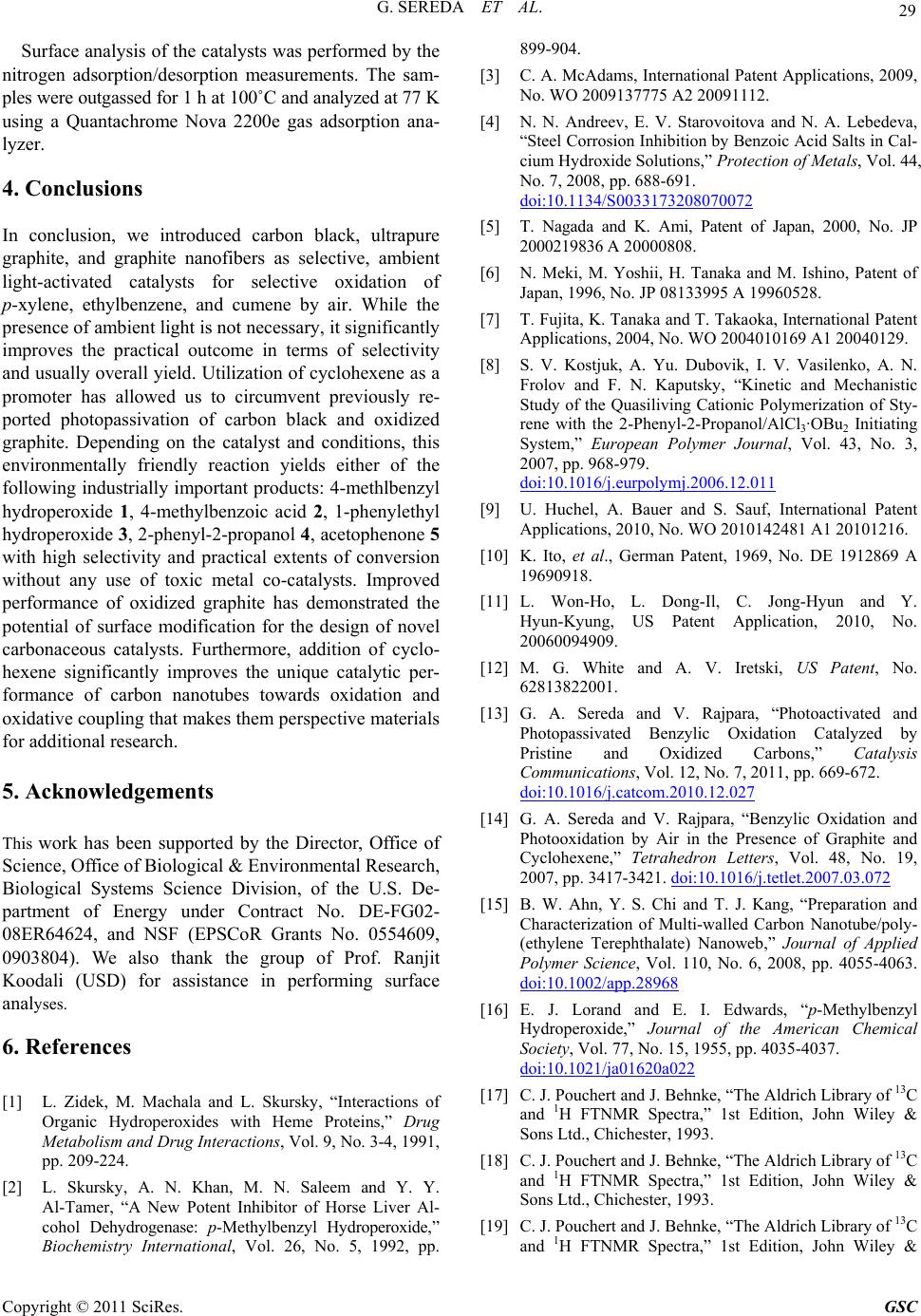
G. SEREDA ET AL.
29
Surface analysis of the catalysts was performed by the
nitrogen adsorption/desorption measurements. The sam-
ples were outgassed for 1 h at 100˚C and analyzed at 77 K
using a Quantachrome Nova 2200e gas adsorption ana-
lyzer.
4. Conclusions
In conclusion, we introduced carbon black, ultrapure
graphite, and graphite nanofibers as selective, ambient
light-activated catalysts for selective oxidation of
p-xylene, ethylbenzene, and cumene by air. While the
presence of ambient light is not necessary, it significantly
improves the practical outcome in terms of selectivity
and usually overall yield. Utilization of cyclohexene as a
promoter has allowed us to circumvent previously re-
ported photopassivation of carbon black and oxidized
graphite. Depending on the catalyst and conditions, this
environmentally friendly reaction yields either of the
following industrially important products: 4-methlbenzyl
hydroperoxide 1, 4-methylbenzoic acid 2, 1-phenylethyl
hydroperoxide 3, 2-phenyl-2-propanol 4, acetophenone 5
with high selectivity and practical extents of conversion
without any use of toxic metal co-catalysts. Improved
performance of oxidized graphite has demonstrated the
potential of surface modification for the design of novel
carbonaceous catalysts. Furthermore, addition of cyclo-
hexene significantly improves the unique catalytic per-
formance of carbon nanotubes towards oxidation and
oxidative coupling that makes them perspective materials
for additional research.
5. Acknowledgements
This work has been supported by the Director, Office of
Science, Office of Biological & Environmental Research,
Biological Systems Science Division, of the U.S. De-
partment of Energy under Contract No. DE-FG02-
08ER64624, and NSF (EPSCoR Grants No. 0554609,
0903804). We also thank the group of Prof. Ranjit
Koodali (USD) for assistance in performing surface
analyses.
6. References
[1] L. Zidek, M. Machala and L. Skursky, “Interactions of
Organic Hydroperoxides with Heme Proteins,” Drug
Metabolism and Drug Interactions, Vol. 9, No. 3-4, 1991,
pp. 209-224.
[2] L. Skursky, A. N. Khan, M. N. Saleem and Y. Y.
Al-Tamer, “A New Potent Inhibitor of Horse Liver Al-
cohol Dehydrogenase: p-Methylbenzyl Hydroperoxide,”
Biochemistry International, Vol. 26, No. 5, 1992, pp.
899-904.
[3] C. A. McAdams, International Patent Applications, 2009,
No. WO 2009137775 A2 20091112.
[4] N. N. Andreev, E. V. Starovoitova and N. A. Lebedeva,
“Steel Corrosion Inhibition by Benzoic Acid Salts in Cal-
cium Hydroxide Solutions,” Protection of Metals, Vol. 44,
No. 7, 2008, pp. 688-691.
doi:10.1134/S0033173208070072
[5] T. Nagada and K. Ami, Patent of Japan, 2000, No. JP
2000219836 A 20000808.
[6] N. Meki, M. Yoshii, H. Tanaka and M. Ishino, Patent of
Japan, 1996, No. JP 08133995 A 19960528.
[7] T. Fujita, K. Tanaka and T. Takaoka, International Patent
Applications, 2004, No. WO 2004010169 A1 20040129.
[8] S. V. Kostjuk, A. Yu. Dubovik, I. V. Vasilenko, A. N.
Frolov and F. N. Kaputsky, “Kinetic and Mechanistic
Study of the Quasiliving Cationic Polymerization of Sty-
rene with the 2-Phenyl-2-Propanol/AlCl3·OBu2 Initiating
System,” European Polymer Journal, Vol. 43, No. 3,
2007, pp. 968-979.
doi:10.1016/j.eurpolymj.2006.12.011
[9] U. Huchel, A. Bauer and S. Sauf, International Patent
Applications, 2010, No. WO 2010142481 A1 20101216.
[10] K. Ito, et al., German Patent, 1969, No. DE 1912869 A
19690918.
[11] L. Won-Ho, L. Dong-Il, C. Jong-Hyun and Y.
Hyun-Kyung, US Patent Application, 2010, No.
20060094909.
[12] M. G. White and A. V. Iretski, US Patent, No.
62813822001.
[13] G. A. Sereda and V. Rajpara, “Photoactivated and
Photopassivated Benzylic Oxidation Catalyzed by
Pristine and Oxidized Carbons,” Catalysis
Communications, Vol. 12, No. 7, 2011, pp. 669-672.
doi:10.1016/j.catcom.2010.12.027
[14] G. A. Sereda and V. Rajpara, “Benzylic Oxidation and
Photooxidation by Air in the Presence of Graphite and
Cyclohexene,” Tetrahedron Letters, Vol. 48, No. 19,
2007, pp. 3417-3421. doi:10.1016/j.tetlet.2007.03.072
[15] B. W. Ahn, Y. S. Chi and T. J. Kang, “Preparation and
Characterization of Multi-walled Carbon Nanotube/poly-
(ethylene Terephthalate) Nanoweb,” Journal of Applied
Polymer Science, Vol. 110, No. 6, 2008, pp. 4055-4063.
doi:10.1002/app.28968
[16] E. J. Lorand and E. I. Edwards, “p-Methylbenzyl
Hydroperoxide,” Journal of the American Chemical
Society, Vol. 77, No. 15, 1955, pp. 4035-4037.
doi:10.1021/ja01620a022
[17] C. J. Pouchert and J. Behnke, “The Aldrich Library of 13C
and 1H FTNMR Spectra,” 1st Edition, John Wiley &
Sons Ltd., Chichester, 1993.
[18] C. J. Pouchert and J. Behnke, “The Aldrich Library of 13C
and 1H FTNMR Spectra,” 1st Edition, John Wiley &
Sons Ltd., Chichester, 1993.
[19] C. J. Pouchert and J. Behnke, “The Aldrich Library of 13C
and 1H FTNMR Spectra,” 1st Edition, John Wiley &
Copyright © 2011 SciRes. GSC