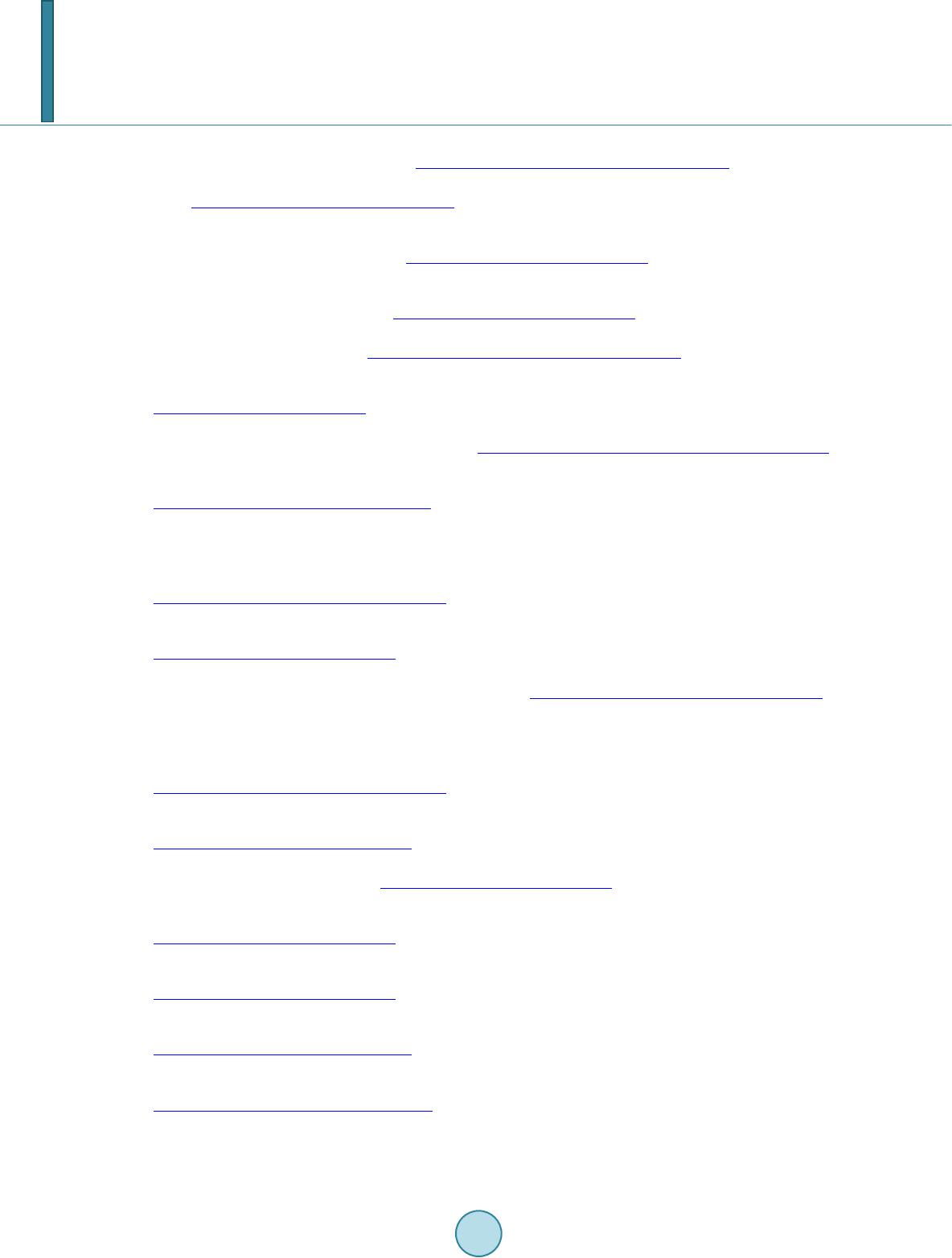
C. O. Nwoko
Giovannetti, M., & Mosse, B. (1980). An Evaluation of Techniques for Measuring Vesicular Arbuscular Mycorrhizal Infec-
tion in Roots. New Phytologist, 84, 489-500. http://dx.doi.org/10.1111/j.1469-8137.1980.tb04556.x
Harborne, B. (1984). Photochemical Methods. A Guide to Modern Techniques of Plant Analysis. London: Chapman & Hall
Press. http://dx.doi.org/10.1007/978-94-009-5570-7
Houngnandan, P., Sanginga, N., Okogun, A., Vanlauwe, B., Merckx, R., & Van Cleemput, O. (2001). Assessment of Soil
Factors Limiting Growth and Establishment of Mucuna in Farmers’ Fields in the Derived Savanna of the Benin Republic.
Biology and Fertility of Soils, 33, 416-422. http://dx.doi.org/10.1007/s003740100347
Houngnandan, P., Sanginga, N., Woomer, P., Vanlauwe, B., & Van Cleemput, O. (2000). Response of Mucuna pruriens to
Symbiotic Nitrogen Fixation by Rhizobia Following Inoculation in Farmers’ Fields in the Derived Savanna of Benin. Bi-
ology and Fertility of Soils, 30, 558-565. http://dx.doi.org/10.1007/s003740050036
Jakobsen, I., & Rosendahl, L. (1990). Carbon Flow into Soil and External Hyphae from Roots of Mycorrhizal Cucumber
Roots. New Phytologist, 115, 77-83. http://dx.doi.org/10.1111/j.1469-8137.1990.tb00924.x
Jones, E. J., & Ley val, C. (2003). Rhizosphere Gradients of Polycyclic Aromatic Hydrocarbon (PAH) Dissipation in Two
Industrial Soils and the Impact of Srbuscular Mycorrhiza. Environmental Science & Technology, 37, 2371-2375.
http://dx.doi.org/10.1021/es020196y
Leyval, C., & Binet, P. (1998). Effect of Polyaromatic Hydrocarbons (PAHs) and Arbuslar Mycorrhizal Colonization of
Plants. Journal of Environmental Quality, 27, 402-407. http://dx.doi.org/10.2134/jeq1998.00472425002700020022x
Miller, R. M., & Jastrow, J. D. (2000). Mycorrhizal Fungi Influence Soil Structure. In: Y. Kapulnik, & D. D. Douds, Eds.,
Arbuscular Mycorrhizas: Physiology and Function (pp. 3-18). Dordrecht: Kluwer Academic.
http://dx.doi.org/10.1007/978-94-017-0776-3_1
Nwoko, C. O., & Ogunyemi, S. (2010). Effect of Palm Oil Mill Effluent (POME) on Microbial Characteristics in a Humid
Tropical Soil under Laboratory Conditions. International Journal of Environmental Science and Development, 1, 308-314.
Nwoko, H., & Sanginga, N. (1999). Dependency of Promiscuous Soybean and Herbaceous Legumes on Arbuscular Mycorr-
hizal Fungi and Their Response to Bradyrhizobial Inoculation in Low P Soils. Applied Soil Ecology, 13, 251-258.
http://dx.doi.org/10.1016/S0929-1393(99)00038-4
Oliveira, R. S., Dodd, J. C., & Castro, P. M. L. (2001). The Mycorrhizal Status of Phragmites australis in Several Polluted
Soils and Sediments of an Industrialized Region of Northern Portugal. Mycorrhiza, 10, 241-247.
http://dx.doi.org/10.1007/s005720000087
Olsson, P. A., Thingstrup, I., Jakobsen, I., & Baath, E. (1999). Estimation of the Biomass of Arbuscular Mycorrhizal Fungi
in a Linseed Field. Soil Biology & Biochemistry, 31, 1879-1887. http://dx.doi.org/10.1016/S0038-0717(99)00119-4
Onosode, G. O. (2003).The Vision: Niger Delta Environmental Survey. In B. A. Chokor, Ed., Environmental Issues and
Challenges of the Niger Delta (pp. 78-98). The CIBN Press Ltd.
Phillips, J. M., & Hayman, D. S. (1970). Improved Procedures for Clearing and Staining Parasitic and Vesicular-Arbuscular
Mycorrhizal Fungi for Rapid Assessment of Infection. Transcriptions of the British Mycology Society, 55, 158-161.
http://dx.doi.org/10.1016/S0007-1536(70)80110-3
Rillig, M. C., Wright, S. F., Nichols, K. A., Schmidt, W. F., & Torn, M. S. (2001). Large Contribution of Arbuscular My-
corrhizal Fungi to Soil Carbon Pools in Tropical Forest Soils. Plant Soil, 233, 167-177.
http://dx.doi.org/10.1023/A:1010364221169
Rogers, J. A., Tedaldi, D. J., & Kavanaugh, M. C. (1993). A Screening Protocol for Bioremediation of Contaminated Soil.
Environmental Progress, 12, 146-149. http://dx.doi.org/10.1002/ep.670120213
Salzer, P., Corbiere, H., & Boller, T. (1999). Hydrogen Peroxide Accumulatiob in Medicago Truncatula Roots Colonized by
the Arbuscualr Mycorrhiza Forming Fungus Glomus intraradices. Planta, 208, 319-325.
http://dx.doi.org/10.1007/s004250050565
Sanginga, N., Carsky, R. J., & Dashiell, K. (1999). Arbuscular Mycorrhizal Fungi Respond to Rhizobial Inoculation and
Cropping Systems in Farmers’ Fields in the Guinea Savanna. Biology and Fertility of Soils, 30, 179-186.
http://dx.doi.org/10.1007/s003740050606
Sanginga, N., Lyasse, O., & Singh, B. B. (2000). Phosphorus Use Efficiency and Nitrogen Balance of Cowpea Breeding
Lines in a Low P Soil of the Derived Savanna Zone in West Africa. Plant and Soil, 220, 119-128.
http://dx.doi.org/10.1023/A:1004785720047
Wu, F. Y., Yu, X. Z., Wu, S. C., Lin, X. G., & Wong, M. H. (2011). Phenanthrene and Pyrene Uptake by Arbuscular My-
corrhizal Maize and Their Dissipation in Soil. Journal of Hazardous Materials, 187, 341-347.
http://dx.doi.org/10.1016/j.jhazmat.2011.01.024