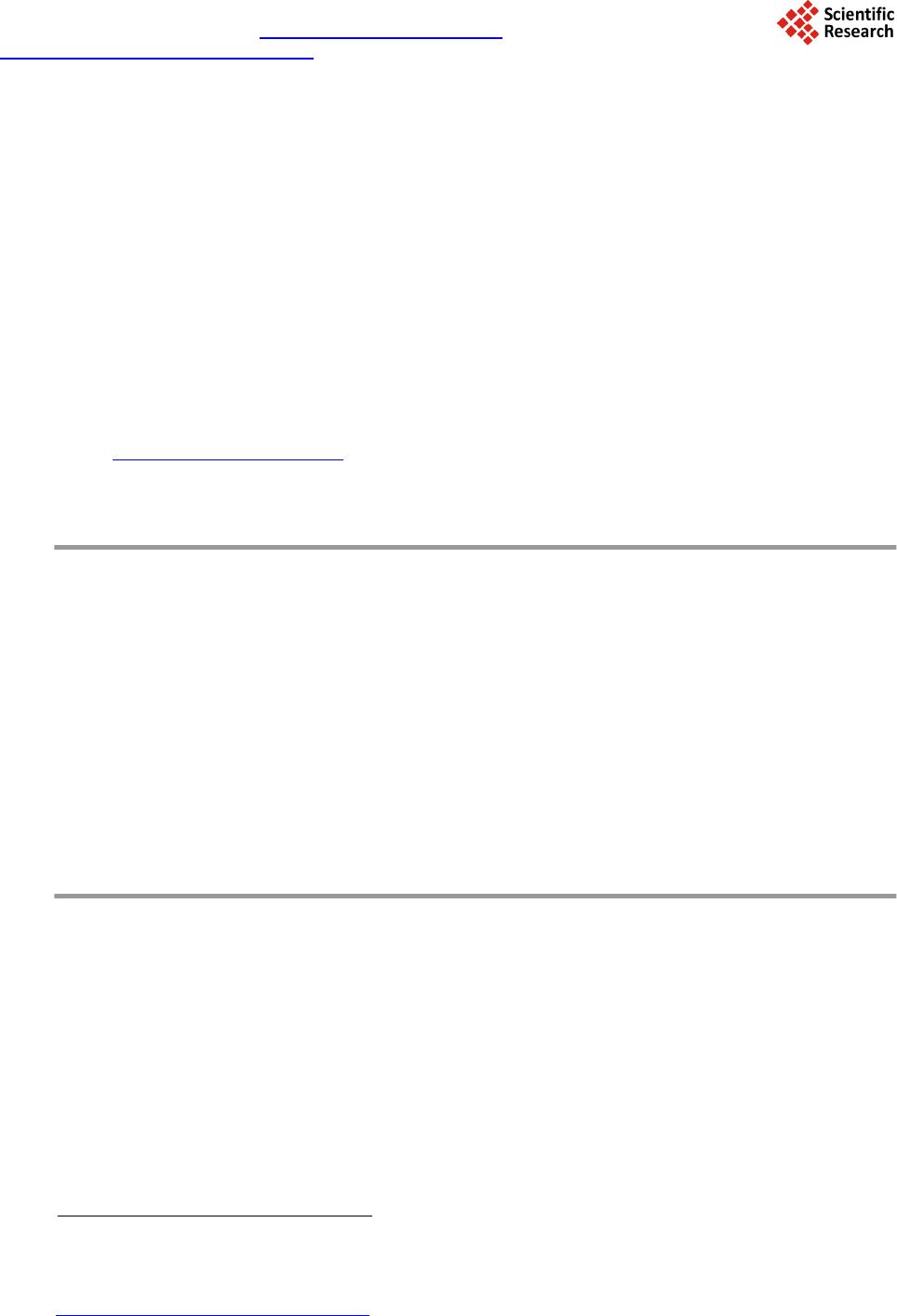 Journal of Agricultural Chemistry and Environment, 2014, 3, 35-40 Published Online April 2014 in SciRes. http://www.scirp.org/journal/jacen http://dx.doi.org/10.4236/jacen.2014.32B006 How to cite this paper: Dragone, R., et al. (2014) Sensor with Intact or Modified Yeast Cells as Rapid Device for Toxicologi- cal Test of Chemicals. Journal of Agricultural Chemistry and Environment, 3, 35-40. http://dx.doi.org/10.4236/jacen.2014.32B006 Sensor with Intact or Modified Yeast Cells as Rapid Device for Toxicological Test of Chemicals Roberto Dragone1*, Chiara Frazzoli2, Gerardo Grasso3, Gloria Rossi1 1Institute of Nanostructured Materials, Consiglio Nazionale delle Ricerche, Rome, Italy 2Department of Veterinary Public Health and Food Safety, Istituto Superiore di Sanità, Rome, Italy 3 Department of Agricultural, Environmental and Food Sciences, University of Molise, Campobasso, Italy Email: *roberto.dragone@uniroma1.it Received February 2014 Abstract Aerobic catabolism of S. cerevisiae (cell respir ation ) is a rapid, cost-effective, and reproducible toxicological endpoint of the whole cells biosensor. To increase the signal intensity, a protocol for the immobilization and modification of the yeast cells is described. In particular, the enzymatic treatment of the immobilized yeast cells allows removing the cell wall and obtaining structurally modified cells namely spheroplasts. Both immobilization and exposure of sensitive cells like spheroplasts confirmed to improve the method’s sensitivity vs. the chemicals. The present paper reports the test of different chemicals (including Mercury and wood preservative like Tanalith) present in consumer products, performed both by sensor with intact and modified whole cells. Keywords Screening Meth od s; Spheroplast; Whole Cell; Tanalit h; Me rc u ry 1. Introduction Since 1990s biosensors have been studied and developed to test and screen inorganic and organic substances [1-3]. A biosensor consists of 1) a biolo gical rec ognition e le ment, na mely t he bio mediator, and 2) a tr ansd uc tion element, namely the sensor. Among the wide range of biosensors, those whole cells-based have the advantage of exposing a live entity: this implies the test of multiple possible functional targets simultaneously [4]. For in- stance, regular aerobic respiration is indicator of normal metabolism of glucose (involving more than 20 en- zymes) and mitochondrial functions. The whole cells biosensor based on cellular respiration offers rapid and re- producible measures while allowing useful toxicity ranking of chemicals: in fact, the semiquantitative result is expressed as toxicity index [4]. A key step in the improvement of the whole cells biosensor based on respiration as functional toxicological endpoint has been the i mmobilizatio n of the biomediator . In fact, the use of suspend ed cells implies the a ddition *
 R. Dragone et al. of the cellular suspension to the sample and therefore a sample dilution that, in some cases and depending on method’s LoD, may impair the measurement. The method’s improvement in sensitivity by immobilization of cells has been detailed and applied in our previous studies, either with yeast (Saccharomyces cerevisiae) and huma n cells (U -937 cell line) [1,5]. The application on human cells confirmed respiration as robust endpoint to investigate effects on different metabolisms, whereas yeast is partic ularly interest ing for its lo w cost, rapid reac- tion and easy handling. In general, yeast is recognized as model organism and is widely used in genetics and molecular biology also for its short generation time (doubling time of ca. 90 - 140 min at 30˚C depending on media). A fur ther ste p in t he improvement of method’s sensitivity involves the partial enzymatic removal of cell wall and therefore the exposure of a more vulnerable entity, namely the spheroplast [6]. In fact, the cell wall (that is a 70 - 100 nm thick layered structure in S. cerevisiae) may act as chemical-physical shie ld again st chemical stres- sors and/or affect the cellular uptake of chemicals (due e.g. to surface adsorption and chelation). The present paper describes the protocol and findings of this improvement. 2. Materials and Methods 2.1. Materials The yeast cells used are of Saccharomyces cerevisiae Type II (Sigma-Aldrich, USA), Agar microbiology tested and powdered (Sigma-Aldrich, US A). The compositio n of the biolo gical medium wa s as follows: yeast e xtracts for microbiology (Merck, Germany), 0.17% Universal Peptone M66, 0.17%, D-Glucose monohydrate, 0.30%, containi ng agar, 1 %. Chemical used in consumer products like herbicides, insecticides, pesticide, antiseptics, detergents, disinfec- tants, and surfactants were tested and in particular: mercury nitrate 0.005 mol/L (0.01N) (Sigma Aldrich); so- dium dodecyl sulphate, SDS (Fluka, Germany). 4-chlorophenol (4-CP) ≥ 99% (Sigma Aldrich), and a number of wood preservatives, as alkaline copper quaternary, ACQ (Hangzhou Lin’a n Tianho ng Bio -tech Co . Ltd, China) , copper azole (Lonza Wood Protection, Holland), formulation containing copper chelate Cu-N-cyclohexyldia - zenium dioxide and Wolmanit CX-10 (copper chelate Cu-N-cyclohexyldiazenium dioxide wood preservative) (BASF Wolman GmbH, Germany) were tested along with inorganic formulations (chromated copper arsenate, or CCA also known as Tanalith C) and Tanalith E (copper azole wood preservative) (Lonza Wood Protection, Holland). All standards solutions were prepared through dilution with high purity deionized water by Milli-Q® Integral Water Purification Systems (Me rck Millipore, USA). The protocol requires the following reagents: D-sorbitol, sodium sulfite and formaldehyde solution 37% (Fluka, Germany), sodium azide purum p.a. ≥99.0%, ethanol ~96%, zymolyase-20T (AMS Biotechnology (Eu- rope) Ltd, Switzerland). To measure and record dissolved O2 consumption rates, Orion® model 97-08 amperometric O2 gas diffusion sensors (MG Scientific, USA), potentiometer GPL 22 (Crison, Italy) and Amel analogical recorder mod. 868 (Amel, Italy) were used. The biosensor was assembled by coupling the oximeter as signal transducer to an agar disk of immobilized yeast ce lls. T he cur r ent s igna l (d ue to red uc tio n o f O2 at the cathode) is exp resse d i n p pmO2. An agar disk of 10 mm of diameter and a thickness of 1 mm contains the immobilized cells and is anchored to the gas -permeable membrane on the electrode tip. Both agar disks preparation and biosensor assembly followed the procedure already detailed [1]. To avoi d cell contamina tio n, the exte rnal surfac e of the sensor , includ ing the gas-permeable membrane, is cleaned with ethanol 70% v/v solution [7]. 2.2. Methods 2.2.1. Respirometric Test As already described in literature [5], the interactions of the che mical with mitoc hondri al me mbra nes can c ause a change of the cellular respiration. This change is measured in ter ms of variatio n o f diss o lved O2 concentration, consumption during the aerobic catabolism of an opportune nutrient. The concentration O2 measured is what remain s after diffusion across the thickness of the agar gel containing the immobilized cells, from the solution up to sensor’s tip. The difference between the O2 concentration in the solution and the O2 measured by the sensor is the O2 consumed during cellular respiration. A typical kinetic curve (ppmO2 vs time) of cellular respiration rec- orded during the test is reported in Figure 1. Measure ments are perfor med in an open system at roo m temperature
 R. Dragone et al. Figure 1. Typical experimental respirometric kinetic curve: analytical parameters are Δppm (O2)I-II and Δppm (O2)I-III, where Δppm (O2)I-II is the variation of O2 consumption between steady-state I and II and Δppm (O2)I-III is the variation of O2 consumption between steady- state I and III. (25.0˚C ± 0.1˚C), and the d issolved O2 remains constant in the solution (no significant variation of PO2 atmo s- pheric d uring the time te st). Sensor calibration is performed daily by measuring O2 in open air (~21% v/v; the values of ppmO2 in water are reported in an apposite table in function of P and T) and in a 10 g/L sodium sulfite solution (zero point cali- bration). After calibration the sensor is coupled with the agar disk and the biosensor is merged in a phosphate buffer (pH = 7.50 ± 0.01). Reached signal stability (fluctuation ≤ 0.01 ppm within 15 minute s), t he O2 concen- tration is ca. 7. 00 ppm (T = 25.0˚C ± 0.1 and P(barometric pressure) = 760 ± 5 mmHg). The respiratory activity of the yeast cells under these conditions is due only to basal metabolism. The addition of the glucose solution (final concentration 0.5 mol /L) implies an increase in respiratory metabolism from the basal to the maximum level. The rate of cellular O2 consumption increases, dissolved O2 concentration, measured after the passage through the agar disk, decreases until a plateau (steady-state I, fluctuation ≤ 0.05 ppm within 15 minutes). At steady-state I the rate of O2 diffusion inside the disk balances ce llular O2 consumption. At steady-state I , the chemical is added: if it exerts an in hibitory effect on cellular respirato ry activity, the O 2 consumption decreases and dissolved O2 concentration rises until the second plateau (steady-state II, fluctuation ≤ 0.05 ppm within 15 minutes). To completely inhibit cellular respiration, 100 μL of sodium azide 0.02 mol /L is added. The signal increases to a third plateau (steady-state III, fluctuation ≤ 0.05 ppm within 15 minutes). The analytical parameter calculated from Δppm(O2)I-II and Δppm (O2)I-III (Figure 1) is a p ercentage index ex- pressed as Inhibition of Respiratory Activity (IRA) [8]: ( )( ) 22 I III III IRA index=ppm Oppm O100 −− ∆∆ × The chemicals were tested each at 3 concentrations (ranging 0.20 - 0.42 % (v/v)). All experiments were per- formed in quadruplicate and the arithmetic means were calculated; repeatability was expressed as relative stan- dard deviation (RSD %). 2.2.2. Improvements in Sensitivit y: Lysis of the Cellular Wall For the cell ular wall l ysis, yea st cells were treated with the lytic enzyme zymolyase. It is a comple x e nz yme tha t
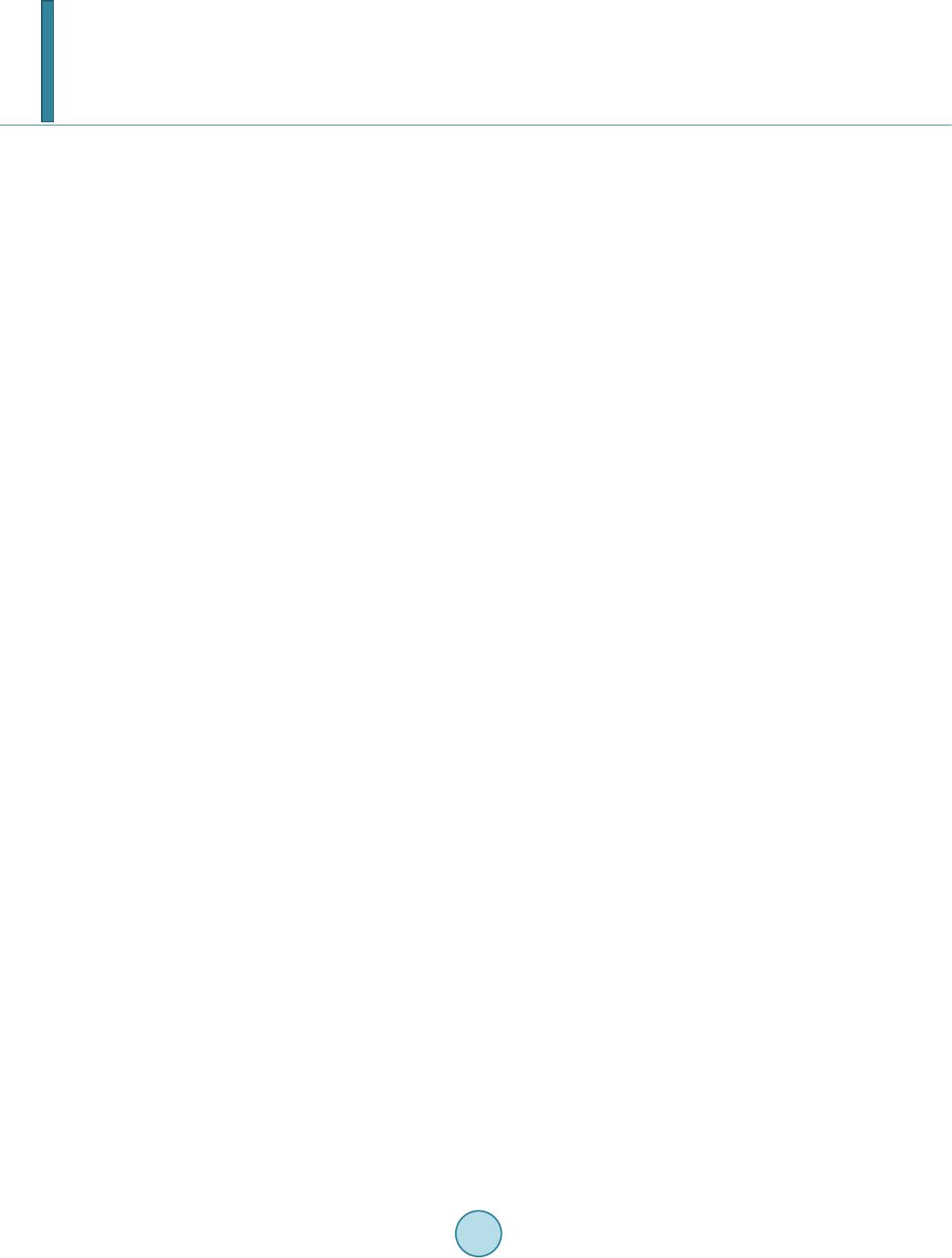 R. Dragone et al. posses β-1,3 glucanase and β-1,3-glucan laminaripentao-hydrolase activity, which hydrolyze glucose polymers at the β-1,3-glucan linkages releasing laminaripentaose as the principal product. Zymolyase also has alkaline protease activity. Cells were l eft in incubatio n (Petri d ish) with the enzy me solution for 2 ho urs at room temper- ature (25.0 ± 0.1˚C). Incubation conditions have been selected by testing different incubation times, and choos- ing the one s triki ng bo th quality result and measurement time. The proper concentration of enzyme was selected depending on the number of cells in the disk. The incubation solution was prepared by a solution of sorbitol 0.5 mol /L with p ho sphat e b uffer 0.06 mol /L at pH = 7.50, and 0.5 mg of Zymolyase. Sorbitol was used as compatible solute, in such concentration to avoid ly- sis of the cell plasma membrane, due to the different osmotic pressure between internal and external of cells. After the fixed ti me the disks are washed with same solution of sorbitol 0.5 mol /L in b uffer . This wash wa s ne- cessary to remove the enzyme and its possible interference with the measurement. Respect to the methodology already established [1,2,5,9,10] other variations have been introduced: 1) Using the serial dilution plate technique, cells counting was performed on Petri dishes with culture media of different composition (Table 1). The composition allowing obtaining a number of cells suitable for respiro- metric test was selected. U nde r substrate excess working conditions, the excessive number o f cells would exces- sively lower re sidual dissolve d O2 concentration at steady-state I ; this condition would not allo w a correct inter- pretation of the results and calculation of the IRA index. 2) Formaldehyde has been replaced by sodium azide as 100% respiration inhibitor. Comparative respirometric tests were performed by adding at the steady-state I formaldehyde 37% (13 mol/L) or different aliquots of so- dium azide 0.02 mol / L until increase to steady-state III. The variation of the dissolved O2 (Δppm O2) between the two steady-states was calculated and results are summarized in Table 2 . Results ob tained from co mparative respirometric tests show that Δpp m O2 obtained by adding already 50 μL of sodium azide 0.02 mol / L are com- parable to those obtained by addition of 500 μL of formaldehyde 13 mol/L. Therefore, sodium azide can replace formaldehyde (volatile chemical classified as carcinogen) [11] as respiration inhibitor in respirometric tests, with the adva ntage of handling l ess amount o f a safer sub s tance. 3. Results and Discussion The biosensor based on intact yeast cells was exposed to SDS, Hg2+ and 4-CP (Ta ble 3 ). For all t he substa nces, IRA index increas es in dose dependent fashion. The higher values of IRA index were found for Hg2+ followed by SDS a n d 4-CP. The biosensor based on intact yeast cells was also exposed to wood preservatives formulations (Tab le 4) . T he higher value o f IRA index (97%) found for CX-10 a t 4.7% (v/v) compared to same conc entration of the others is explai ned by its known efficient ki ll of certain microorganisms, including S. cerevisi a e [12]. For ACQ, CCA-C, and T analith E at the lo west c oncen trati on (0 .25% (v/v)) the I RA ind ex was undetectable, probably because at this concentration these substances are unable to pass the cell wall and act on mitochondrial function. The reason may be still valid for Tanalith E at 2.4% (v/v) and therefore for this substance we tested a concentration point more. The same methodology, exposure of modified yeast cells, was applied to a selection of chemicals: Hg2+ as more reactive agent on the system, and CCA-C. The onl y pro ced ural c hange was t he inc ub atio n ti me of disk s (2 hours) with Zymolyase before assembling the biosensor. Results (Table 5) shows how the lysis of the cellular wall 1) increases the IRA index at all concentrations of all substances tested, up to 33%; 2) allows detection at lower conce ntrations. In parti cular, the resu lt of CCA-C at 0.25% (v/v) is significant. The RSD% (from 4 repli- cate analyses) ranged 3 to 7%. The metal cations (e.g. Hg2+) deserve some separate reflections. S. cerevisiae is capable of non-selective ac- cumulation mechanis m of cations; both intact yeast cells and/or isolated cell wall components sho w such chela- tion based cations retention [13]. Cell wall is structured as a β 1,6 and β 1,3 glucans chains network (55% - 65%) closely associated with chitin (1% - 2%), and mannoproteins (35% - 40%) [14]. All these cell wall co mponents possess several binding sites (e.g. carboxyl, hydroxyl, amino, or imidazole groups) that could facilitate physic- chemical interactions between the cell wall and the cations [15,16] and could therefore slow down the in- tra-cellular passage. The second step of yeast uptake of metal cations involves the transport across the cell membrane through different transport mechanisms (e.g. diffusion or active transport) [17]. Probably in conse- quence of the partial wall removal, the diffusion of the cations within the cell is not hampered, thus leading
 R. Dragone et al. Table 1. Cells counting by serial dilution plate technique; a: Traditional, b: Alternative; RSD% ≤ 3 on 4 replicates.. Culture medium compositio n CFU/mL (106) a) yeast extract, 1%; pep tone , 1%, glucose, 2% 50.28 b) yeast ext ract, 0.17%; pep tone , 0.17% , glucose, 0.30% 9.77 Table 2. Comparison of respiration inhibitors; RSD% ≤ 10 on 4 replicates. Chemical Aliquot (µL) Δppm (O2) Formaldehyde (13 mol/L) 500 5.78 Sodium Azide (0. 02 mol/L) 100 200 500 5.82 5.86 5.95 Table 3. IRA index %; RSD% ≤ 6 on 4 replicates. IRA index % Chemical Concentration (%(v/ v)) 0.20 0.30 0.42 Hg(NO3)2 53 67 92 4-CP 20 26 32 Table 4. IRA index %; RSD% ≤ 7 on 4 replicates; ud = undetect abl e: nd = not determined. IRA index % Chemical Concentration (%(v/ v)) 0.25 2.4 4.7 9.0 (Wolmanit) CX-10 88 93 97 nd ACQ ud 22 79 nd CCA-C (Tanalith C) ud 63 66 nd Tanalith E ud ud 42 93 Table 5. Comparison of different biomediators: IRA index % obtained with intact and modified yeast cells; ud = undetec- table; RSD% ≤ 7 on 4 replicates. Chemical Concentration IRA index % Intact cells IRA index % Modified cells CCA-C 2.4% (v/v) 1.5% (v/v) 0.25% (v/v) 63 61 ud 84 80 34 Hg 0.42% (v/v) 0.30% (v/v) 0.20% ( v/v) 92 67 53 98 81 69 to the highe r sensit ivity of the mod ified yeast cells. 4. Conclus ions Aerobic respiration of S. cerevisiae is a rapid and useful toxicological endpoint. The simple treatment of the immobilized yeast cells dis k with Zymolyase allo ws preparation of a more sensitive bio mediator, still maintai n- ing acceptable rapidity of the test. The proposed methodology’s improvement allows gaining in sensitivity, but the use of intact yeast cells al- lows working with solutions with different osmolarity (from distilled water to glucose 1M) without cell death due to lyses of cell plasma membrane. The proper design of the biosensor depends on the intended application.
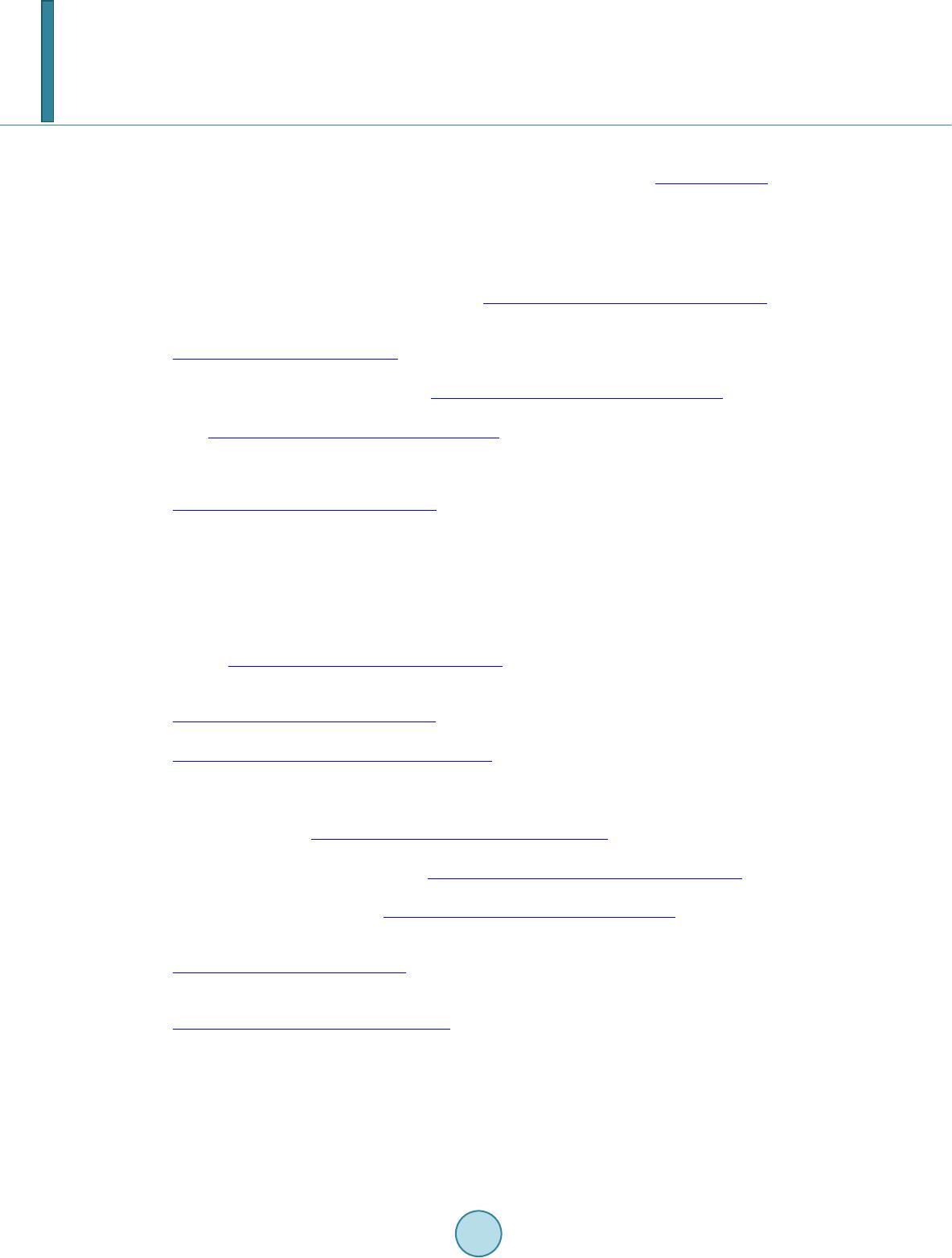 R. Dragone et al. Acknowledgements The work has been carried out in the fra me of activities of the ALE RT pr oject (www.alert2015 . it) fund ed by the Italian Ministry of economical development under the Call Industria 2015 “New technologies for Made in Ita- l y” . References [1] Campanella, L., Favero, G. and Tomassetti, M. (1995) Immobilised Yeast Cells Biosensor for Total Toxicity Testing. The S cience of the Total Envir onment, 171, 227-234. http://dx.doi.org/10.1016/0048-9697(95)04673-0 [2] Haub enstr icker, M. E., Meier, P.G., M ancy, K.H. and Br abec, M.J. (1990 ) Rapid Toxicit y Testing Based on Yeast Res- piratory Activity. Bulletin of Environmental Contamination and Toxicology, 44, 669-674. http://dx.doi.org/10.1007/BF01701786 [3] Campanella, L, Favero, G., Mastrofini, D. and Tomassetti, M. (1997) Further Developments in Toxicity Cell Biosen- sors. Sensors and Actuators B, 44, 279-286. http://dx.doi.org/10.1016/S0925-4005(97)00219-0 [4] Dragone, R. and Grasso, G. (2012) Biosensoristic Devices: Monitoring and Diagnostics in Ag ro -Zo otechn ical P roduc- tions. http://www.iss.it/binary/publ/cont/12_49_web.pdf [5] Frazzoli, C., Dragone, R., Mantovani A., Massimi, C. and Campanella, L. (2007) Functional Toxicity and Tolerance Patterns of Bioavailable Pd(II), Pt(II), and Rh(III) on Suspended Saccharomyces cerevisiae Cells Assayed in Tandem by a Resp irometric Biosensor. Analytical and Bioanalytical Chemistry, 389, 21 8 5-2194. http://dx.doi.org/10.1007/s00216-007-1623-2 [6] Necas, O . (1971) Cell Wall Synthesis in Yeast Protopl asts. Bacteriological Reviews, 35, 149. [7] Skaalure, S.C., Oppegard, S.C. and Eddington, D.T. (2008) Characterization of Sterilization Techniques on a Micro- fuidic oxygen deli very device. Journal of Undergraduate Researcher, 2, 1-5. [8] Campanella, L., Dragone, R. and Favero, G. (2000) Method to Determine the Toxici ty Cau sed by Exposure to Chemi- cal and Physical Agents. Patent PCT Int Appl WO 00/75361 A2. [9] Dragone, R., Frazzoli, C., Grappelli, C. and Campanella, L. (2009) A New Respirometric end Point-Based Biosensor to Assess the Relative Toxicity of Chemicals on Immobilized Hu man Cell s. Ecotoxicology and Environmental Safety, 72, 273-279. http://dx.doi.org/10.1016/j.ecoenv.2008.02.011 [10] Taccari, M ., Comitin i, F. and Casucci, C . (2011) Toxicit y Assessment of Compounds in So il Using a Simple Res piro- metric Techniq ue. Intern at ional Bi ode te r i oration and Bi odegr ad a t ion, 65, 60-64. http://dx.doi.org/10.1016/j.ibiod.2010.02.008 [11] International Agency for Research on Can cer (2004) IARC Classifies Formaldehyde as Carcinogenic to Humans. http://www.iarc.fr/en/media-centre/pr/2004/pr153.html [12] Huff, J., Qureshi, S., Hodgkinson, D., Nicklin, C., Göttsche, R., Hettler, W. and Roper, D.V. (2011) Microbiocidal Compositions and Their Use. US Patent No. 7,910,569. [13] Wang, J. and Chen, C. (2006) Biosorption of Heavy Metals by Saccharomyces cerevisiae: A Review. Biotechnology advan ces , 24, 427-451. http://dx.doi.org/10.1016/j.biotechadv.2006.03.001 [14] Klis, F.M., Mol, P., Hellingwerf, K. and Brul, S. (2002) Dynamics of Cell Wall Structure in Saccharomyces cerevisiae. FEMS Microbi ol ogy Reviews, 26, 2 3 9-256. http://dx.doi.org/10.1111/j.1574-6976.2002.tb00613.x [15] Jianlong, W. (2002) Biosorption of Copper (II) by Chemically Modified Biomass of Saccharomyces cerevisiae. Process Biochemistry, 37, 847-850. http://dx.doi.org/10.1016/S0032-9592(01)00284-9 [16] Brady, D., Stoll, A., D. Starke, L. and Duncan, J. R. (1994) Chemical and Enzymatic Extractio n of Heav y Metal Bind- ing Polymers from Isolated Cell Walls of Saccharomyces cerevisiae. Biotechnology and Bioengineering, 44, 297-302. http://dx.doi.org/10.1002/bit.260440307 [17] Pócsi, I. (2011) Toxic Metal /Met alloid Toleran ce in Fungi—A Biotechnology-Oriented Approach. In: Bánfalvi, G., Ed., Cellular Effects of Heavy Metals, Springer Scien ce+B usiness Media, 31-58. http://dx.doi.org/10.1007/978-94-007-0428-2_2
|