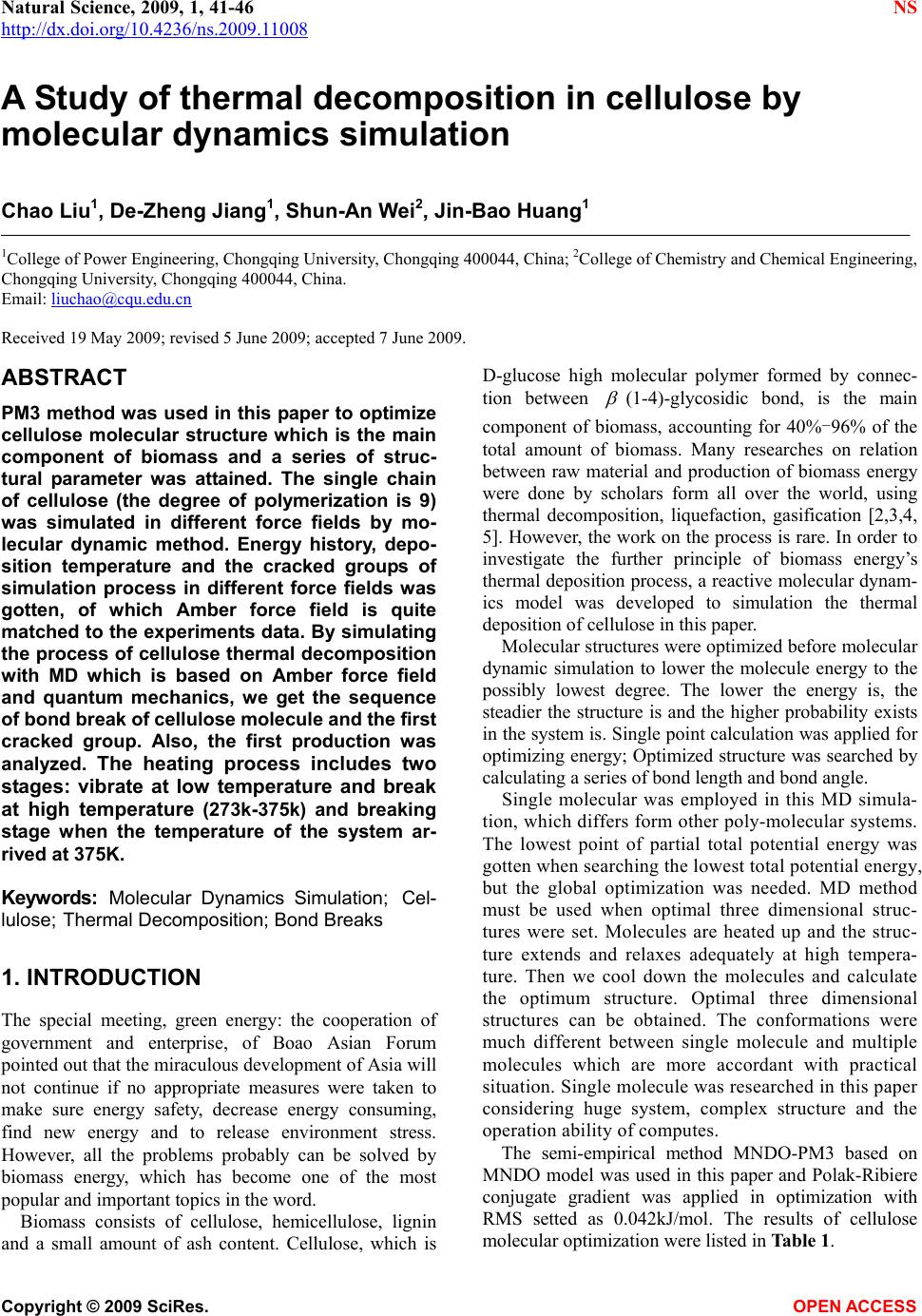
Natural Science, 2009, 1, 41-46 NS
http://dx.doi.org/10.4236/ns.2009.11008
Copyright © 2009 SciRes. OPEN ACCESS
A Study of thermal decomposition in cellulose by
molecular dynamics simulation
Chao Liu1, De-Zheng Jiang1, Shun-An Wei2, Jin-Bao Huang1
1College of Power Engineering, Chongqing University, Chongqing 400044, China; 2College of Chemistry and Chemical Engineering,
Chongqing University, Chongqing 400044, China.
Email: liuchao@cqu.edu.cn
Received 19 May 2009; revised 5 June 2009; accepted 7 June 2009.
ABSTRACT
PM3 method was used in this paper to optimize
cellulose molecular structure which is the main
component of biomass and a series of struc-
tural parameter was attained. The single chain
of cellulose (the degree of polymerization is 9)
was simulated in different force fields by mo-
lecular dynamic method. Energy history, depo-
sition temperature and the cracked groups of
simulation process in different force fields was
gotten, of which Amber force field is quite
matched to the experiments data. By simulating
the process of cellulose thermal decomposition
with MD which is based on Amber force field
and quantum mechanics, we get the sequence
of bond break of cellulose molecule and the first
cracked group. Also, the first production was
analyzed. The heating process includes two
stages: vibrate at low temperature and break
at high temperature (273k-375k) and breaking
stage when the temperature of the system ar-
rived at 375K.
Keywords: Molecular Dynamics Simulation; Cel-
lulose; Thermal Decomposition; Bond Breaks
1. INTRODUCTION
The special meeting, green energy: the cooperation of
government and enterprise, of Boao Asian Forum
pointed out that the miraculous development of Asia will
not continue if no appropriate measures were taken to
make sure energy safety, decrease energy consuming,
find new energy and to release environment stress.
However, all the problems probably can be solved by
biomass energy, which has become one of the most
popular and important topics in the word.
Biomass consists of cellulose, hemicellulose, lignin
and a small amount of ash content. Cellulose, which is
D-glucose high molecular polymer formed by connec-
tion between
(1-4)-glycosidic bond, is the main
component of biomass, accounting for 40%-96% of the
total amount of biomass. Many researches on relation
between raw material and production of biomass energy
were done by scholars form all over the world, using
thermal decomposition, liquefaction, gasification [2,3,4,
5]. However, the work on the process is rare. In order to
investigate the further principle of biomass energy’s
thermal deposition process, a reactive molecular dynam-
ics model was developed to simulation the thermal
deposition of cellulose in this paper.
Molecular structures were optimized before molecular
dynamic simulation to lower the molecule energy to the
possibly lowest degree. The lower the energy is, the
steadier the structure is and the higher probability exists
in the system is. Single point calculation was applied for
optimizing energy; Optimized structure was searched by
calculating a series of bond length and bond angle.
Single molecular was employed in this MD simula-
tion, which differs form other poly-molecular systems.
The lowest point of partial total potential energy was
gotten when searching the lowest total potential energy,
but the global optimization was needed. MD method
must be used when optimal three dimensional struc-
tures were set. Molecules are heated up and the struc-
ture extends and relaxes adequately at high tempera-
ture. Then we cool down the molecules and calculate
the optimum structure. Optimal three dimensional
structures can be obtained. The conformations were
much different between single molecule and multiple
molecules which are more accordant with practical
situation. Single molecule was researched in this paper
considering huge system, complex structure and the
operation ability of computes.
The semi-empirical method MNDO-PM3 based on
MNDO model was used in this paper and Polak-Ribiere
conjugate gradient was applied in optimization with
RMS setted as 0.042kJ/mol. The results of cellulose
molecular optimization were listed in Table 1.