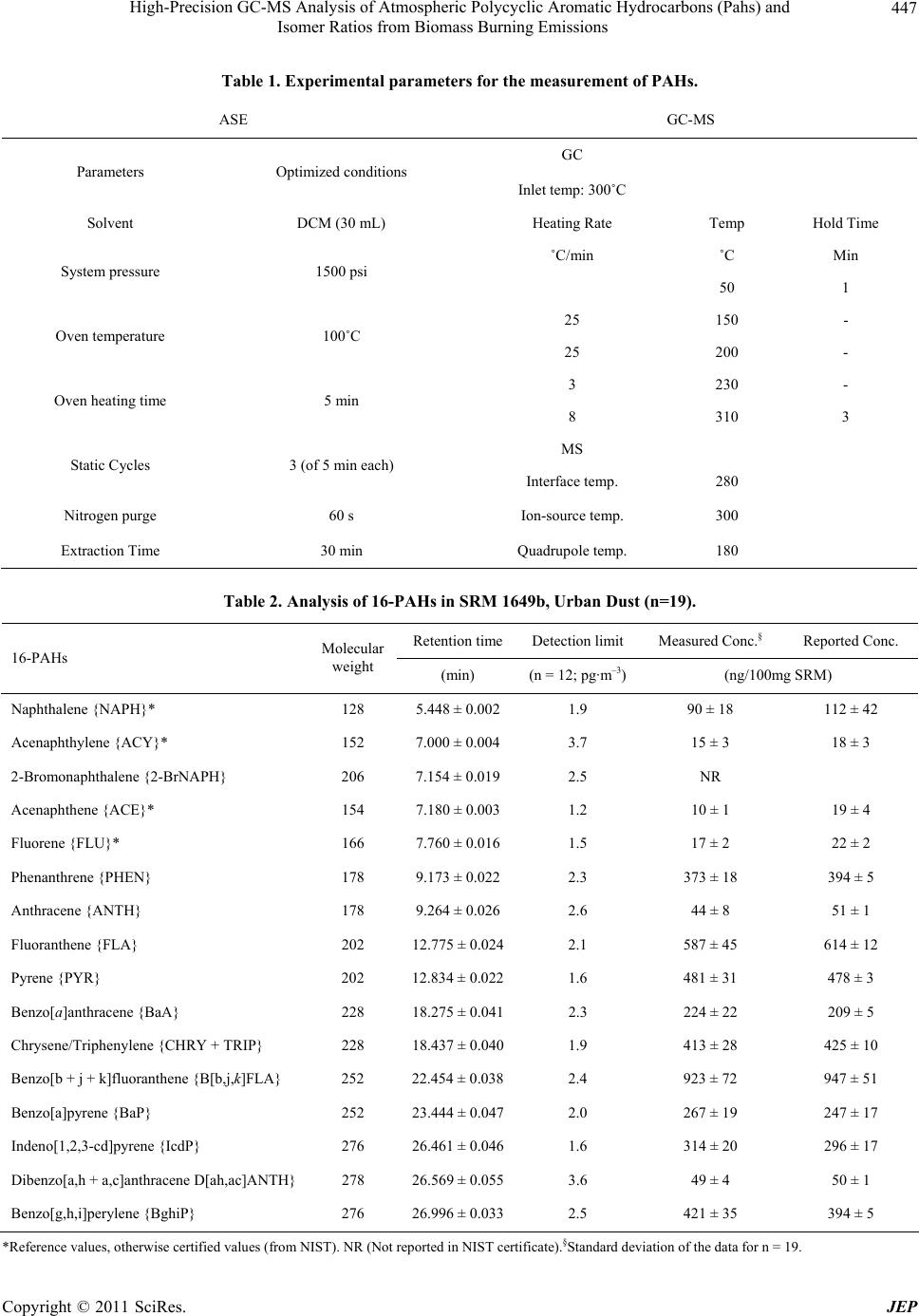 High-Precision GC-MS Analysis of Atmospheric Polycyclic Aromatic Hydrocarbons (Pahs) and 447 Isomer Ratios from Biomass Burning Emissions Table 1. Experimental parameters for the measurement of PAHs. ASE GC-MS GC Parameters Optimized conditions Inlet temp: 300˚C Solvent DCM (30 mL) Heating Rate Temp Hold Time ˚C/min ˚C Min System pressure 1500 psi 50 1 25 150 - Oven temperature 100˚C 25 200 - 3 230 - Oven heating time 5 min 8 310 3 MS Static Cycles 3 (of 5 min each) Interface temp. 280 Nitrogen purge 60 s Ion-source temp. 300 Extraction Time 30 min Quadrupole temp. 180 Table 2. Analysis of 16-PAHs in SRM 1649b, Urban Dust (n=19). Retention timeDetection limitMeasured Conc.§ Reported Conc. 16-PAHs Molecular weight (min) (n = 12; pg·m–3)(ng/100mg SRM) Naphthalene {NAPH}* 128 5.448 ± 0.002 1.9 90 ± 18 112 ± 42 Acenaphthylene {ACY}* 152 7.000 ± 0.004 3.7 15 ± 3 18 ± 3 2-Bromonaphthalene {2-BrNAPH} 206 7.154 ± 0.019 2.5 NR Acenaphthene {ACE}* 154 7.180 ± 0.003 1.2 10 ± 1 19 ± 4 Fluorene {FLU}* 166 7.760 ± 0.016 1.5 17 ± 2 22 ± 2 Phenanthrene {PHEN} 178 9.173 ± 0.022 2.3 373 ± 18 394 ± 5 Anthracene {ANTH} 178 9.264 ± 0.026 2.6 44 ± 8 51 ± 1 Fluoranthene {FLA} 202 12.775 ± 0.0242.1 587 ± 45 614 ± 12 Pyrene {PYR} 202 12.834 ± 0.0221.6 481 ± 31 478 ± 3 Benzo[a]anthracene {BaA} 228 18.275 ± 0.0412.3 224 ± 22 209 ± 5 Chrysene/Triphenylene {CHRY + TRIP} 228 18.437 ± 0.0401.9 413 ± 28 425 ± 10 Benzo[b + j + k]fluoranthene {B[b,j,k]FLA} 252 22.454 ± 0.0382.4 923 ± 72 947 ± 51 Benzo[a]pyrene {BaP} 252 23.444 ± 0.0472.0 267 ± 19 247 ± 17 Indeno[1,2,3-cd]pyrene {IcdP} 276 26.461 ± 0.0461.6 314 ± 20 296 ± 17 Dibenzo[a,h + a,c]anthracene D[ah,ac]ANTH} 278 26.569 ± 0.0553.6 49 ± 4 50 ± 1 Benzo[g,h,i]perylene {BghiP} 276 26.996 ± 0.0332.5 421 ± 35 394 ± 5 Reference values, otherwise certified values (from NIST). NR (Not reported in NIST certificate).§Standard deviation of the data for n = 19. * Copyright © 2011 SciRes. JEP
 High-Precision GC-MS Analysis of Atmospheric Polycyclic Aromatic Hydrocarbons (Pahs) and 448 Isomer Ratios from Biomass Burning Emissions (RT < 0.001% shift). The optimized GC-MS conditions for the determination of PAHs are listed in Table 1, and are used for the measurement of 16-PAHs in ambient aerosols. Data acquisition and processing for the GC-MS analysis is performed on a HP-Enhanced Chemstation Data System. 2.3. Optimization of Purification Step on Silica Cartridge Aerosol samples contain a wide range of matrices in- volving mineral dust, organic carbon and elemental car- bon. These matrices cause mass interferences with the analytes and affect the resolution of measurements, par- ticularly for the measurements of PAHs on GC-MS. In this study, we have used the silica-SPE cartridge for the purification of PAHs [30]. Prior to the application of silica-SPE cartridges for purification of PAHs in aerosol samples, the elution recovery from 16-PAHs standard is investigated . Accordingly, the silica cartridges, installed over the vacuum manifold are conditioned through 10 mL DCM followed by 10 mL hexane under the vacuum (<340 millibar). The cartridges are dried under normal conditions for 5 min. Subsequently, the 16-PAHs liquid mixture of varying amount 100 ng (n = 3), 400 ng (n = 3) and, 800 ng (n = 3), in 3 mL hexane are loaded on silica cartridges. The matrix is allowed to fall under gravity, and is discarded. The cartridges are dried again for 5 min. The affect of cartridge drying on the PAHs mass recov- ery has been discussed elsewhere [30]. Subsequently, the elution of PAHs from each cartridge is performed under gravity with 3 mL, 20% DCM in hexane (v/v). The elu- ate is evaporated to ~ 500 μL under gentle nitrogen gas stream, to which 200 ng of Pyrene-D10 is added. The final solution is made to 1 mL in hexane and stored in amber coloured glass vials at –19˚C until analysis on GC-MS. 2.4. Optimization of Extraction Parameters on ASE The extraction parameters on ASE such as solvent selec- tion, temperature and extraction time on the recovery efficiency of PAHs were investigated at a constant pres- sure of 1500 psi (103 bars). The extraction at 1500 psi pressure is considered to be optimal for the aerosol sam- ples [12]. The extraction protocol was developed based on the analysis of standard reference material (NIST, SRM-1649b, Urban Dust). The toxic solvents e.g. ben- zene and its derivatives were not used to assess the ex- traction efficiency of PAHs. Furthermore, the loss of analyte during sample processing [16], if any, was checked with the low boiling point solvents e.g. DCM (40˚C), which not only extract PAHs quantitatively from aerosols but also can undergo rapid evaporation. Fur- thermore, the PAHs were also extracted from SRM in two different solvents viz. DCM (n = 6) and DCM: Ace- tone (n = 6; 1:1 v/v) at 100˚C, 1500 psi and 3 static cy- cles of 5 min each. These extracts were evaporated to 1 mL in evaporator (<30˚C) and further to near dryness by gentle nitrogen gas purge. The residue was dissolved in 3 mL hexane. Subsequently, the optimized protocol, de- scribed in the previous sections is used for the purifica- tion and sample preparation for PAHs analysis. The re- sults suggest that, within the uncertainty of measure- ments on GC-MS, the yields for individual PAHs were equal with DCM or DCM:Acetone (1:1 v/v). However, DCM was used for the PAHs extraction, due to its rapid evaporation (b.p. 40˚C) in comparison to its mixture with acetone (56.3˚C). The analytical accuracy (Table 2) of the protocol was determined by the SRM analysis (n = 19) following the protocol listed in Table 1. The molecular weight (quantification ion), retention times and the de- tection limits (inferred from analyses of n = 12 blanks) for 16-PAHs are also given in Table 2. 3. Results & Discussion 3.1. Temporal Variations in PM2.5 and Carbonaceous Species (OC, EC) The PM2.5 samples, selected for the evaluation of ana- lytical protocol for PAHs analysis, show temporal vari- ability in aerosol mass from 48 to 391 μg·m–3. The or- ganic and elemental carbon (OC, EC) varied from 15 to 188 μg·m–3 and 2.2 to 18.5 μg·m–3 respectively, whereas Σ PAHs varied from 2 to 46 ng·m–3. The high OC/EC ratios (range: 4 to 19) indicate the dominant contributions of carbonaceous species from biomass burning emissions (agricultural-waste burning and the wood fuel combus- tion) [2,3,31]. 3.2. Sample Preparation for PAHs Analysis The elution recovery of 16-PAHs on silica-SPE cartridge is optimized using 16-PAHs standard, prior to the analy- sis of aerosol samples (Figure 1). The 16-PAHs standard of varying concentrations; 100 ng (n = 3), 400 ng (n = 3) and, 800 ng (n = 3) in 3 mL hexane was eluted from the silica-SPE cartridges. A near quantitative recovery for all 16-PAHs (Figure 1) was achieved with the adopted pro- tocol (as discussed in section 2.3). The assessment of extraction parameters (Figure 2) on ASE suggests that extraction at 100˚C for 30 min (@ 1500 psi) is optimum for the quantification of PAHs in SRM. Moreover, analysis of SRM extracts (n = 12) for different conditions on ASE (tested range: 90˚C - 120˚C; 5 - 15 min static cycle), suggest that though the mass recovery of PAHs Copyright © 2011 SciRes. JEP
 High-Precision GC-MS Analysis of Atmospheric Polycyclic Aromatic Hydrocarbons (Pahs) and Isomer Ratios from Biomass Burning Emissions Copyright © 2011 SciRes. JEP 449 depends on the extraction conditions, their isomers do not fractionate under these conditions on ASE (Figure 2). A one-eighth or one-fourth portion of the quartz filter (based on Total Carbon/ Aerosol Mass) was cut into strips and loaded on the ASE. The PAHs were extracted fol- lowing a developed protocol (Table 1) in 30 mL DCM. Figure 1. Recovery of 16-PAHs after purification on silica cartridge, as ascertained from a standard (QTM PAH Mix; 47930-U, in Methylene Chloride). The consistent recovery of PAHs (~100%) at varying concentrations of standard solution is noteworthy. Figure 2. Replicate analysis (n = 12) of three different isomer pairs in SRM ascertain that accelerated solvent extraction (ASE) does not lead to fractionation of PAHs isomers. The extraction of PAHs at 100 ˚C for 15 min has been used as an optimized tep for quantitative recovery in aerosol samples. s
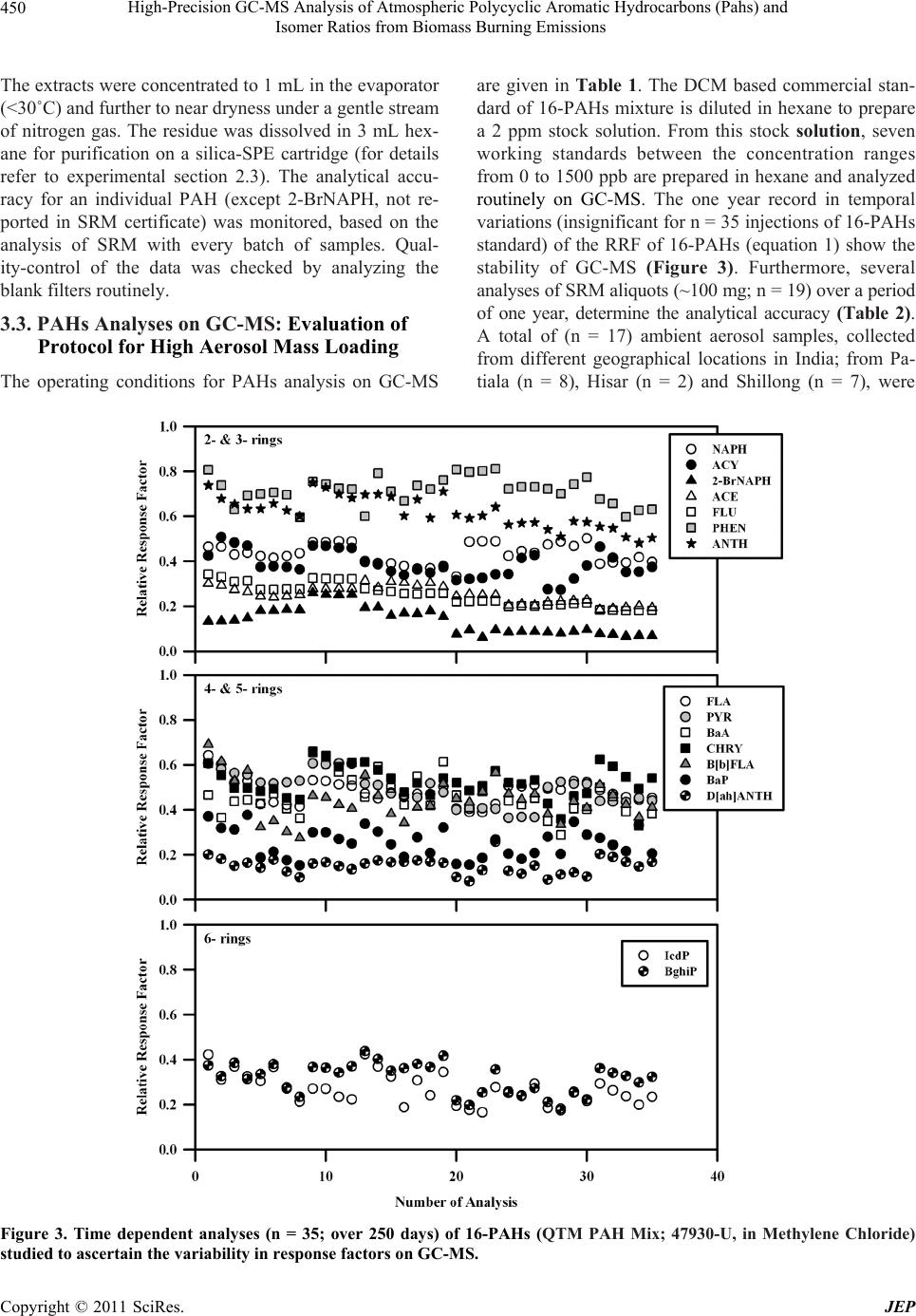 High-Precision GC-MS Analysis of Atmospheric Polycyclic Aromatic Hydrocarbons (Pahs) and 450 Isomer Ratios from Biomass Burning Emissions The extracts were concentrated to 1 mL in the evaporator (<30˚C) and further to near dryness under a gentle stream of nitrogen gas. The residue was dissolved in 3 mL hex- ane for purification on a silica-SPE cartridge (for details refer to experimental section 2.3). The analytical accu- racy for an individual PAH (except 2-BrNAPH, not re- ported in SRM certificate) was monitored, based on the analysis of SRM with every batch of samples. Qual- ity-control of the data was checked by analyzing the blank filters routinely. 3.3. PAHs Analyses on GC-MS: Evaluation of Protocol for High Aerosol Mass Loading The operating conditions for PAHs analysis on GC-MS are given in Table 1. The DCM based commercial stan- dard of 16-PAHs mixture is diluted in hexane to prepare a 2 ppm stock solution. From this stock solution, seven working standards between the concentration ranges from 0 to 1500 ppb are prepared in hexane and analyzed routinely on GC-MS. The one year record in temporal variations (insignificant for n = 35 injections of 16-PAHs standard) of the RRF of 16-PAHs (equation 1) show the stability of GC-MS (Figure 3). Furthermore, several analyses of SRM aliquots (~100 mg; n = 19) over a period of one year, determine the analytical accuracy (Table 2). A total of (n = 17) ambient aerosol samples, collected from different geographical locations in India; from Pa- tiala (n = 8), Hisar (n = 2) and Shillong (n = 7), were Figure 3. Time dependent analyses (n = 35; over 250 days) of 16-PAHs (QTM PAH Mix; 47930-U, in Methylene Chloride) studied to ascertain the variability in response factors on GC-MS. Copyright © 2011 SciRes. JEP
 High-Precision GC-MS Analysis of Atmospheric Polycyclic Aromatic Hydrocarbons (Pahs) and 451 Isomer Ratios from Biomass Burning Emissions analyzed for the mass recovery of PAHs. Repeat extrac- tions and analyses of these samples (n = 17; Figure 4) reveal that the extraction efficiency for 2- to 3-ring PAHs is 85 % whereas the recovery is ~100% for 4- to 6-ring compounds. The overall extraction efficiency of PAHs is 97% ± 2%. The extraction efficiency of PAHs in aerosol samples (Figure 4) is calculated as PAHs recovery in first extraction 100* PAHs recovery infirst+secondextraction (2) Analysis of several sample repeats (n = 17) showed that on an average the external precision of the measurements is ± 4 %. We reemphasis that these samples are repre- sentative of tarry matter and soot (along with the mineral dust), and therefore, the analytical protocol investigate the extraction efficiency of PAHs in the presence of varying matrices. 3.4. Investigation of Loss of 2- to 3- ring PAHs During Sample Processing The 100 ng of 16-PAHs mixture (QTM PAH Mix; 47930-U, in Methylene Chloride, Supelco) was spiked on pre-cleaned quartz fibre filters (1.5 sq cm; n = 6). The extraction of PAHs, followed by matrix purification and sample preparation is done in the similar way to aerosol samples. The analyses on GC-MS ensure recovery close to 100% for the individual PAHs. In contrast to the low recovery for 2- to3-ring PAHs in aerosol samples, the high recovery for all 16-PAHs from spiked filters (~100%), indicate the low concentrations of these PAHs (lighter mass) in aerosol samples lead to their low recovery. 4. Conclusions An analytical method developed for the quantitative de- termination of PAHs from standard reference material (NIST-1649b), show analytical accuracy of (100% ± 15%). The adopted protocol for the quantification of PAHs include ASE extraction with DCM at 100˚C for 3 static cycles (of 5 min each) at a constant pressure of 1500 psi, followed by the matrix purification on a pre-cleaned silica cartridge and subsequent analysis on GC-MS, operated at 300˚C as the optimum ion-source temperature. Analysis of field-based aerosol samples show the average extraction efficiency (equation 2) for 4- to 6- ring PAHs is ~100%. The somewhat lower re- covery (mean ~85%) for 2- to 3- ring PAHs in the field-based samples is attributable to their lower concen- trations in the aerosols. The analytical protocol, for PAHs analysis is ideal to eliminate the matrix effect from tarry matter, soot and mineral dust associated with high atmospheric loading of aerosols. Figure 4. The extraction efficiency of PAHs, as determined in aerosol samples collected from different geographical regions. The 4- to 6- ring PAHs are recovered with ~100 % efficiency, whereas 2- to 3- ring PAHs show somewhat lower recovery (~85%) in the first extraction. Copyright © 2011 SciRes. JEP
 High-Precision GC-MS Analysis of Atmospheric Polycyclic Aromatic Hydrocarbons (Pahs) and 452 Isomer Ratios from Biomass Burning Emissions 5. Acknowledgements We acknowledge the partial financial support from the ISRO-Geosphere Biosphere Programme (Bangaluru, In- dia). REFERENCES [1] O. L. Mayol-Bracero, P. Guyon, B. Graham, G. Roberts, M. O. Andreae, S. Decesari, M. C. Facchini, S. Fuzzi and P. Artaxo, “Water-Soluble Organic Compounds in Bio- mass Burning Aerosols over Amazonia 2. Apportionment of the Chemical Composition and Importance of the Polyacidic Fraction,” Journal of Geophysical Research, Vol. 107, No. D20, 2002. doi:10.1029/2001JD000522 [2] R. Rengarajan, M. M. Sarin and A. K. Sudheer, “Carbo- naceous and Inorganic Species in Atmospheric Aerosols during Wintertime over Urban and High-Altitude Sites in North India,” Journal of Geophysical Research, Vol. 112, No. D21307, 2007. [3] K. Ram and M. M. Sarin, “Spatio-Temporal Variability in Atmospheric Abundances of EC, OC and WSOC over Northern India,” Journal of Aerosol Science, Vol. 41, No. 1, 2010, pp. 88-98. doi:10.1016/j.jaerosci.2009.11.004 [4] J. H. Seinfeld and J. F. Pankow, “Organic Atmospheric Particulate Material,” Annual Review of Physical Chem- istry, Vol., 54, 2003, pp. 121-140. doi:10.1146/annurev.physchem.54.011002.103756 [5] J. H. Seinfeld and S. N. Pandis, “Atmospheric Chemistry and Physics—From Air Pollution to Climate Change,” 2nd Edition, John Wiley & Sons, New York, 2006. [6] S. O. Baek, M. E. Goldstone, P. W. W. Kirk, J. N. Lester and R. Perry, “Phase Distribution and Particle Size De- pendency of Polycyclic Aromatic Hydrocarbons in the Urban Atmosphere,” Chemosphere, Vol. 22, No. 5-6, 1991, pp. 503-520. doi:10.1016/0045-6535(91)90062-I [7] Y. Rudich, N. M. Donahue and T. F. Mentel, “Aging of Organic Aerosol: Bridging the Gap Between Laboratory and Field Studies,” Annual Review of Physical Chemistry, Vol. 58, 2007, pp. 321-352. doi:10.1146/annurev.physchem.58.032806.104432 [8] M. C. Jacobson, H. C. Hansson, K. J. Noone and R. J. Charlson, “Organic Atmospheric Aerosols: Review and State of the Science,” Reviews of Geophysics, Vol. 38, No. 2, 2000, pp. 267-294. doi:10.1029/1998RG000045 [9] E. Perraudin, H. Budzinski and E. Villenave, “Analysis of Polycyclic Aromatic Hydrocarbons Adsorbed on Particles of Atmospheric Interest using Pressurised Fluid Extrac- tion,” Analytical Bioanalytical Chemistry, Vol. 383, No. 1, 2005, pp. 122-131. doi:10.1007/s00216-005-3398-7 [10] K. Miet, K. Le Menach, P. M. Flaud, H. Budzinski and E. Villenave, “Heterogeneous Reactivity of Pyrene and 1-Nitropyrene with NO2: Kinetics, Product Yields and Mechanism,” Atmospheric Environment, Vol. 43, No. 4, 2009, pp. 837-843. doi:10.1016/j.atmosenv.2008.10.041 [11] K. Miet, K. Le Menach, P. M. Flaud, H. Budzinski and E. Villenave, “Heterogeneous Reactions of Ozone with Pyrene, 1-Hydroxypyrene and 1-Nitropyrene Adsorbed on Particles,” Atmospheric Environment, Vol. 43, No. 24, 2009, pp. 3699-3707. doi:10.1016/j.atmosenv.2009.04.032 [12] B. E. Richter, B. A. Jones, J. L. Ezzell, N. L.Porter, N. Avdalovic and C. Pohl, “Accelerated Solvent Extraction: A Technique for Sample Preparation,” Analytical Chem- istry, Vol. 68, No. 6, 1996, pp. 1033-1039. doi:10.1021/ac9508199 [13] G. Kiss, Z. Varga-Puchony and J. Hlavay, “Determination of Polycyclic Aromatic Hydrocarbons in Precipitation using Solid-Phase Extraction and Column Liquid Chro- matography,” Journal of Chromatography A, Vol. 725, No. 2, 1996, pp. 261-272. doi:10.1016/0021-9673(95)00940-X [14] S. A. Wise, L. C. Sander, M. M. Schantz, M. J. Hays and B. A. Benner, “Recertification of Standard Reference Material (SRM) 1649, Urban Dust, for the Determination of Polycyclic Aromatic Hydrocarbons (PAHs),” Poly- cyclic Aromatic Compounds, Vol. 13, No. 4, 2000, pp. 419-456. doi:10.1080/10406630008233854 [15] M. M. Schantz, J. J. Nichols and S. A. Wise, “Evaluation of Pressurized Fluid Extraction for the Extraction of En- vironmental Matrix Reference Materials,” Analytical Chemistry, Vol. 69, No. 20, 1997, pp. 4210-4219. doi:10.1021/ac970299c [16] N. Alexandrou, M. Smith, R. Park, K. Lumb and K. Brice, “The Extraction of Polycyclic Aromatic Hydrocarbons from Atmospheric Particulate Matter Samples by Accel- erated Solvent Extraction (ASE),” International Journal of Environmental Analytical Chemistry, Vol. 81, No. 4, 2001, pp. 257 - 280. doi:10.1080/03067310108044248 [17] G. Kiss, A.Gelencsér, Z. Krivácsy and J. Hlavay, “Oc- currence and Determination of Organic Pollutants in Aerosol, Precipitation, and Sediment Samples Collected at Lake Balaton,” Journal of Chromatography A, Vol. 774, Nos. 1-2, 1997, pp. 349-361. doi:10.1016/S0021-9673(97)00265-3 [18] S. A. Wise, B. A. Benner, S. N. Chesler, L. R. Hilpert, C. R. Vogt and W. E. May, “Characterization of the Poly- cyclic Aromatic Hydrocarbons from Two Standard Ref- erence Material Air Particulate Samples,” Analytical Chemistry, Vol. 58, No. 14, 1986, pp. 3067-3077. doi:10.1021/ac00127a036 [19] J. Duan, X. Bi, J. Tan, G. Sheng and J. Fu, “The Differ- ences of the Size Distribution of Polycyclic Aromatic Hydrocarbons (PAHs) between Urban and Rural Sites of Guangzhou, China,” Atmospheric Research, Vol. 78, Nos. 3-4, 2005, pp. 190-203. doi:10.1016/j.atmosres.2005.04.001 [20] M. Mandalakis, Ö. Gustafsson, T. Alsberg, A.-L. Ege- bäck, C. M. Reddy, L. Xu, J. Klanova, I. Holoubek and E. G. Stephanou, “Contribution of Biomass Burning to At- mospheric Polycyclic Aromatic Hydrocarbons at Three European Background Sites,” Environmental Science & Technology, Vol. 39, No. 9, 2005, pp. 2976-2982. Copyright © 2011 SciRes. JEP
 High-Precision GC-MS Analysis of Atmospheric Polycyclic Aromatic Hydrocarbons (Pahs) and 453 Isomer Ratios from Biomass Burning Emissions doi:10.1021/es048184v [21] S. B. Hawthorne, D. J. Miller, M. D. Burford, J. J. Lan- genfeld, S. Eckert-Tilotta and P. K. Louie, “Factors Con- trolling Quantitative Supercritical Fluid Extraction of En- vironmental Samples,” Journal of Chromatography A, Vol. 642, No. 1-2, 1993, pp. 301-317. doi:10.1016/0021-9673(93)80095-P [22] O. Alvarez-Avilés, L. Cuadra-Rodríguez, F. González- Illán, J. Quiñones-González and O. Rosario, “Optimiza- tion of a Novel Method for the Organic Chemical Char- acterization of Atmospheric Aerosols Based on Micro- wave-Assisted Extraction Combined with Stir Bar Sorp- tive Extraction,” Analytica Chimica Acta, Vol. 597, No. 2, 2007, pp. 273-281. doi:10.1016/j.aca.2007.07.004 [23] K. K. Chee, M. K. Wong and H. K. Lee, “Micro- wave-Assisted Solvent Extraction of Air Particulates for the Determination of PAHs,” Environmental Monitoring and Assessment, Vol. 44, Nos. 1-3, 1997, pp. 391-403. doi:10.1023/A:1005708117992 [24] L. Turrio-Baldassarri, C. L. Battistelli and A. L. Iamiceli, “Evaluation of the Efficiency of Extraction of PAHs from Diesel Particulate Matter with Pressurized Solvents,” Analytical Bioanalytical Chemistry, Vol. 375, No. 4, 2003, pp. 589-595. [25] D. L. Poster, M. M. Schantz, L. C. Sander and S. A. Wise, “Analysis of Polycyclic Aromatic Hydrocarbons (PAHs) in Environmental Samples: A Critical Review of Gas Chromatographic (GC) Methods,” Analytical Bioanalyti- cal Chemistry, Vol. 386, No. 4, 2006, pp. 859-881. doi:10.1007/s00216-006-0771-0 [26] M. Punia, V. P. Nautiyal and Y. Kant, “Identifying Bio- mass Burned Patches of Agricultural Residue using Satel- lite Remote Sensing Data,” Current Science, Vol. 94, No. 9, 2008, pp. 1185-1190. [27] P. K. Gupta, S. Sahai, N. Singh, C. K. Dixit, D. P. Singh, C. Sharma, M. K. Tiwari, R. K. Gupta and S. C. Garg, “Residue Burning in Rice-Wheat Cropping System: Causes and Implications,” Current Science, Vol. 87, No. 12, 2004, pp. 1713-1717. [28] K. V. S. Badarinath, T. R. K. Chand and V. K. Prasad, “Agricultural Crop Residue Burning in the Indo-Gangetic Plains - A Study using IRS-P6 A WiFS Satellite Data,” Current Science, Vol. 91, No. 8, 2006, pp. 1085-1089. [29] J. J. Schauer, B. T. Mader, J. T. DeMinter, G. Heidemann, M. S. Bae, J. H. Seinfeld, R. C. Flagan, R. A. Cary, D. Smith, B. J. Huebert, T. Bertram, S. Howell, J. T. Kline, P. Quinn, T. Bates, B. Turpin, H. J. Lim, J. Z. Yu, H. Yang and M. D. Keywood, “ACE-Asia Intercomparison of a Thermal-Optical Method for the Determination of Particle-Phase Organic and Elemental Carbon,” Envi- ronmental Science & Technology, Vol. 37, No. 5, 2003, pp. 993-1001. doi:10.1021/es020622f [30] M. -X. Xie, F. Xie, Z.-W. Deng and G.-S. Zhuang, “De- termination of Polynuclear Aromatic Hydrocarbons in Aerosol by Solid-Phase Extraction and Gas Chromatog- raphy-Mass Spectrum,” Talanta, Vol. 60, No. 6, 2003, pp. 1245-1257. doi:10.1016/S0039-9140(03)00224-8 [31] K. Ram, M. M. Sarin and P. Hegde, “Atmospheric Abun- dances of Primary and Secondary Carbonaceous Species at Two High-Altitude Sites in India: Sources and Tempo- ral Variability,” Atmospheric Environment, Vol. 42, No. 28, 2008, pp. 6785-6796. doi:10.1016/j.atmosenv.2008.05.031 Copyright © 2011 SciRes. JEP
|