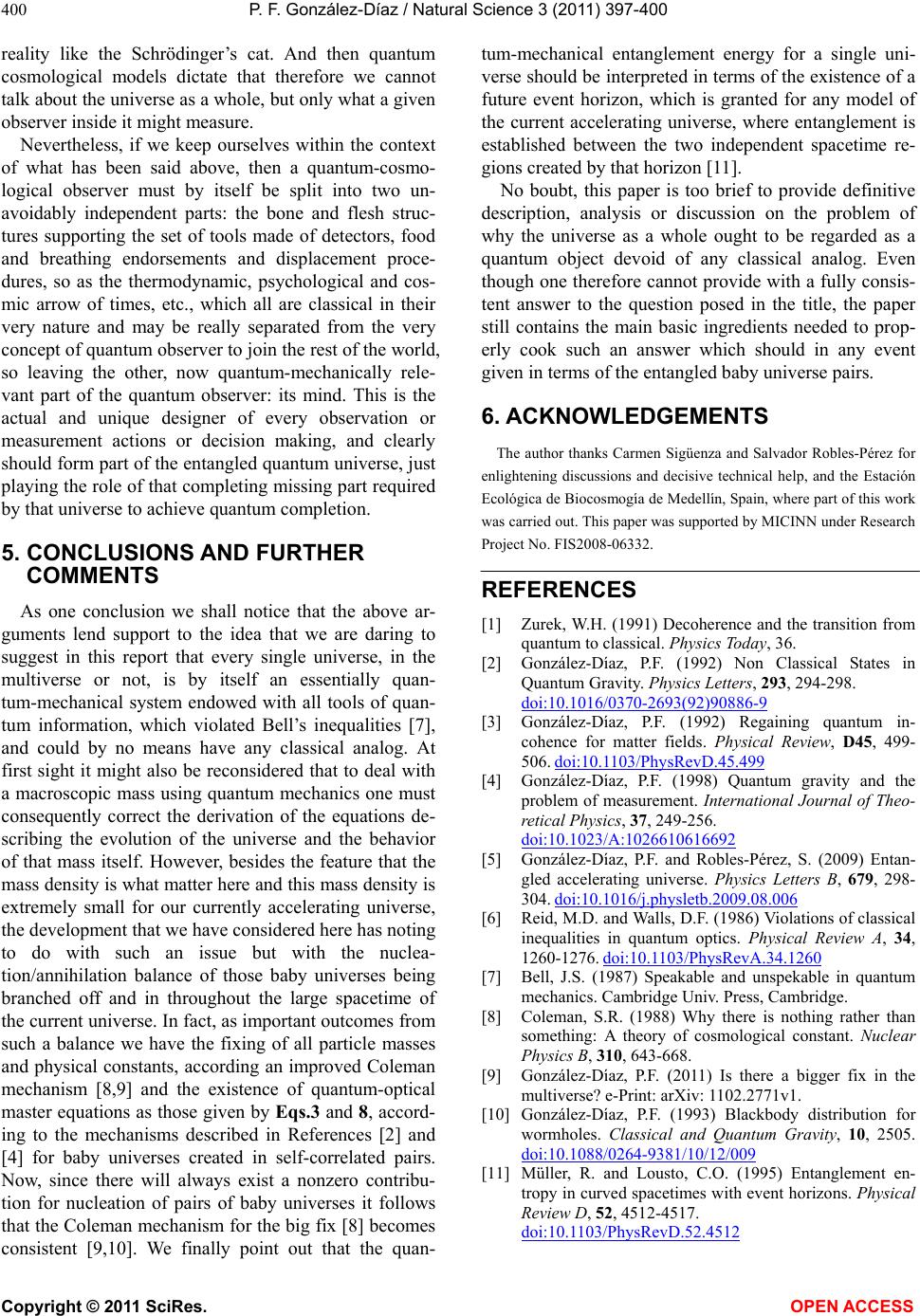
P. F. González-Díaz / Natural Science 3 (2011) 397-400
Copyright © 2011 SciRes. OPEN ACCESS
400
reality like the Schrödinger’s cat. And then quantum
cosmological models dictate that therefore we cannot
talk about the universe as a whole, but only what a given
observer inside it might measure.
Nevertheless, if we keep ourselves within the context
of what has been said above, then a quantum-cosmo-
logical observer must by itself be split into two un-
avoidably independent parts: the bone and flesh struc-
tures supporting the set of tools made of detectors, food
and breathing endorsements and displacement proce-
dures, so as the thermodynamic, psychological and cos-
mic arrow of times, etc., which all are classical in their
very nature and may be really separated from the very
concept of quantum observer to join the rest of the world,
so leaving the other, now quantum-mechanically rele-
vant part of the quantum observer: its mind. This is the
actual and unique designer of every observation or
measurement actions or decision making, and clearly
should form part of the entangled quantum universe, just
playing the role of that completing missing part required
by that universe to achieve quantum completion.
5. CONCLUSIONS AND FURTHER
COMMENTS
As one conclusion we shall notice that the above ar-
guments lend support to the idea that we are daring to
suggest in this report that every single universe, in the
multiverse or not, is by itself an essentially quan-
tum-mechanical system endowed with all tools of quan-
tum information, which violated Bell’s inequalities [7],
and could by no means have any classical analog. At
first sight it might also be reconsidered that to deal with
a macroscopic mass using quantum mechanics one must
consequently correct the derivation of the equations de-
scribing the evolution of the universe and the behavior
of that mass itself. However, besides the feature that the
mass density is what matter here and this mass density is
extremely small for our currently accelerating universe,
the development that we have considered here has noting
to do with such an issue but with the nuclea-
tion/annihilation balance of those baby universes being
branched off and in throughout the large spacetime of
the current universe. In fact, as important outcomes from
such a balance we have the fixing of all particle masses
and physical constants, according an improved Coleman
mechanism [8,9] and the existence of quantum-optical
master equations as those given by Eqs.3 and 8, accord-
ing to the mechanisms described in References [2] and
[4] for baby universes created in self-correlated pairs.
Now, since there will always exist a nonzero contribu-
tion for nucleation of pairs of baby universes it follows
that the Coleman mechanism for the big fix [8] becomes
consistent [9,10]. We finally point out that the quan-
tum-mechanical entanglement energy for a single uni-
verse should be interpreted in terms of the existence of a
future event horizon, which is granted for any model of
the current accelerating universe, where entanglement is
established between the two independent spacetime re-
gions created by that horizon [11].
No boubt, this paper is too brief to provide definitive
description, analysis or discussion on the problem of
why the universe as a whole ought to be regarded as a
quantum object devoid of any classical analog. Even
though one therefore cannot provide with a fully consis-
tent answer to the question posed in the title, the paper
still contains the main basic ingredients needed to prop-
erly cook such an answer which should in any event
given in terms of the entangled baby universe pairs.
6. ACKNOWLEDGEMENTS
The author thanks Carmen Sigüenza and Salvador Robles-Pérez for
enlightening discussions and decisive technical help, and the Estación
Ecológica de Biocosmogía de Medellín, Spain, where part of this work
was carried out. This paper was supported by MICINN under Research
Project No. FIS2008-06332.
REFERENCES
[1] Zurek, W.H. (1991) Decoherence and the transition from
quantum to classical. Physics Today, 36.
[2] González-Díaz, P.F. (1992) Non Classical States in
Quantum Gravity. Physics Letters, 293, 294-298.
doi:10.1016/0370-2693(92)90886-9
[3] González-Díaz, P.F. (1992) Regaining quantum in-
cohence for matter fields. Physical Review, D45, 499-
506. doi:10.1103/PhysRevD.45.499
[4] González-Díaz, P.F. (1998) Quantum gravity and the
problem of measurement. International Journal of Theo-
retical Physics, 37, 249-256.
doi:10.1023/A:1026610616692
[5] González-Díaz, P.F. and Robles-Pérez, S. (2009) Entan-
gled accelerating universe. Physics Letters B, 679, 298-
304. doi:10.1016/j.physletb.2009.08.006
[6] Reid, M.D. and Walls, D.F. (1986) Violations of classical
inequalities in quantum optics. Physical Review A, 34,
1260-1276. doi:10.1103/PhysRevA.34.1260
[7] Bell, J.S. (1987) Speakable and unspekable in quantum
mechanics. Cambridge Univ. Press, Cambridge.
[8] Coleman, S.R. (1988) Why there is nothing rather than
something: A theory of cosmological constant. Nuclear
Physics B, 310, 643-668.
[9] González-Díaz, P.F. (2011) Is there a bigger fix in the
multiverse? e-Print: arXiv: 1102.2771v1.
[10] González-Díaz, P.F. (1993) Blackbody distribution for
wormholes. Classical and Quantum Gravity, 10, 2505.
doi:10.1088/0264-9381/10/12/009
[11] Müller, R. and Lousto, C.O. (1995) Entanglement en-
tropy in curved spacetimes with event horizons. Physical
Review D, 52, 4512-4517.
doi:10.1103/PhysRevD.52.4512