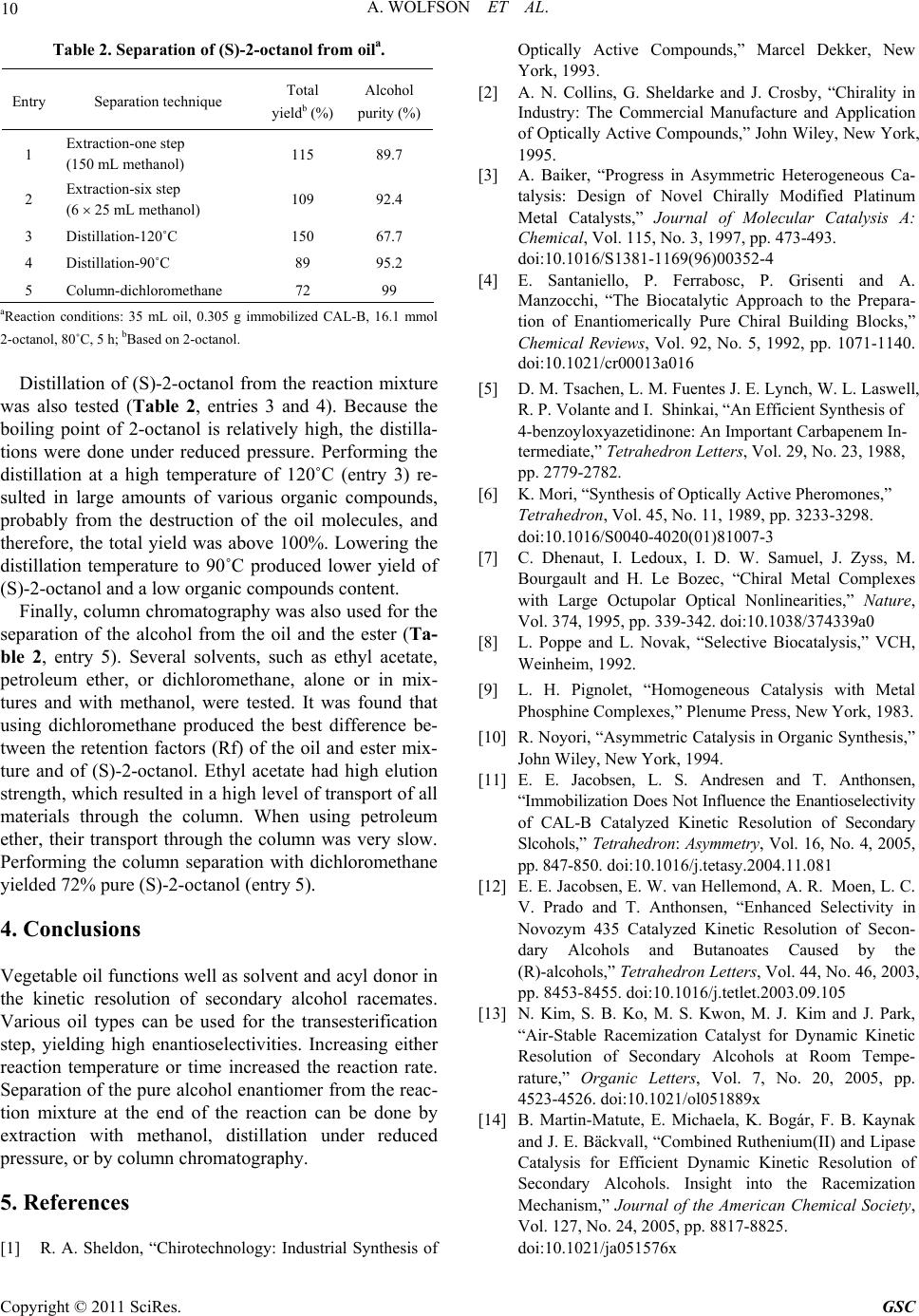
A. WOLFSON ET AL.
10
Entry yield (%)
hol
purity (%)
Table 2. Separation of (S)-2-octanol from oila.
Separation technique Total
b
Alco
1 Extr
(150 mL methanol)
action-one step 115 89.7
2 Extraction-six step
(6 25 mL methanol)
4 89 95.2
109 92.4
3 Distillation-120˚C 150 67.7
Distillation-90˚C
5 Column-dichloromethane 72 99
aReon il, 0.305 g immobi CAL-B,
2-ocol, l.
rom the reaction mixture
as also tested (Table 2, entries 3 and 4). Because the
bo
Ta-
bl
ons well as solvent and acyl donor in
e kinetic resolution of secondary alcohol racemates.
, “Chirotechnology: Industrial Synthesis of
Optically Active Compounds,” Marcel Dekker, New
[2]
ommercial Manufacture and Application
Design of Novel Chirally Modified Platinum
and A.
ch to the Prepara-
ai, “An Efficient Synthesis of
l. 45, No. 11, 1989, pp. 3233-3298.
, M.
tal Complexes
xes,” Plenume Press, New York, 1983.
ence the Enantioselectivity
ectivity in
Kinetic
enium(II) and Lipase
acticonditions: 35 mL olized 16.1 mmol
tion of Enantiomerically Pure Chiral Building Blocks,”
Chemical Reviews, Vol. 92, No. 5, 1992, pp. 1071-1140.
tan 80˚C, 5 h; bBased on 2-octano
Distillation of (S)-2-octanol f
w
iling point of 2-octanol is relatively high, the distilla-
tions were done under reduced pressure. Performing the
distillation at a high temperature of 120˚C (entry 3) re-
sulted in large amounts of various organic compounds,
probably from the destruction of the oil molecules, and
therefore, the total yield was above 100%. Lowering the
distillation temperature to 90˚C produced lower yield of
(S)-2-octanol and a low organic compounds content.
Finally, column chromatography was also used for the
separation of the alcohol from the oil and the ester (
e 2, entry 5). Several solvents, such as ethyl acetate,
petroleum ether, or dichloromethane, alone or in mix-
tures and with methanol, were tested. It was found that
using dichloromethane produced the best difference be-
tween the retention factors (Rf) of the oil and ester mix-
ture and of (S)-2-octanol. Ethyl acetate had high elution
strength, which resulted in a high level of transport of all
materials through the column. When using petroleum
ether, their transport through the column was very slow.
Performing the column separation with dichloromethane
yielded 72% pure (S)-2-octanol (entry 5).
4. Conclusions
Vegetable oil functi
th
Novo
Various oil types can be used for the transesterification
step, yielding high enantioselectivities. Increasing either
reaction temperature or time increased the reaction rate.
Separation of the pure alcohol enantiomer from the reac-
tion mixture at the end of the reaction can be done by
extraction with methanol, distillation under reduced
pressure, or by column chromatography.
5. References
[1] R. A. Sheldon
Cata
York, 1993.
A. N. Collins, G. Sheldarke and J. Crosby, “Chirality in
Industry: The C
of Optically Active Compounds,” John Wiley, New York,
1995.
[3] A. Baiker, “Progress in Asymmetric Heterogeneous Ca-
talysis:
Metal Catalysts,” Journal of Molecular Catalysis A:
Chemical, Vol. 115, No. 3, 1997, pp. 473-493.
doi:10.1016/S1381-1169(96)00352-4
[4] E. Santaniello, P. Ferrabosc, P. Grisenti
Manzocchi, “The Biocatalytic Approa
doi:10.1021/cr00013a016
[5] D. M. Tsachen, L. M. Fuentes J. E. Lynch, W. L. Laswell,
R. P. Volante and I. Shink
4-benzoyloxyazetidinone: An Important Carbapenem In-
termediate,” Tetrahedron Letters, Vol. 29, No. 23, 1988,
pp. 2779-2782.
[6] K. Mori, “Synthesis of Optically Active Pheromones,”
Tetrahedron, Vo
doi:10.1016/S0040-4020(01)81007-3
[7] C. Dhenaut, I. Ledoux, I. D. W. Samuel, J. Zyss
Bourgault and H. Le Bozec, “Chiral Me
with Large Octupolar Optical Nonlinearities,” Nature,
Vol. 374, 1995, pp. 339-342. doi:10.1038/374339a0
[8] L. Poppe and L. Novak, “Selective Biocatalysis,” VCH,
Weinheim, 1992.
[9] L. H. Pignolet, “Homogeneous Catalysis with Metal
Phosphine Comple
[10] R. Noyori, “Asymmetric Catalysis in Organic Synthesis,”
John Wiley, New York, 1994.
[11] E. E. Jacobsen, L. S. Andresen and T. Anthonsen,
“Immobilization Does Not Influ
of CAL-B Catalyzed Kinetic Resolution of Secondary
Slcohols,” Tetrahedron: Asymmetry, Vol. 16, No. 4, 2005,
pp. 847-850. doi:10.1016/j.tetasy.2004.11.081
[12] E. E. Jacobsen, E. W. van Hellemond, A. R. Moen, L. C.
V. Prado and T. Anthonsen, “Enhanced Sel
zym 435 Catalyzed Kinetic Resolution of Secon-
dary Alcohols and Butanoates Caused by the
(R)-alcohols,” Tetrahedron Letters, Vol. 44, No. 46, 2003,
pp. 8453-8455. doi:10.1016/j.tetlet.2003.09.105
[13] N. Kim, S. B. Ko, M. S. Kwon, M. J. Kim and J. Park,
“Air-Stable Racemization Catalyst for Dynamic
Resolution of Secondary Alcohols at Room Tempe-
rature,” Organic Letters, Vol. 7, No. 20, 2005, pp.
4523-4526. doi:10.1021/ol051889x
[14] B. Martin-Matute, E. Michaela, K. Bogár, F. B. Kaynak
and J. E. Bäckvall, “Combined Ruth
lysis for Efficient Dynamic Kinetic Resolution of
Secondary Alcohols. Insight into the Racemization
Mechanism,” Journal of the American Chemical Society,
Vol. 127, No. 24, 2005, pp. 8817-8825.
doi:10.1021/ja051576x
Copyright © 2011 SciRes. GSC