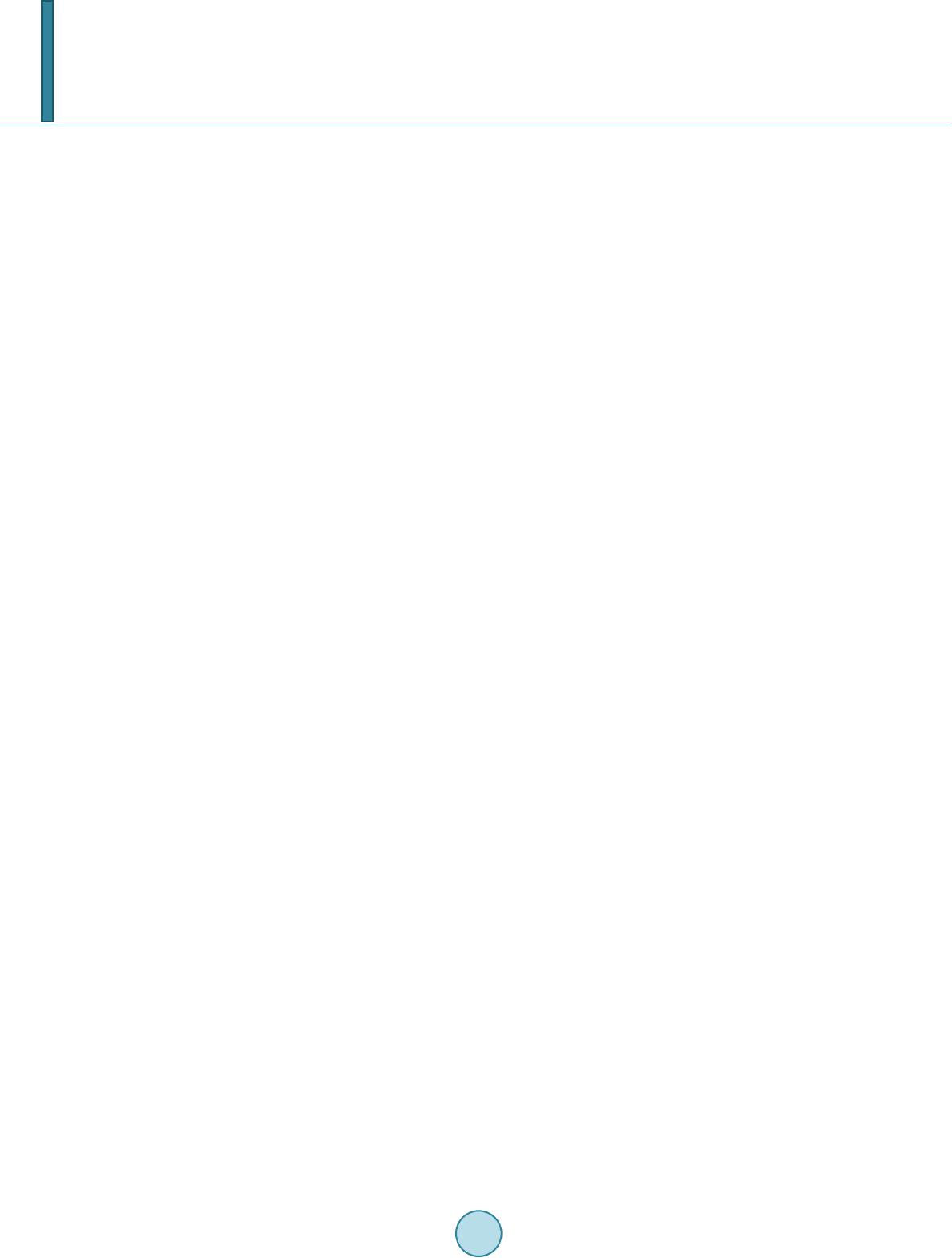
H. M. M. Mansour, N. Bakheet
Software (CMSSW) via associated package developed by the CMS PH Generator group. In the current work all
the produced results are via using Monte Carlo (MC) programs, FEYNRULES program for the implementation
of the new model beyond the Standard Model. Our model here will be the B-L model which is based on a La-
grangian for calculation of Feynman rules in momentum space for any QFT physics mode. We also use
PYTHIA8 event generator for proton-proton collision and partons showers (PS), MadGraph5/Madevent is a
Matrix Element Monte Carlo Generator, CALCHEP for computation of Feynman diagrams and for effective
evaluation and simulation of high energy physics collider processes at parton level, COMPHEP is a Matrix
Element Monte Carlo (MC) Generator (MEG). As for MEG, it generates partons based on a Lagrangian also the
programs ROOT, Physical Analysis Works station (PAW) and MADANALYSIS are used for physical analysis
and to draw the results, in addition to Mathematica, C++, FORTRAN and Python programming languages, all
this works on Scientific Linux. Does the Standard Model of particles physics need an extension? The standard
model of electroweak and strong interactions is once again going to be severely tested as well as many of its ex-
tensions that have been proposed to cure its flaws. The observed pattern of neutrino masses the existence of dark
matter and the observed matter-antimatter asymmetry are the most severe evidences where the SM fails to ex-
plain. It is widely accepted that the SM ought to be extended but no one knows if the proper way has already
been explored in the literature. A joint collaboration is therefore needed between the experimental and the theo-
retical communities. The aim of this work has been guided by these principles. The aim is to fill some of the
gaps in the overall preparation towards real data, as well as to interact with the experimentalists. An extension of
the SM has been systematically studied, from the definition of its parameter space to the collider signatures,
leading to some new and exciting possibilities for the LHC to shed light on. Within the ambition of a complete
study, in all its aspects, the experimental help has been fundamental to efficiently concentrate on aspects of ac-
tual interest in a way which is useful for both communities. The main motivations for the extension of the SM
that we will describe concern the lack of a natural explanation for the observed pattern of neutrino masses, the
unknown origin of a global and not anomalous accidental U(1) symmetry in the SM (related to the baryon mi-
nus lepton (B-L) quantum numbers), and the absence of any observation of a fundamental scalar degree of free-
dom (the Higgs boson). B-L model is a triply-minimal extension of the SM. It is minimal in the gauge sector, in
which a single U(1) factor is added, related to the B-L number, by simply promoting to local the already existing
U(1) B-L global symmetry of the SM. It is minimal in the fermions sector, in which a SM singlet fermions per
generation. These fermions can naturally be interpreted as the Right Hand neutrinos (RH). It is minimal in the
scalar sector, in which a complex neutral scalar singlet is added to spontaneously break the new U(1) symme-
try, and at the same time to give to the new gauge boson a mass. The two latter points, once the U(1) symmetry
is spontaneously broken; naturally provide a dynamical implementation of the see-saw mechanism explaining
the neutrino masses. As we will see, the remnant degree of freedom of the new complex scalar severely im-
pinges in the phenomenology of the scalar sector. The general model we introduce is a one-dimensional class of
U(1) extensions of the SM, in which each element is characterized by the properties of the new gauge boson as-
sociated to the extra U(1) factor. The “pure” B-L model is identified by the fact that the extra gauge boson, or
Z′B-L, couples to fermions proportionally to their B-L number only. On the one side, this directly implies a
vanishing of Z-Z′ mixing (at the tree-level), that is consistent with the existing tight constraints on such mixing,
compatible with a negligible value. Moreover, the B-L charge does not distinguish the chirality, i.e., the LH and
the RH degrees of freedom of the same fermion which has the same B-L quantum numbers. It is important to
emphasize that this is a TeV scale extension of the SM. This means that the U(1) B-L breaking vacuum expecta-
tion value (VEV) is of O (TeV). Hence, the new particles will have masses at the TeV scale. We are mainly
concerned with the impact of the model at Large Hadrons Collider, on which the above phases do not play any
role. We will show that the Z′ new neutral massive boson in this model, with TeV scale heavy neutrinos, can
decay into pairs of the heavy neutrinos. The presence of new coupled matter, the heavy neutrinos, has important
phenomenological consequences. The possibility of the Z′ boson (and of the Higgs bosons, as we will show) to
decay into pairs of them, will provide new and exciting signatures. It is worth to briefly mention that the peculiar
decays of the Higgs bosons into pairs of heavy neutrinos, or into pairs of Z′ bosons, is a distinctive signature of
this model, offering the chance to distinguish it from the plethora of the otherwise identical, concerning the sca-
lar sector, singlet extensions of the SM in the literature. We will consider all the heavy neutrinos as degenerate
and with masses that are free parameters. In this work we study the details of the gauge and fermion sectors.
Their mutual interactions are fully included in the Z′ decay into pairs of heavy neutrinos. Altogether, this decay
provides new and spectacular multi-lepton signatures of the Z′ boson. One of the main results of this work is the
study of one of them, the tri-lepton decay mode (i.e., when the Z′ decays into exactly 3 charged leptons and