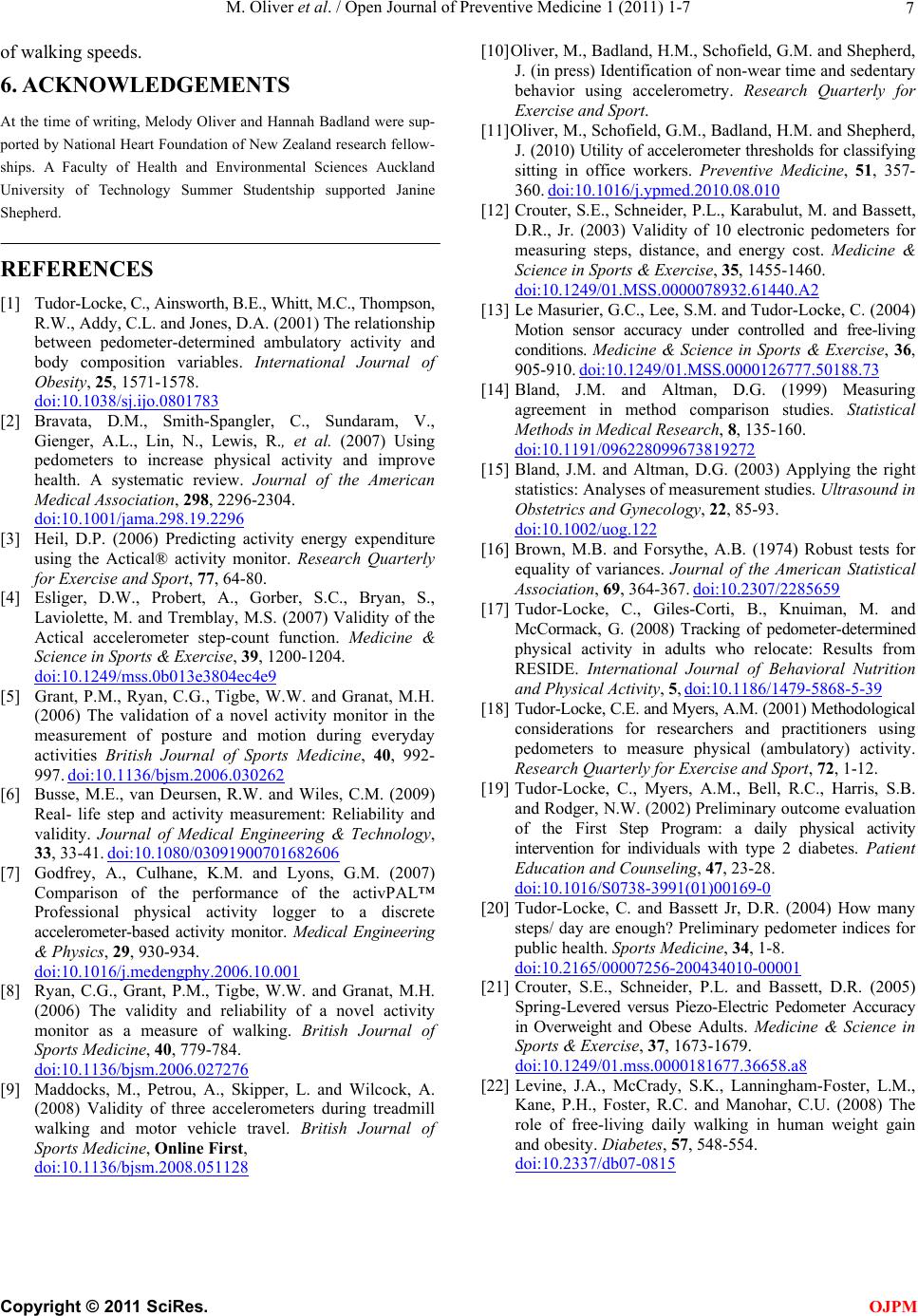
M. Oliver et al. / Open Journal of Preventive Medicine 1 (2011) 1-7
Copyright © 2011 SciRes.
7
[10] Oliver, M., Badland, H.M., Schofield, G.M. and Shepherd,
J. (in press) Identification of non-wear time and sedentary
behavior using accelerometry. Research Quarterly for
Exercise and Sport.
of walking speeds.
6. ACKNOWLEDGEMENTS
At the time of writing, Melody Oliver and Hannah Badland were sup-
ported by National Heart Foundation of New Zealand research fellow-
ships. A Faculty of Health and Environmental Sciences Auckland
University of Technology Summer Studentship supported Janine
Shepherd.
[11]
Oliver, M., Schofield, G.M., Badland, H.M. and Shepherd,
J. (2010) Utility of accelerometer thresholds for classifying
sitting in office workers. Preventive Medicine, 51, 357-
360. doi:10.1016/j.ypmed.2010.08.010
[12] Crouter, S.E., Schneider, P.L., Karabulut, M. and Bassett,
D.R., Jr. (2003) Validity of 10 electronic pedometers for
measuring steps, distance, and energy cost. Medicine &
Science in Sports & Exercise, 35, 1455-1460.
doi:10.1249/01.MSS.0000078932.61440.A2
REFERENCES
[1] Tudor-Locke, C., Ainsworth, B.E., Whitt, M.C., Thompson,
R.W., Addy, C.L. and Jones, D.A. (2001) The relationship
between pedometer-determined ambulatory activity and
body composition variables. International Journal of
Obesity, 25, 1571-1578.
doi:10.1038/sj.ijo.0801783
[13] Le Masurier, G.C., Lee, S.M. and Tudor-Locke, C. (2004)
Motion sensor accuracy under controlled and free-living
conditions. Medicine & Science in Sports & Exercise, 36,
905-910. doi:10.1249/01.MSS.0000126777.50188.73
[14] Bland, J.M. and Altman, D.G. (1999) Measuring
agreement in method comparison studies. Statistical
Methods in Medical Research, 8, 135-160.
doi:10.1191/096228099673819272
[2] Bravata, D.M., Smith-Spangler, C., Sundaram, V.,
Gienger, A.L., Lin, N., Lewis, R., et al. (2007) Using
pedometers to increase physical activity and improve
health. A systematic review. Journal of the American
Medical Association, 298, 2296-2304.
doi:10.1001/jama.298.19.2296
[15] Bland, J.M. and Altman, D.G. (2003) Applying the right
statistics: Analyses of measurement studies. Ultrasound in
Obstetrics and Gynecology, 22, 85-93.
doi:10.1002/uog.122
[3] Heil, D.P. (2006) Predicting activity energy expenditure
using the Actical® activity monitor. Research Quarterly
for Exercise and Sport, 77, 64-80.
[16] Brown, M.B. and Forsythe, A.B. (1974) Robust tests for
equality of variances. Journal of the American Statistical
Association, 69, 364-367. doi:10.2307/2285659
[4] Esliger, D.W., Probert, A., Gorber, S.C., Bryan, S.,
Laviolette, M. and Tremblay, M.S. (2007) Validity of the
Actical accelerometer step-count function. Medicine &
Science in Sports & Exercise, 39, 1200-1204.
doi:10.1249/mss.0b013e3804ec4e9
[17] Tudor-Locke, C., Giles-Corti, B., Knuiman, M. and
McCormack, G. (2008) Tracking of pedometer-determined
physical activity in adults who relocate: Results from
RESIDE. International Journal of Behavioral Nutrition
and Physical Activity, 5, doi:10.1186/1479-5868-5-39
[5] Grant, P.M., Ryan, C.G., Tigbe, W.W. and Granat, M.H.
(2006) The validation of a novel activity monitor in the
measurement of posture and motion during everyday
activities British Journal of Sports Medicine, 40, 992-
997. doi:10.1136/bjsm.2006.030262
[18] Tudor-Locke, C.E. and Myers, A.M. (2001) Methodological
considerations for researchers and practitioners using
pedometers to measure physical (ambulatory) activity.
Research Quarterly for Exercise and Sport, 72, 1-12.
[19] Tudor-Locke, C., Myers, A.M., Bell, R.C., Harris, S.B.
and Rodger, N.W. (2002) Preliminary outcome evaluation
of the First Step Program: a daily physical activity
intervention for individuals with type 2 diabetes. Patient
Education and Counseling, 47, 23-28.
doi:10.1016/S0738-3991(01)00169-0
[6] Busse, M.E., van Deursen, R.W. and Wiles, C.M. (2009)
Real- life step and activity measurement: Reliability and
validity. Journal of Medical Engineering & Technology,
33, 33-41. doi:10.1080/03091900701682606
[7] Godfrey, A., Culhane, K.M. and Lyons, G.M. (2007)
Comparison of the performance of the activPAL™
Professional physical activity logger to a discrete
accelerometer-based activity monitor. Medical Engineering
& Physics, 29, 930-934.
doi:10.1016/j.medengphy.2006.10.001
[20] Tudor-Locke, C. and Bassett Jr, D.R. (2004) How many
steps/ day are enough? Preliminary pedometer indices for
public health. Sports Medicine, 34, 1-8.
doi:10.2165/00007256-200434010-00001
[21] Crouter, S.E., Schneider, P.L. and Bassett, D.R. (2005)
Spring-Levered versus Piezo-Electric Pedometer Accuracy
in Overweight and Obese Adults. Medicine & Science in
Sports & Exercise, 37, 1673-1679.
doi:10.1249/01.mss.0000181677.36658.a8
[8] Ryan, C.G., Grant, P.M., Tigbe, W.W. and Granat, M.H.
(2006) The validity and reliability of a novel activity
monitor as a measure of walking. British Journal of
Sports Medicine, 40, 779-784.
doi:10.1136/bjsm.2006.027276 [22] Levine, J.A., McCrady, S.K., Lanningham-Foster, L.M.,
Kane, P.H., Foster, R.C. and Manohar, C.U. (2008) The
role of free-living daily walking in human weight gain
and obesity. Diabetes, 57, 548-554.
doi:10.2337/db07-0815
[9] Maddocks, M., Petrou, A., Skipper, L. and Wilcock, A.
(2008) Validity of three accelerometers during treadmill
walking and motor vehicle travel. British Journal of
Sports Medicine, Online First,
doi:10.1136/bjsm.2008.051128
OJPM