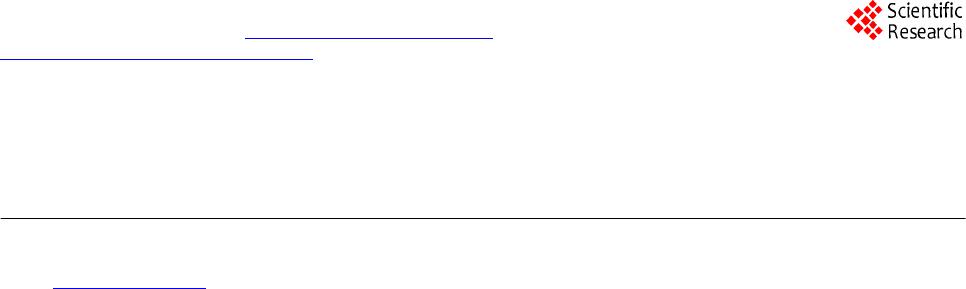 Journ al of Cosmetics, Derm atolog ical Sciences a nd Applications, 2014, 4, 24-30 Published Online February 2014 (http://www.scirp.org/journal/jcdsa) http://dx.doi.org/10.4236/jcdsa.2014.41004 Is the P hotosut uring Agent, Rose Bengal, a Mutagen? Lindsey Hunter-El lul1, Shuguang Wang2, Jeffrey Wickliffe2, Michael Wilkers on1 1Department of Dermatology, The University of Texas Medical Branch, Galveston, Texas, USA; 2Department of Global Environ- mental Heal th Sciences, School of Public Health and Tropical Medicine, Tulane University, New Orleans, Louisiana, USA. Email: mgwilker@utmb.edu Received December 11th, 2013; revised January 9th, 2014; accepted January 17th, 2014 Copyright © 2014 Lindsey Hunter-Ellul et al. This is an open access article distributed under the Creative Commons Attribution License, which permits unrestricted use, distribution, and reproduction in any medium, provided the original work is properly cited. In accordance of the Creative Commons Attribution License all Copyrights © 2014 are reserved for SCIRP and the owner of the intellectual property Lindsey Hunter-Ellul et al. All Copyright © 2014 are guard ed by law and by SCIRP as a guardian. ABSTRACT Rose Bengal (RB) is a potential photosuturing agent that may improve standard dermatologic surgical closure techniques. However, RB produces reactive oxygen species with photoactivation and its photomutagenic poten- tial must be considered in clinical application. We investigated cytotoxicity, mutagenicity, and singlet oxygen (SO) productio n of RB on epit helial Chinese ha mster ovary cell line. Cells were exposed to RB concentrations: 0.1%, 0.01%, 0.001%, 0.0001%, 0.00001%; irradiated for 400 s using a high-intensity visible wavelength lamp or maintained in the dark. Cell viability was assessed by XTT assay, mutagenicity by HPRT gene mutation assay, and SO pro duction by Sens or Green reagent . RB > 0.001% was significantly cytotoxic. Viabilities were uninflu- enced by ≤0.0001% RB controls, or 30-min incubation. 49% of irradiated cells died after 24-h in 0.0001% RB. At ≥0.001% RB, >90% of cells died. Irradiating 0.00001% - 0.001% RB inc reased SO; lev els dropped sig nificantly between 0.01% - 0.1%. Controls exhibited negligible SO production. HPRT suggested that RB was not muta- genic (0.0001%, 0.00001%); SO induction increased between 0.00001% - 0.001%, with reduced production at higher concentrations. Pilot studies suggested irradiated 0.0001% RB is mutagenic in vitro; current data suggest RB is not photomutagenic. The contribution of RB’s cytotoxicity on observed clinical improvement of scars and mutagenic potential remains unclear, necessitating further study. KEYWORDS Rose Bengal; Photochemical Tissue B onding; Mutagenicity; Cytotoxicity; Wound Healing; Dermatological Surgery 1. Introduction Wound healing and scar formation are researched exten- sively, and especially important to dermatological sur- gery. Skin incisions and excisions are closed b y a variety suture types, staples, wound closure strips, and adhesives. However, recent research shows promise in improved epidermal wound closure and healing with a p hotoche m- ical tissue bonding (PTB) agent, 0.1% Rose Bengal (RB;4,5,6,7-tetrachloro-2’,4’,5’,7’-te traiodo -fluorescein) suspended in phosphate buffered saline (PBS), when applied to wound edges and activated by green laser light [1,2]. Upon photoactivation, Rose Bengal may behave as a sort of “nanosuture,” as the dye may crosslink proteins bet wee n ti ss ues s ur fa ce s ther e b y sea li n g ski n wo und s [ 2 ]. Rose Bengal does not appear to be phototoxic in porcine skin ex vivo or rabbit skin in vivo [3]. However, pr evious studies have demonstrated that photoactivated RB ge- nerates reactive oxygen species (ROS), including singlet oxygen (1O2), which promote lipid peroxidation, DNA damage, cell membrane damage, and can cause cell death [4,5]. In this study, we investigated toxicity, mutagenici- ty, and singlet oxygen production of photoactivated Rose Bengal using epi theli al cells in cult ure. Rose Bengal, a fluorescein derivative, is a historical Ophthalmologic stain used to aid in diagnosing ocular surface disorders. RB absorbs and is excited by green wavel engths (~532 nm) leading to a tissue bonding reac- tion when appli ed to wound edges via an unkno wn mech- anism. Photoactivating RB may aid in wound closure, improve wound healing, promote skin graft adhesion, and cell viability appears unaffected [1,2,6]. There are OPEN ACCESS JC D SA
 Is the Photosuturing Agent, Rose Bengal, a Mutagen? many potential benefits of PTB, including simplicity, rapidity, and painlessness. Further, PTB could avoid su- ture removal, foreign body reactions, additional tissue injury, and infection. The first in-human clinical trial of RB-induced PTB was recently completed in 2009. Re- sults showed that PTB provided adequate tensile strength preventing wound dehiscence and less inflammation compared to sutures, and may also reduce scarring [7]. Research shows photoactivated RB’s ability to cross- link collagen scaffolds and found this is effective for producing strong, stable collagen microstructures with improved physiochemical scaffold properties [8]. PTB is hypothesized to work via a light-initiated covalent cross- linking of proteins between the wound surfaces, leading to the immediate formation of a relatively impermeable seal [7,9,10]. When excited states of the activated photo- sensitizer and photoproducts (e.g., ROS) are formed, they interact with pr oximal bio molecules (e.g., amino acids in collagen) leading to their photoexcitation. Photoexcited biomolecules can then react with adjacent side chains for ming co vale nt p rotein -protein crosslinks [11-13]. Pho- toactivated RB reaction has shown to favor the produc- tion of 1O2 (~75%), the remainder being primarily super- oxide anion radicals (20%) [14]. As a photosensitizer molecule in an aerobic environ- ment, RB ’s activatio n b y eithe r ultraviole t o r visib le li ght irradiation leads to its excited singlet state (Figure 1) [12,15]. Each photosensitizer (PS) molecule has the po- tential to generate 103 - 105 1O2 molecules prior to re- duction with 1O2 or by another reaction, thus exhausting the cycle [12]. With this capacity to regenerate until reacting with proximal biomolecules or auto-exhaustion, there is ample potential for activated photosensitizers to create harmful ROS. In patients treated with intralesional RB chemo-abla- tion for subcutaneous melanoma metastases, systemic phototoxicity has been observed [16]. Using RB on HaCat keratinocytes, the production of 1O2 has been found using a spectrophotometer in photosensitized cells. Again, RB’s potential for mutagenicity was not assessed [17]. Because RB can enter living cells and stain the nucleus, this raises questions about mutagenicity, espe- cially since studies have demonstrated that byproducts of Rose Bengal are capable of interacting with and damag- ing DNA [18,19]. This study examines the cytotoxicity and mutagenicity associated with photoactivated RB in hopes of gaining a better insight into the potential ha- zards associated with the use of RB in PTB procedures. Figure 1. Photosensitization Cycle. PS = Photosensitizer; 1PS* = PS excited singlet state; 3PS* = PS excited triplet state; hv = energy of a photon of UV or visible radiation [12]. 2. Materials and Methods Chinese hamster ovary cells (CHO), a mammalian epi- thelial cell line with fibroblast-like and epithelial-like morphology, were obtained from American Type Cell Culture (Manassas, Virginia). Fetal bovine serum (FBS), Ham’s F-12, L-glutamine, penicillin/streptomycin, 0.05% tryps in-EDTA solution, and Singlet Oxygen Sensor Green were purchased from Invitrogen (Frederick, MD). 6-thioguanine (6-TG) and 2,3-bis[2-Methoxy-4-nitro-5- sulfophenyl]-2H-tetrazoliu m-5-caboxyanilide (XTT) were purchase from Sigma-Aldrich (St. Louis, MO). Phos- phate buffered saline (PBS) and methanol were pur- chased from Fisher Scientific (Houston, TX). Crystal violet was purchased from Acros Organics (Geel, Bel- gium). The high-intensity visible light (81,500 lux; Mas- tech Digital 4-Range 200,000 Lux Meter, Model: LX1330B) with a 65 Watt fluorescent bulb was pur- chased from a hardware store. 2.1. Cell Culture and Treatment CHO cells were cultured in Ham’s F-12 medium sup- plemented with 10% FBS, 1% L-glutamine, and 1% pen- icillin/streptomycin in 25 cm2 culture flasks in a dark, humidified incubator at 37˚C with 5% CO2. Cells were maintained under exponential growth conditions and RB suspended in Ham’s F-12 was added to control and RB flasks at the time of light irradiation, creating 0%, 0.1%, 0.01%, 0.001%, 0.0001%, or 0.00001% RB concentra- tions. All exposure groups were maintained in duplicate cult ures a nd hand led i n dar k lightin g, avoid ing R B photo- activation. 2.2. Light Irradiation Cells were suspended in Ham’s F-12 with RB and irra- diated for 400 seconds to high-intensity visible light (~400 - 700 nm), using a 65 Watt fluorescent bulb (T- max 33.9˚C at 400 seconds) by placing culture flasks on glass 3 cm above the bulb [15]. Controls remained in the dark. 2.3. Cell Viability Trypan Blue staining was used for initial cell counts to assist in calculating appropriate cell quantities necessary for each of the assays used. Cell viability was assessed using the XTT assay (TOX2) according to the manufac- turer’s protocol (Sigma-Aldrich), to assess mitochond rial dehydrogenase activity. After 30 minutes or 24 hours post light irradiation, 20 μL of activated XTT solution was added to the cells, then incubated for 3 hours. Six replicates for each exposure concentration were ex- amined. Background absorption was eliminated by cen- trifuging plates at 200× g for 5 minutes and supernatant was transferred to a new plate. Absorbance at 450 nm PS hv 1PS* 3PS*PS + O2(1∆g) O2 OPEN ACCESS JC D SA
 Is the Photosuturing Agent, Rose Bengal, a Mutagen ? (corrected at 690 nm) was measured using a microplate spectrophotometer (Bio-RAD Benchmark Plus). 2.4. HPRT Gene Mutation Assay The HPRT mutation assay and methods for calculating plating efficiency and mutation frequencies followed Gupta and Singh’s technique [20]. The assay was re- peated for each test condition 3 separate times, using 3 plates to determine plating efficiency and 6 plates for 6TG resistance. Cells were maintained in the dark incu- bator for 5 days to select for mutant colonies, replacing complete medium once on day 3 of incubation. On day 5, medium was removed, cells were rinsed with PBS, stained with Cresyl violet, and colonies were counted. 2.5. Singlet Oxygen Production Test concentrations of RB (0%, 0.1%, 0.01%, 0.001%, 0.0001%, or 0.00001%) were suspended in Ham’s F-12 or PBS. 10 μL of 5 mM Sensor Green (SG) reagent was added to 90 μL of the range of RB solutions, and 100 μL of RB-SG solution were pipetted into 96-well tissue cul- ture plates, 3 replicates for each concentration with and without light irradiation. Controls were covered with aluminum foil d uring and afte r light irradia tion, avoiding irradiation. The F-12/SG and PBS/SG plates were irra- diated for 0, 200, or 400 seconds. Specimens were eval- uated for ROS using a fluorescence microplate reader (Fluostar Optima, BMG Labtech) at the following set- tings: Excitation 485 nm, Emission 520 nm, Position 3, “Fluoresc ence.” 2.6. Data Analysis Dose-response relationships for the examined endpoints were statistically tested using a general linear model (univariate analysis of variance procedure) in SPSS ver- sion 16.0 for Windows. Post-hoc mean comparisons were conducted using a Bonferroni correction for multiple comparisons. P-value <0.05 was used to determine sig- nificance. 3. Resul ts 3.1. XTT Assay Viability was unaffected at 0.0001% RB in Ham’s F-12 with and without light irradiation after 30 minutes of incubation (Figure 2). Nearly 45% of cells were lost after light irradiation and 24 hours of incubation with 0.0001% RB (Figure 3). Over 90% of cells died when exposed to 0.001% up to 0.1% RB in Ham’s F-12 re- gardless of light irradiation, after 30 minutes and 24 hours of incubation. There appeared to be significant dose-dependent negative effects (p < 0.01) in viabilities. Figure 2. Cell viability was determined by the XTT assay after 30 minutes of incubation. The results were expressed as a perce ntage of t he readi ng in control w ells. The mean ± SD are from two independent exper iments. Figure 3. Cell viability was determined by the XTT assay after 1440 minutes (24 hours) of incubation. The results were expressed as a percentage of the reading in control wells. The mean ± SD are from two independent experi- ments. 3.1.1. No Light, 30 Minutes Incubation There was no difference between the control and the lowest dose (0.0001% RB); however, comparing these to the higher concentrations in this group, controls and 0.0001% RB were significantly different from all other concentrations (p < 0.01 ), with viabilities greatl y reduced in the latter concentrations. There were no significant differences amongst other concentrations. 3.1.2. Light, 30 Minutes Incubation Simi lar fi ndings o ccurr ed wit hin this group a s in the “No Light” group above. There was no difference between the control and the lowest dose (0.0001% RB). Comparing these concentrations to higher concentrations in this group, controls and 0.0001% RB were significantly dif- ferent from all other concentrations (p < 0.01), with greatly reduced viabilities in the latter. There were no significant differences a mong st other concentrations. 3.1.3. No Light, 1440 Minutes (24 Hours) of Incubation Similar findings occurred here as in the “No Light” and OPEN ACCESS JC D SA
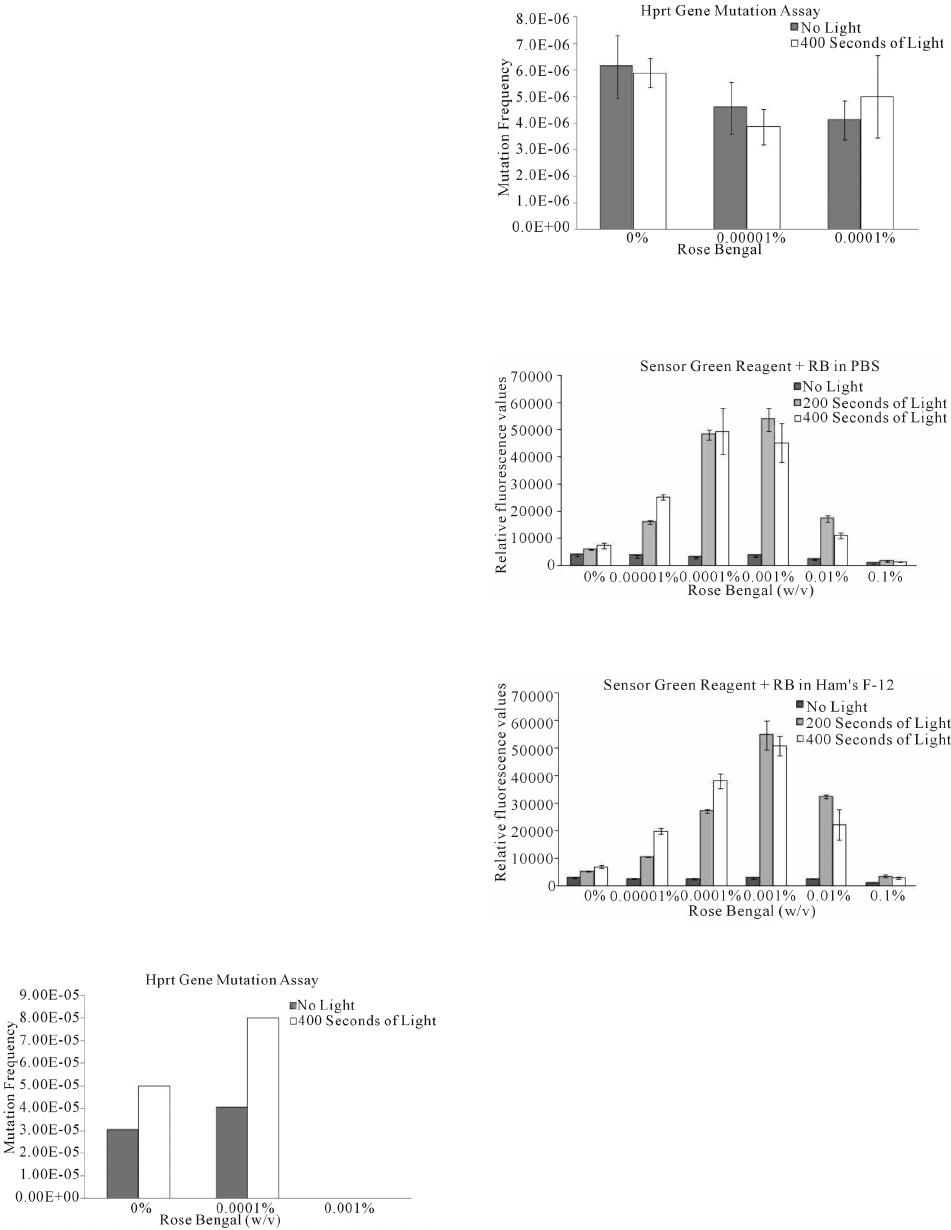 Is the Photosuturing Agent, Rose Bengal, a Mutagen? “Light, 30 Minutes of Incubation” groups in F igure 2. There was no difference between controls and lowest dose (0.0001% RB), however, comparing these to the remaining concentrations in this group, controls and 0.0001% RB were significantly different from all other concentrations (p < 0.01) with greatl y reduced viabilitie s in the latter concentrations. There were no significant differences amongst other concentrations. 3.1.4. Light, 1440 Minutes (24 Hours) of Incubation Controls were significantly different from all other con- centrations (p < 0.01), with viabilities showing a slight increase after irradiation with light. Additionally, the lowest dose (0.0001% RB) differed from all other con- centrations (p < 0.01), where the irradiated group had nearly 50% reduction in viability compared to the group without irradiation. There was no difference amongst all other concentrations. 3.2. HPRT Gene Mutation Assay The initial HPRT gene mutation assay revealed that irra- diating cells with 0.0001% RB suspended in Ham’s F-12 was likely mutagenic, with roughly a doubling of the mutant frequency above background (Figure 4). A repeat study including 0.00001% RB did not appear to be mu- tagenic at 0%, 0.00001%, or 0.0001% RB in Ham’s F-12 regardless of irradiation status (Figure 5). Higher con- centrations were cytotoxic and the assay was not per- formed. 3.3. Singlet Oxygen Production The Sensor Green Assay detected 1O2 production by RB in PBS (Figure 6) or Ham’s F-12 (Figure 7) at varying concentrations with and without light irradiation for 0, 200, or 400 seconds. 3.3.1. Sensor Green Assay in PBS Buffer 1) PBS, No Light Controls, 0.00001%, 0.0001%, or 0.001% RB did not Figure 4. Mutation frequencies at the hgprt locus in CHO cells induced by 0%, 0.0001%, and 0.001% RB suspended in Ham’s F-12 with a nd without light irradiation [21]. Figure 5. Mutation frequencies at the hgprt locus in CHO cells induced by 0%, 0.00001%, and 0.0001% RB sus- pended in H am’s F-12 with and without light irradiation. Figure 6. Fluor esce nce int ensity of 1O2 measur ed by Se nsor Green Reagent : RB suspended in PBS. Figure 7. Fluor esce nce int ensity of 1O2 measur ed by Se nsor Green Reagent: RB suspe nded in Ha m’s F-12. differ from one another, but were found to be signif- icantly higher than the two highest concentrations, 0.01 and 0.1% RB (p < 0.01). 0.1% RB was significantly dif- ferent from all other concentrations (p < 0.02), with nearly undetectable levels of 1O2 species. 2) PBS, 200 Seconds of Light Controls had significantly lower amounts of 1O2 pro- duction when compared all other concentrations (p < 0.01), except for the highest concentration, 0.1% RB. Singlet oxygen production for controls and 0.1% RB remained quite low, whereas from 0% to 0.001% RB the levels of 1O2 increased. Levels of 1O2 production were greatly reduced from 0.001% to 0.01% RB. OPEN ACCESS JC D SA
Is the Photosuturing Agent, Rose Bengal, a Mutagen ? 3) PBS, 400 Seconds of Light Controls had significantly lower amounts of 1O2 pro- duction from all other concentrations (p < 0.01), except for the two highest concentrations, 0.01% and 0.1% RB. Levels of 1O2 production increased from 0% to 0.001% RB, but there was a decrease in 1O2 production between 0.001% and 0.1% RB. 3.3.2. Sensor Green Assay in Ha m’s F-12 Medium 1) F-12, No Light Similar findings as in the PBS, “No Light” group above were discovered. 0.1% RB concentration was sig- nificantly different from all groups (p < 0.02), with near- ly undetectable levels of 1O2 molecules. 2) F-12, 200 Seconds of Light Controls produced low levels of 1O2 species, and were not significantly different from 0.00001% or 0.1% RB. Most of the remaining concentrations significantly dif- fered from one another (p < 0.01), with increased 1O2 production from 0% to 0.001% RB, and decreased de- tectable 1O2 from 0.001% to 0.1% RB. 3) F-12, 400 Seconds of Light There was an increase in 1O2 production from 0% to 0.001% RB, and reduced detection of 1O2 levels from 0.001% to 0.1% RB. Controls produced low amounts of 1O2 and were not statistically different from 0.1% RB, but were significantly different from all other concentra- tions (p < 0.01). All other concentrations were signifi- cantly different from one another (p < 0.01). 4. Discussion Finding improved wound closure techniques with few side effects is an important topic [22-24]. RB has only recently been investigated as a PTB agent [1,2,7]. It was initially thought to be harmless and unable to penetrate vital cellular o r ganelles, however, studies show RB stains nuclei and other e ssential organelles, i.e., in huma n inte s- tine smooth muscle cells (HISM) [18]. Additionally, photoactivated RB generates ROS and has been used to induce strokes in mice [17,25]. ROS are important for many normal physiological processes, but high levels cause DNA and cell membrane damage, which can be cytot oxic. S tudie s have i nvest igated RB’s RO S induct ion, cytotoxicity, staining of cellular organelles, and histo- logical and macroscopic outcomes of PTB, but few ad- dress the issue of photoactivated RB-induced mutagenic- ity [3]. Both UVA and chemical- or photochemically- induced formation of 1O2 exhibit the ability to modulate gene expression, enzyme induction (i.e., collagenases, and intercellular adhesion molecule-1) [26]. Chemical- or photochemically-induced formation of 1O2 appears to have effects si milar to those o f UVA radiation, incl uding activation of c-Jun amino-terminal kinases (JNK) in hu- man skin fibroblasts [15]. 1O2 has been implicated in al- tered dermal states arising from abnormal collagen cross- linking from 1O2 targeting histidine and histidinohydrox- ylsino no r le uc ine via p ho to o xid a tio n i n bo vine sk in wh ic h may reduce tensile properties [27]. Reducing incubation from 5 minutes to seconds, there is rapid dye uptake by stained cell membranes and nuc- leus in HISM cells with 0.1% RB. Cells incubated for longer periods of time have reduced intracellular fluo- rescence emission, likely a result of the dye’s self- quenching effect. Regarding RB’s intrinsic toxicity, morphologic changes were exhibited in four cell types (rabbit tendon fibroblasts, cells from bovine pulmonary artery, HISM, and rabbit corneal epithelial cells) in the presence of varying concentrations in the dark and am- bient lighting, where higher (1%) concentrations of RB appear to cause cell detachment and loss, and lower (e.g., 0.01%) concentrations reduce cellular motility [18]. With the above information in mind, this study was designed to test the hypothesis that photoactivated RB induce s RO S (name ly 1O2) at low concentrations reduces cell viability and increa ses genotoxicit y in epithelial cells. The range of RB concentrations selected increased from 0 to 0.1% RB (concentration recently investigated in human subjects) [1,2,7]. Light irradiation exposure time s included 0, 200, and 400 seconds (double the length of time in recent research) to compensate for the lack of a source of concentrated green wavelength. Having piloted a study where cultures exposed to 0% and 0.1% RB in PBS, PBS was itself cytotoxic within 24 hours. As a re- sult, we suspended RB in Ham’s F-12, as this was not overtly detrimental to viability. Green light minimally penetrates the epidermis in animal models, which should thereby localize photodynamic therapies to the epidermis [28]. We irradiated cells with a high intensity light source, containing green wavelengths, and is also mini- mally penetrating [1 5]. RB concentrations >0.001% in Ham’s F-12 were very cytotoxic. The XTT assay revealed that viabilities were uninfluenced by low RB concentrations (i.e., ≤0.0001% RB) maintai ned in the d ar k, or a fter 30 min of inc uba tion . These values are quite low compared to concentrations used in recent human research (i.e., 0.1% RB) [2,7]. Perhaps at RB concentrations as low as 0.0001%, there is not enough 1O2 production to exhaust the cells’ innate ability to overcome the detrimental effects of ROS at these low concentrations. Time appears to play a role in the effect on viabilit y, with 49% of irradiated cells being lost after 24 ho urs of incubati on in co ncentr atio ns as l ow as 0.0001% RB. This is possibly due to an increased amount of time the cells were exposed to oxidative stress and the associated detrimental effects. At ≥0.001% RB, the effects were even more obvious with >90% of cells being lost. This supports a dose-dependent negative ef- fect on viability and a possible interaction with light and time at the lowest concentration out to 24 hours. Again, OPEN ACCESS JC D SA
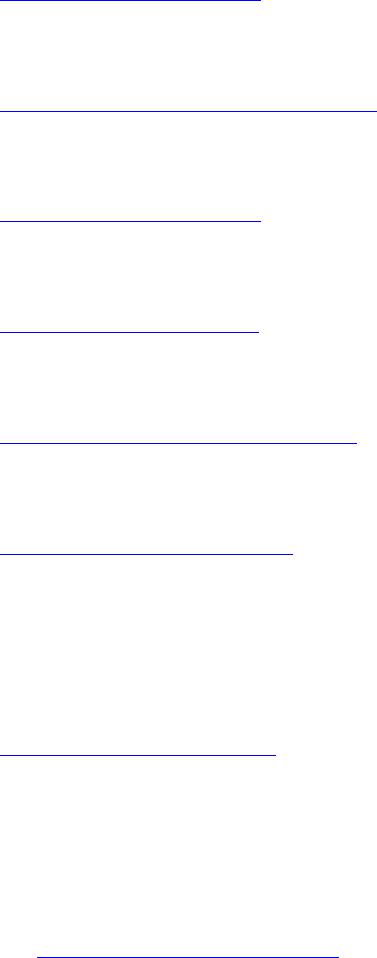 Is the Photosuturing Agent, Rose Bengal, a Mutagen? the resulting cytotoxicity from these low RB concentra- tions (e.g., 0.001%) is well below the 0.1% conce ntratio n used in clinical studies. The Sensor Green reagent revealed that irradiating 0.00001% - 0.001% RB increased amounts of detectable 1O2 species, with a significant reduction between 0.01% - 0.1%. While an increase in 1O2 production with increas- ing RB concentrations seems relatively intuitive, the data concerning 0.01% - 0.1% RB concentrations were unex- pected. Perhaps this observation can be explained by photoactivated RB’s ability to undergo auto-exhaustion during shorter periods compared to lower concentrations. Assessing 1O2 production at varying time points might be a way to investigate these observations. Alternatively, perhaps higher concentrations of RB absorb or mask Sensor Green’s fluorescence as these concentrations are much mo re hea vil y pig mented than lower c once ntratio ns. As expected, controls exhibited almost no 1O2 produc- tion. The initial HPRT gene mutation assay showed that ir- radiating ce lls with 0. 000 1% RB in Ha m’s F -12 was pos- sibly mutagenic, with roughly a doubling of the mutant frequency above background. However, results from the second experiment using lower RB concentrations sug- gested that RB was not mutagenic at 0.0001% or 0.00001% concentr ations . This is no t unrea sonabl e since the highest co ncentratio n is quite lo w (~1 uM). All ot her concentrations resulted in low or nonexistent colony formation due to RB’s cytotoxicity. Qualitatively, when comparing the HPRT groups to controls (colony forming plates), which were elevated beyond expected, there were no apparent differences in freq uency of H PRT mutations amongst experimental groups. In our opinion, the assay did not meet the quality standard s that we set, and several experiments could be repeated. Investigating the muta- genicity of photoactivated RB on skin cell lines should also be considered. 5. Conclusion With the current information at hand, it appears that higher concentrations of RB on viable cells are relatively cytotoxic. Singlet oxygen production increased from 0 to 0.001% RB, with detectable levels decreasing from 0.001% to 0.1% RB for unknown reasons. Further re- search might include a shorter time period between pho- toactivation and microplate fluorometry to rule out the auto-exhaustion theory, as well as different time points between exposure and ROS measurement. Given that photoactivated RB is currently being tested as a PTB agent on open wounds, additional research on the poten- tial mutagenicity of RB needs to be done. Should RB prove not to be mutagenic, future research might focus on determining the exact mechanisms and/or effects of PTB on wound healing a nd scar fo rmation. Perhap s PTB has potent ial to reduc e infectio n, additional post-operative pain, and poor cosmetic outcomes. Of note, photoacti- vated RB has recently shown promise as a fungicidal agent agai ns t Trichophyton rubrum [29]. Other ite ms that should be considered include the potential for an in- creased risk of future complications, such as localized pigment di s orders or even skin malig nancies. REFERENCES [1] Y. Kamegaya, W. Farinelli, A. V. Vila Echague, et al., “Evaluation of Photochemical Tissue Bonding for Clo- sure of Skin Incisions and Excisions,” Lasers in Surgery and Medicine, Vol. 37, No. 4, 2005, pp. 264-270. http://dx.doi.org/10.1002/lsm.20221 [2] S. Tsao, M. Yao, H. Tsao, et al., “Light-Activated Tissue Bonding for Excisional Wound Closure: A Split-Lesion Clinical Trial,” British Journal of Dermatology, Vol. 16 6 , No. 3, 2012, pp. 555-563. http://dx.doi.org/10.1111/j.1365-2133.2011.10710.x [3] M. Yao, A. Yaroslavsky, F. Henry, et al., “Photo-Toxi - city Is Not Associated with Photochemical Tissue Bond- ing of Skin,” Lasers in Surgery and Medicine, Vol. 42, No. 2, 2010, pp. 123-131. http://dx.doi.org/10.1002/lsm.20869 [4] D. Olivier, S. Douillard, I. Lhommeau, et al., “Photody- namic Treatment of Culture Medium Containing Serum Induces Long-Lasting Toxicity in Vitro,” Radiation Re- search, Vol. 172, No. 4, 2009, pp. 451-462. http://dx.doi.org/10.1667/RR1646.1 [5] S. Y. Kim and J.-W. Park, “Cellular D efense against Sing- let Oxygen-Induced Oxidative Damage by Cytosolic NADP+-dependent Isocitrate Dehydrogenase,” Free Ra- dical Research , Vol. 37, No. 3, 2003, pp. 309-316. http://dx.doi.org/10.1080/1071576021000050429 [6] B. P. Chan, I. E. Kochevar and R. W. Redmond, “En- hancement of Porcine Skin Graft Adherence Using a Light-Activated Process,” Journal of Surgical Research, Vol.108, No. 1, 2002, pp. 77-84. http://dx.doi.org/10.1006/jsre.2002.6516 [7] S. Tsao, M. Yao, F. P. Henry, et al., “Photochemical Tis- sue Bonding for Closure of Excision Wounds: A First - in-Human Clinical Trial Dermatology,” Journal of Inves- tigative Dermatology, Vol. 129, No. S37, 2009. [8] B. P. Chan and K.-F. So, “Photochemical Cross-Linking Improves the Physicochemical Properties of Collagen Scaffolds,” Journal of Biomedical Materials Research, Vol. 75A, N o. 3, 20 05, pp. 68 9-701. http://dx.doi.org/10.1002/jbm.a.30469 [9] L. Mulroy, J. Kim, I. Wu, et al., “Photochemical Kerato- desmos for Repair of Lamellar Corneal Incisions,” In- vestigative Ophthalmology & Visual Science, Vol. 41, 2000, pp . 3335-3340. [10] A. Camoirano, S. De Flora and T. A. Dahl, “Genotoxici- ty of Volatile and Secondary Reactive Oxygen Species Generated by Photosensitization,” Environmental and Molecular Mutagenesis, Vol. 21, No. 3, 1993, pp. 219- 228. http://dx.doi.org/10.1002/em.2850210304 OPEN ACCESS JC D SA
 Is the Photosuturing Agent, Rose Bengal, a Mutagen ? [11] B. P. Chan, O. C. M. Chan and K.-F. So, “Effects of Photochemical Crosslinking on the Microstructure of Collagen and a Feasibility Study on Controlled Protein Release,” Acta Biomaterlialia, Vol. 4, No. 6, 2008, pp. 1627-1636. http://dx.doi.org/10.1016/j.actbio.2008.06.007 [12] I. E. Kochevar and R. W. Redmond, “Photosensitized Production of Singlet Oxygen,” Methods in Enzymology, Vol. 319, 2000, pp. 20-28. http://dx.doi.org/10.1016/S0076-6879(00)19004-4 [13] A. Webster, D. Britton, A. Apap-Bologna, et a l., “A D ye - Photosensitized Reaction that Generates Stable Protein- Protein Crosslinks,” Analytical Biochemistry, Vol. 179, No. 1, 1989, pp. 154-157. http://dx.doi.org/10.1016/0003-2697(89)90216-9 [14] P. C. C. Lee and M. A. J. Rodgers, “Laser Flash Photoki- netic Studies of Rose Bengal Sensitized Photodynamic Interactions of Nucleotides and DNA,” Photochemistry and Photobiology, Vol. 45, No. 1, 1987, p p. 79-86. http://dx.doi.org/10.1111/j.1751-1097.1987.tb08407.x [15] L.-O. Klotz, K. Briviba and H. Sies, “Singlet Oxygen Mediates the Activation of JNK by UVA Radiation in Human Skin Fibroblasts,” FEBS Lett ers, V ol. 408, No. 3, 1997, pp . 289-291. http://dx.doi.org/10.1016/S0014-5793(97)00440-7 [16] M. Wiener, D. D amian and J. Thompson, “Syst e mi c Ph o - totoxicity Following Intralesional Rose Bengal for Sub- cutaneous Melanoma Metastases,” Dermatology, Vol. 216, No. 4, 2008, pp. 361-362. http://dx.doi.org/10.1159/000117707 [17] P. Bilski, B. Kukielczak and C. Chignell, “Photo-Pro- duction and Direct Sp ectral Detection of Singlet Molecu- lar Oxygen (1O2) in Keratinocytes Stained with Rose Bengal,” Photochemistry and Photobiology, Vol. 68, No. 5, 1998, pp. 675-678. [18] R. P. G. Feenstra and S. C. G. Tseng, “What Is Actually Stained by Rose Bengal?” Archives of Ophthalmology, Vol. 110, No. 7, 1992, pp. 984-993. http://dx.doi.org/10.1001/archopht.1992.01080190090035 [19] R. Costa de Oliveir, D. T. Ribeir, R. G. Nigro, et al., “Singlet Oxygen Induced Mutation Spectrum in Mamma- lian Cells,” Nucleic Acids Research, Vol. 20, No. 16, 1992, pp . 4319-4323. http://dx.doi.org/10.1093/nar/20.16.4319 [20] R. S. Gupta and B. Singh, “Mutagenic Responses of Five Independent Genetic Loci in CHO Cells to a Variety of Mutagens: Development and Characteristics of a Mutagen Screening System Based on Selection of Multiple Drug- Resistant Markers,” Mutation Research, Vol. 94, No. 2, 1982, pp . 449-466. http://dx.doi.org/10.1016/0027-5107(82)90307-4 [21] L. Hunter, S. Wang, J. Wickliffe, et al., “Photoactivated Rose Bengal, a Possible Mutagen in Chinese Hamster Ovary Cells,” Journal of Investigative Dermatology, Vol . 130, No. S138, 2010. [22] A. L. Tajirian and D. J. Goldberg, “A Review of Sutures and Other Skin Closure Materials,” Journal of Cosmetic and Laser Therapy, Vol. 12, No. 6, 2010, pp. 296-302. http://dx.doi.org/10.3109/14764172.2010.538413 [23] E. P. Tierney, R. L. Moy and D. J. Kouba, “Rapid Ab- sorbing Gut Suture versus 2-Octylethylcyanoacrylate Tis- sue Adhesi ve in the Epidermal Closure of Linear Rep airs,” Journal of Drugs in Dermatology, Vol. 8, No. 2, 2009, pp. 115-119. [24] A. D. Altman, V. M. Allen, S. A. McNeil, et al., “Pfa n- nenstiel Incision Closure: A Review of Current Skin Closure Techniques,” Journal of Obstetrics & Gynaecol- ogy Canada, Vol. 31, No. 6, 2009, pp. 514-520. [25] J. W. Eichenbaum, P. H. Pevsner, G. Pivawer, et al., “A Murine Photochemical Stroke Model with Histologic Cor- relates of Apoptotic and Nonapoptotic Mechanisms,” Journal of Pharmacological and Toxicological Methods, Vol. 47, N o. 2, 20 02 , pp. 67-71. http://dx.doi.org/10.1016/S1056-8719(02)00215-0 [26] S. Basu-Modak and R. Tyrrell, “Singlet Oxygen: A Pri- mary Effector in the Ultraviolet A/Near-Visible Light In- duction of the Human Heme Oxygenase Gene,” Cancer Research, Vol. 53, 1993, pp. 4505-4510. [27] V. Au and S. A. Madison, “Effects of Singlet Oxygen on the Extracellular Matrix Protein Collagen: Oxidation of the Collagen Crosslink Histi-Dinohydroxylsinonorleucine and Histidine,” Archives of Biochemistry and Biophysics, Vol. 384, No. 1, 20 00, pp. 133-142. http://dx.doi.org/10.1006/abbi.2000.2070 [28] E. Wachter, C. Dees, J. Harkins, et al., “Topical Rose Bengal: Pre-Clinical Evaluation of Pharma-Cokinetics and S afety,” Lasers in Surgery and Medicine, Vol. 32, No. 2, 2003, pp. 101-110. http://dx.doi.org/10.1002/lsm.10138 [29] L. Cronin, M. Moffitt, D. Mawad, et al., “An in Vitro Study of the Photodynamic Effect of Rose Bengal on Trichophyton rubrum,” Journal of Biophotonics, 2012, pp. 1-9. OPEN ACCESS JC D SA
|