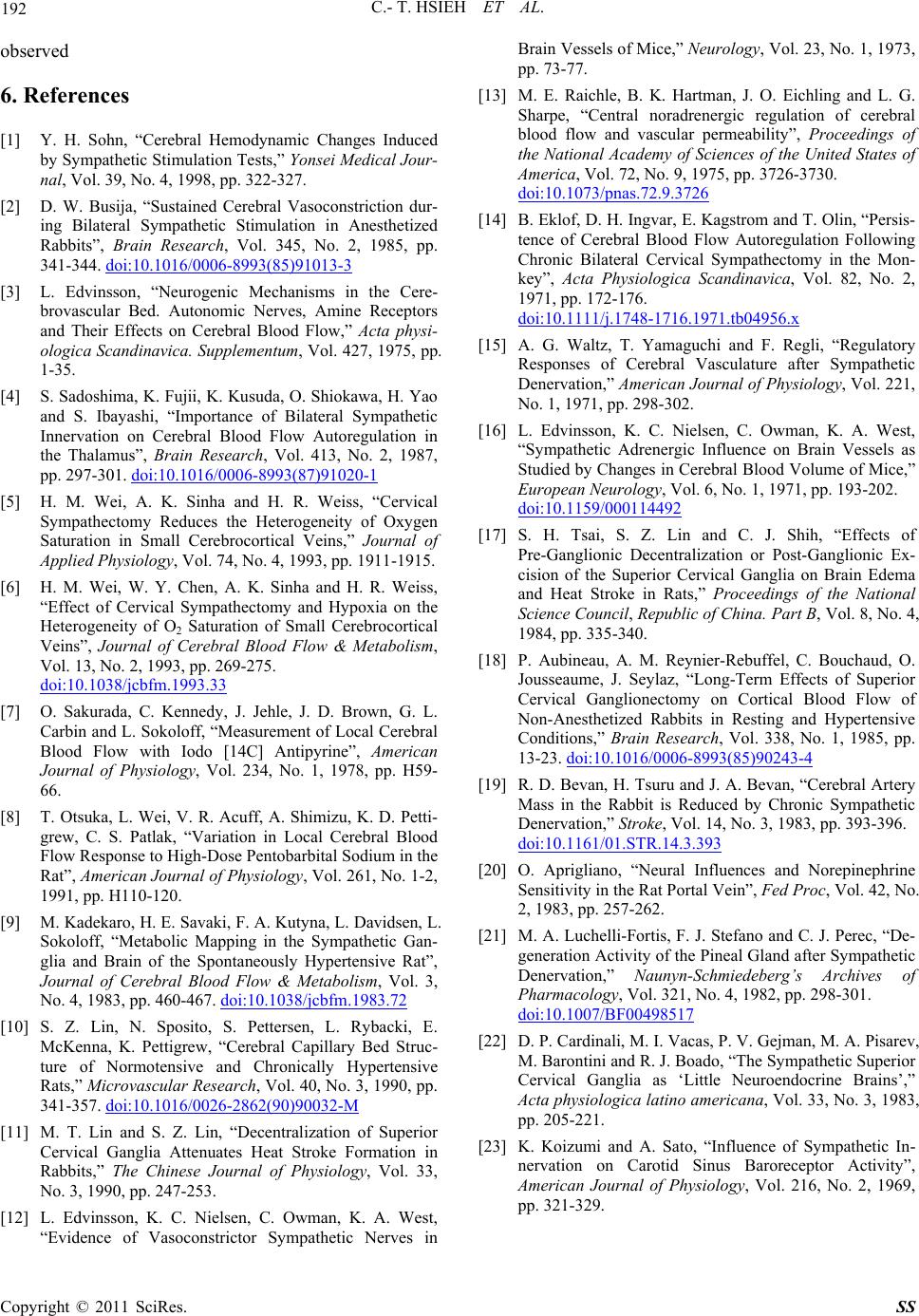
192 C.- T. HSIEH ET AL.
observed
6. References
[1] Y. H. Sohn, “Cerebral Hemodynamic Changes Induced
by Sympathetic Stimulation Tests,” Yonsei Medical Jour-
nal, Vol. 39, No. 4, 1998, pp. 322-327.
[2] D. W. Busija, “Sustained Cerebral Vasoconstriction dur-
ing Bilateral Sympathetic Stimulation in Anesthetized
Rabbits”, Brain Research, Vol. 345, No. 2, 1985, pp.
341-344. doi:10.1016/0006-8993(85)91013-3
[3] L. Edvinsson, “Neurogenic Mechanisms in the Cere-
brovascular Bed. Autonomic Nerves, Amine Receptors
and Their Effects on Cerebral Blood Flow,” Acta physi-
ologica Scandinavica. Supplementum, Vol. 427, 1975, pp.
1-35.
[4] S. Sadoshima, K. Fujii, K. Kusuda, O. Shiokawa, H. Yao
and S. Ibayashi, “Importance of Bilateral Sympathetic
Innervation on Cerebral Blood Flow Autoregulation in
the Thalamus”, Brain Research, Vol. 413, No. 2, 1987,
pp. 297-301. doi:10.1016/0006-8993(87)91020-1
[5] H. M. Wei, A. K. Sinha and H. R. Weiss, “Cervical
Sympathectomy Reduces the Heterogeneity of Oxygen
Saturation in Small Cerebrocortical Veins,” Journal of
Applied Physiology, Vol. 74, No. 4, 1993, pp. 1911-1915.
[6] H. M. Wei, W. Y. Chen, A. K. Sinha and H. R. Weiss,
“Effect of Cervical Sympathectomy and Hypoxia on the
Heterogeneity of O2 Saturation of Small Cerebrocortical
Veins”, Journal of Cerebral Blood Flow & Metabolism,
Vol. 13, No. 2, 1993, pp. 269-275.
doi:10.1038/jcbfm.1993.33
[7] O. Sakurada, C. Kennedy, J. Jehle, J. D. Brown, G. L.
Carbin and L. Sokoloff, “Measurement of Local Cerebral
Blood Flow with Iodo [14C] Antipyrine”, American
Journal of Physiology, Vol. 234, No. 1, 1978, pp. H59-
66.
[8] T. Otsuka, L. Wei, V. R. Acuff, A. Shimizu, K. D. Petti-
grew, C. S. Patlak, “Variation in Local Cerebral Blood
Flow Response to High-Dose Pentobarbital Sodium in the
Rat”, American Journal of Physiology, Vol. 261, No. 1-2,
1991, pp. H110-120.
[9] M. Kadekaro, H. E. Savaki, F. A. Kutyna, L. Davidsen, L.
Sokoloff, “Metabolic Mapping in the Sympathetic Gan-
glia and Brain of the Spontaneously Hypertensive Rat”,
Journal of Cerebral Blood Flow & Metabolism, Vol. 3,
No. 4, 1983, pp. 460-467. doi:10.1038/jcbfm.1983.72
[10] S. Z. Lin, N. Sposito, S. Pettersen, L. Rybacki, E.
McKenna, K. Pettigrew, “Cerebral Capillary Bed Struc-
ture of Normotensive and Chronically Hypertensive
Rats,” Microvascular Research, Vol. 40, No. 3, 1990, pp.
341-357. doi:10.1016/0026-2862(90)90032-M
[11] M. T. Lin and S. Z. Lin, “Decentralization of Superior
Cervical Ganglia Attenuates Heat Stroke Formation in
Rabbits,” The Chinese Journal of Physiology, Vol. 33,
No. 3, 1990, pp. 247-253.
[12] L. Edvinsson, K. C. Nielsen, C. Owman, K. A. West,
“Evidence of Vasoconstrictor Sympathetic Nerves in
Brain Vessels of Mice,” Neurology, Vol. 23, No. 1, 1973,
pp. 73-77.
[13] M. E. Raichle, B. K. Hartman, J. O. Eichling and L. G.
Sharpe, “Central noradrenergic regulation of cerebral
blood flow and vascular permeability”, Proceedings of
the National Academy of Sciences of the United States of
America, Vol. 72, No. 9, 1975, pp. 3726-3730.
doi:10.1073/pnas.72.9.3726
[14] B. Eklof, D. H. Ingvar, E. Kagstrom and T. Olin, “Persis-
tence of Cerebral Blood Flow Autoregulation Following
Chronic Bilateral Cervical Sympathectomy in the Mon-
key”, Acta Physiologica Scandinavica, Vol. 82, No. 2,
1971, pp. 172-176.
doi:10.1111/j.1748-1716.1971.tb04956.x
[15] A. G. Waltz, T. Yamaguchi and F. Regli, “Regulatory
Responses of Cerebral Vasculature after Sympathetic
Denervation,” American Journal of Physiology, Vol. 221,
No. 1, 1971, pp. 298-302.
[16] L. Edvinsson, K. C. Nielsen, C. Owman, K. A. West,
“Sympathetic Adrenergic Influence on Brain Vessels as
Studied by Changes in Cerebral Blood Volume of Mice,”
European Neurology, Vol. 6, No. 1, 1971, pp. 193-202.
doi:10.1159/000114492
[17] S. H. Tsai, S. Z. Lin and C. J. Shih, “Effects of
Pre-Ganglionic Decentralization or Post-Ganglionic Ex-
cision of the Superior Cervical Ganglia on Brain Edema
and Heat Stroke in Rats,” Proceedings of the National
Science Council, Republic of China. Part B, Vol. 8, No. 4,
1984, pp. 335-340.
[18] P. Aubineau, A. M. Reynier-Rebuffel, C. Bouchaud, O.
Jousseaume, J. Seylaz, “Long-Term Effects of Superior
Cervical Ganglionectomy on Cortical Blood Flow of
Non-Anesthetized Rabbits in Resting and Hypertensive
Conditions,” Brain Research, Vol. 338, No. 1, 1985, pp.
13-23. doi:10.1016/0006-8993(85)90243-4
[19] R. D. Bevan, H. Tsuru and J. A. Bevan, “Cerebral Artery
Mass in the Rabbit is Reduced by Chronic Sympathetic
Denervation,” Stroke, Vol. 14, No. 3, 1983, pp. 393-396.
doi:10.1161/01.STR.14.3.393
[20] O. Aprigliano, “Neural Influences and Norepinephrine
Sensitivity in the Rat Portal Vein”, Fed Proc, Vol. 42, No.
2, 1983, pp. 257-262.
[21] M. A. Luchelli-Fortis, F. J. Stefano and C. J. Perec, “De-
generation Activity of the Pineal Gland after Sympathetic
Denervation,” Naunyn-Schmiedeberg’s Archives of
Pharmacology, Vol. 321, No. 4, 1982, pp. 298-301.
doi:10.1007/BF00498517
[22] D. P. Cardinali, M. I. Vacas, P. V. Gejman, M. A. Pisarev,
M. Barontini and R. J. Boado, “The Sympathetic Superior
Cervical Ganglia as ‘Little Neuroendocrine Brains’,”
Acta physiologica latino americana, Vol. 33, No. 3, 1983,
pp. 205-221.
[23] K. Koizumi and A. Sato, “Influence of Sympathetic In-
nervation on Carotid Sinus Baroreceptor Activity”,
American Journal of Physiology, Vol. 216, No. 2, 1969,
pp. 321-329.
Copyright © 2011 SciRes. SS