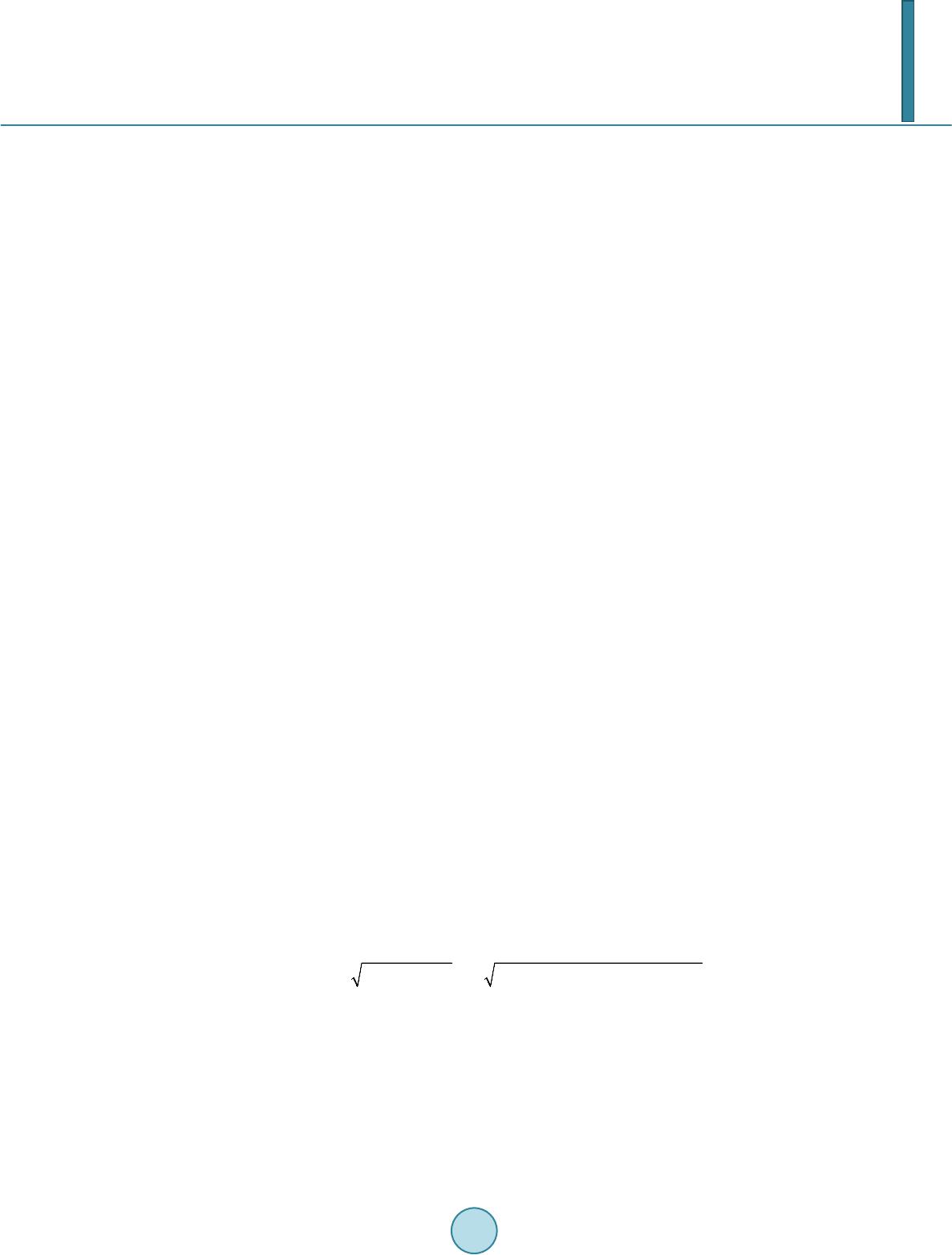
C. J. Xia et al.
the fault.
c) The relationship between real power and reactive power: the real power and the reactive power have stabi-
lized to a small range within 90 ms since the fault o ccurred and the power factor is relatively low. In the later
recovery stage, reactive power becomes an important factor which restrict s the recovery of real power. It can be
confirmed by this p he no me no n: as the reactive power nearly reaches to the peak value, the real power decreases
quickly.
3) When a three-phase metallic short circuit occurs on the commutation bus, P and Q will drop to zero and
never cause oscillation. When the fault is cleared, great real power may be transferred reversely and the reactive
power increases with oscillation. As the commutation recover, the real power increases continuously, while the
reactive power still oscillates. When the real power recovers to about 0.74 p.u., the reactive power reaches to the
peak value of 0.94 p.u. and the restoration of DC power will be significantly decelerated.
In order to make the DC system have better operating performance, it’s hoped that the real power of HVDC
will recover as soon as possible in engineering to maintain the rotor angle stability between sending and receiv-
ing system and the reactive power will be consumed as little as possible to prevent the voltage instabilit y of the
receiving power grid. According to the analysis above, we can find that: P and Q are relevant to the dynamic
feature of converter: the reactive power demand may reaches to two peak values. The peak value appears during
the fault has great impact on the voltage stability of the AC grid, while the reactive power that capacitors of the
converter station provide decreases because of the voltage drop, which means the convertors will consume great
reactive power from the AC grid and drag the voltage down, leading to a vicious circle. During the recovery, the
restoration of real power is restricted by the reactive power. The former 100 ms since the fault was cleared, the
real power has increased quickly because of the abundant reactive power, while as the reactive power nearly
reached to the peak (about 0.75 p.u.), the real power increased slowly. The following passage will focus on the
physical process analysis related to the two peaks of dynamic reactive power, and put forward several strategies
for suppressing the peak of the dynamic reactive power and real power recovery acceleration.
3. Physical Process Analysis Related to the Peak of Dynamic Reactive Power
The control system plays an important part in the operation of HVDC which influences the power and voltage
[8]. T he control mode will usually be switched many times during the faul t and its recovery, so it’s not appro-
priate to anal yze based on a constant control mode assumption. The following passage will analyze the physical
process related to dynamic reactive power peak combining with the switch logic of control system.
Though many new features are added to HVDC control system as the development of mordent control theory,
the typical control feature is still be used in Yun-Guang HVDC control system because of the conservatism of
power industry. The control mode of the rectifier includes constant current control (including VDCOL) and con-
stant αmin control while the control mode of the inverter includes constant voltage control, constant γ co ntr o l,
constant current control (including VDCOL) and constant αmin control. The control instructions of control layers
above pole control such as power modulation are not considered for the following two reasons: 1) the time con-
stant of pole control instruction is high; 2) the minimum current value is chosen from all current instructions in
pole control. The current value set by the power control instruction won’t be chosen since it is high. Though the
control mode is chosen automatically in valve control layer (rectifier chooses the minimum value, while inverter
chooses the maximum value), the control mode switch logic during the transient period of large-di st ur ba nce is
shown in Figure 3 according to a lot of simulation results. The inverter power expression is derived below:
i
22 22
( cos)
()()(cos )
didi dmici dd
did midid mimiicid
PUIKERI I
QI KEUIKEKERI
γ
γ
= =−
=−=− −
(1)
Pdi and Qdi are respectively the real power the converters transmit and reactive power the converters consume.
Ei, Udi, Id, γi are respectively the AC voltage, DC voltage, DC current and extinction angle of inverter. Km and Rci
are constant values.
3.1. The First Peak of Dynamic Reactive Power
The fault may cause an inverter commutation failure which leads to the decrease of Udc in inverter and the in-
crease of Id. It can be found in Figure 3 that inverter will choose the constant γ control mode which will increase
β rapidly. Since the voltage of commutation bus doesn’t drop to zero during the fault, the inverter will recover