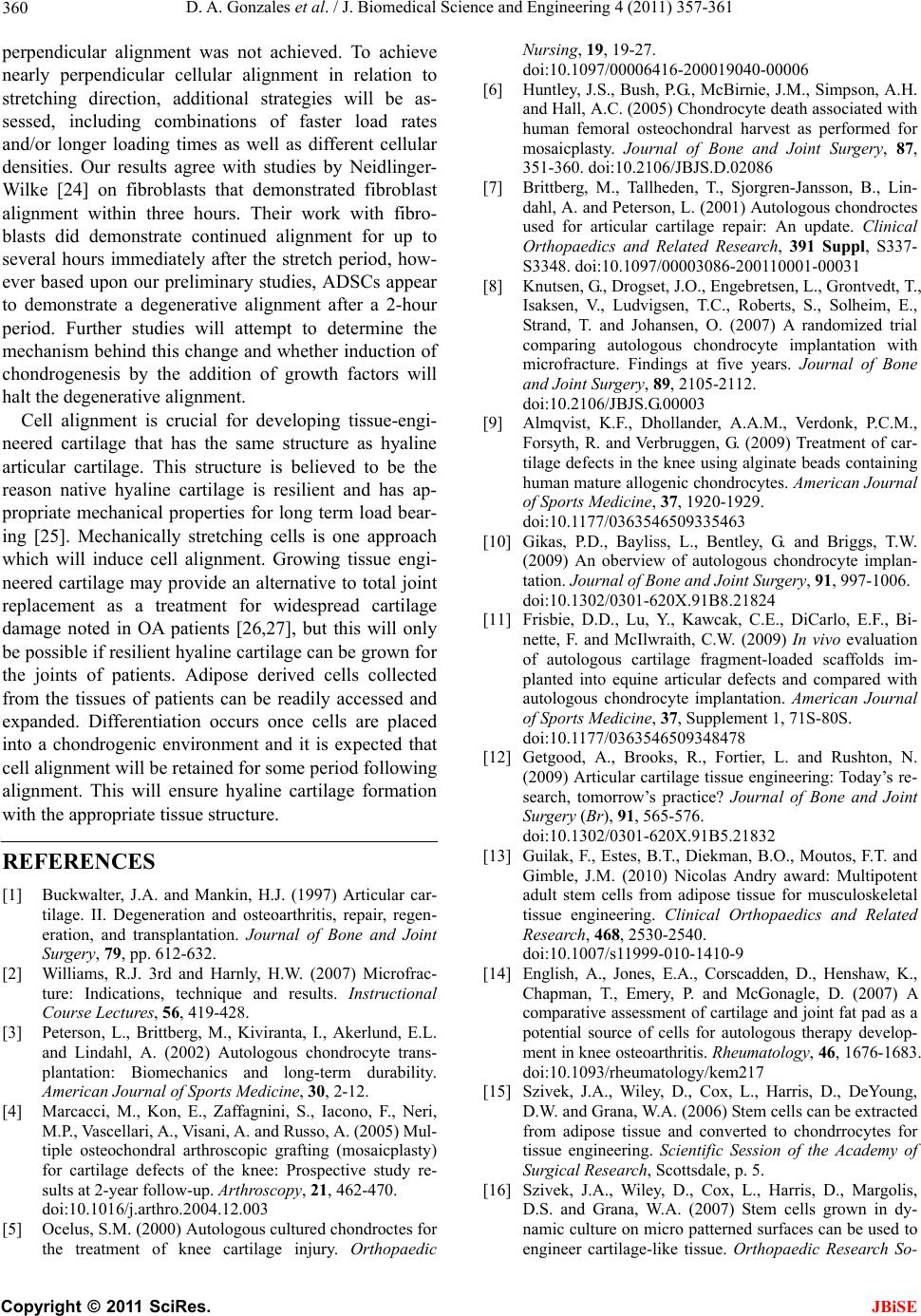
D. A. Gonzales et al. / J. Biomedical Science and Engineering 4 (2011) 357-361
360
perpendicular alignment was not achieved. To achieve
nearly perpendicular cellular alignment in relation to
stretching direction, additional strategies will be as-
sessed, including combinations of faster load rates
and/or longer loading times as well as different cellular
densities. Our results agree with studies by Neidlinger-
Wilke [24] on fibroblasts that demonstrated fibroblast
alignment within three hours. Their work with fibro-
blasts did demonstrate continued alignment for up to
several hours immediately after the stretch period, how-
ever based upon our preliminary studies, ADSCs appear
to demonstrate a degenerative alignment after a 2-hour
period. Further studies will attempt to determine the
mechanism behind this change and whether induction of
chondrogenesis by the addition of growth factors will
halt the degenerative alignment.
Cell alignment is crucial for developing tissue-engi-
neered cartilage that has the same structure as hyaline
articular cartilage. This structure is believed to be the
reason native hyaline cartilage is resilient and has ap-
propriate mechanical properties for long term load bear-
ing [25]. Mechanically stretching cells is one approach
which will induce cell alignment. Growing tissue engi-
neered cartilage may provide an alternative to total joint
replacement as a treatment for widespread cartilage
damage noted in OA patients [26,27], but this will only
be possible if resilien t hya line cartilage can be grown for
the joints of patients. Adipose derived cells collected
from the tissues of patients can be readily accessed and
expanded. Differentiation occurs once cells are placed
into a chondrogenic environment and it is expected that
cell alignment will be retained for so me period fo llowing
alignment. This will ensure hyaline cartilage formation
with the appropriate tissue structure.
REFERENCES
[1] Buckwalter, J.A. and Mankin, H.J. (1997) Articular car-
tilage. II. Degeneration and osteoarthritis, repair, regen-
eration, and transplantation. Journal of Bone and Joint
Surgery, 79, pp. 612-632.
[2] Williams, R.J. 3rd and Harnly, H.W. (2007) Microfrac-
ture: Indications, technique and results. Instructional
Course Lectures, 56, 419-428.
[3] Peterson, L., Brittberg, M., Kiviranta, I., Akerlund, E.L.
and Lindahl, A. (2002) Autologous chondrocyte trans-
plantation: Biomechanics and long-term durability.
American Journal of Sports Medicine, 30, 2-12.
[4] Marcacci, M., Kon, E., Zaffagnini, S., Iacono, F., Neri,
M.P., Vascellari, A., Visani, A. and Russo, A. (2005) Mul-
tiple osteochondral arthroscopic grafting (mosaicplasty)
for cartilage defects of the knee: Prospective study re-
sults at 2-year follow-up. Arthroscopy, 21, 462-470.
doi:10.1016/j.arthro.2004.12.003
[5] Ocelus, S.M. (2000) Autologous cultured chondroctes for
the treatment of knee cartilage injury. Orthopaedic
Nursing, 19, 19-27.
doi:10.1097/00006416-200019040-00006
[6] Huntley, J.S., Bush, P.G. , McBirnie, J.M., Simpson, A.H.
and Hall, A.C. (2005) Chondrocyte death associated with
human femoral osteochondral harvest as performed for
mosaicplasty. Journal of Bone and Joint Surgery, 87,
351-360. doi:10.2106/JBJS.D.02086
[7] Brittberg, M., Tallheden, T., Sjorgren-Jansson, B., Lin-
dahl, A. and Peterson, L. (2001) Autologous chondroctes
used for articular cartilage repair: An update. Clinical
Orthopaedics and Related Research, 391 Suppl, S337-
S3348. doi:10.1097/00003086-200110001-00031
[8] Knutsen, G., Drogset, J.O., Engebretsen, L., Grontvedt, T.,
Isaksen, V., Ludvigsen, T.C., Roberts, S., Solheim, E.,
Strand, T. and Johansen, O. (2007) A randomized trial
comparing autologous chondrocyte implantation with
microfracture. Findings at five years. Journal of Bone
and Joint Surgery, 89, 2105-2112.
doi:10.2106/JBJS.G.00003
[9] Almqvist, K.F., Dhollander, A.A.M., Verdonk, P.C.M.,
Forsyth, R. and Verbruggen, G. (2009) Treatment of car-
tilage defects in the knee using alginate beads containing
human mature allogenic chondrocytes. American Journal
of Sports Medicine, 37, 1920-1929.
doi:10.1177/0363546509335463
[10] Gikas, P.D., Bayliss, L., Bentley, G. and Briggs, T.W.
(2009) An oberview of autologous chondrocyte implan-
tation. Journal of Bone and Joint Surgery, 91, 997-1006.
doi:10.1302/0301-620X.91B8.21824
[11] Frisbie, D.D., Lu, Y., Kawcak, C.E., DiCarlo, E.F., Bi-
nette, F. and McIlwraith, C.W. (2009) In vivo evaluation
of autologous cartilage fragment-loaded scaffolds im-
planted into equine articular defects and compared with
autologous chondrocyte implantation. American Journal
of Sports Medicine, 37, Supplement 1, 71S-80S.
doi:10.1177/0363546509348478
[12] Getgood, A., Brooks, R., Fortier, L. and Rushton, N.
(2009) Articular cartilage tissue engineering: Today’s re-
search, tomorrow’s practice? Journal of Bone and Joint
Surgery (Br), 91, 565-576.
doi:10.1302/0301-620X.91B5.21832
[13] Guilak, F., Estes, B.T., Diekman, B.O., Moutos, F.T. and
Gimble, J.M. (2010) Nicolas Andry award: Multipotent
adult stem cells from adipose tissue for musculoskeletal
tissue engineering. Clinical Orthopaedics and Related
Research, 468, 2530-2540.
doi:10.1007/s11999-010-1410-9
[14] English, A., Jones, E.A., Corscadden, D., Henshaw, K.,
Chapman, T., Emery, P. and McGonagle, D. (2007) A
comparative assessment of cartilage and joint fat pad as a
potential source of cells for autologous therapy develop-
ment in knee osteoarthritis. Rheumatology, 46, 1676-1683.
doi:10.1093/rheumatology/kem217
[15] Szivek, J.A., Wiley, D., Cox, L., Harris, D., DeYoung,
D.W. and Grana, W.A. (2006) Stem cells can be extracted
from adipose tissue and converted to chondrrocytes for
tissue engineering. Scientific Session of the Academy of
Surgical Research, Scottsdale, p. 5.
[16] Szivek, J.A., Wiley, D., Cox, L., Harris, D., Margolis,
D.S. and Grana, W.A. (2007) Stem cells grown in dy-
namic culture on micro patterned surfaces can be used to
engineer cartilage-like tissue. Orthopaedic Research So-
C
opyright © 2011 SciRes. JBiSE