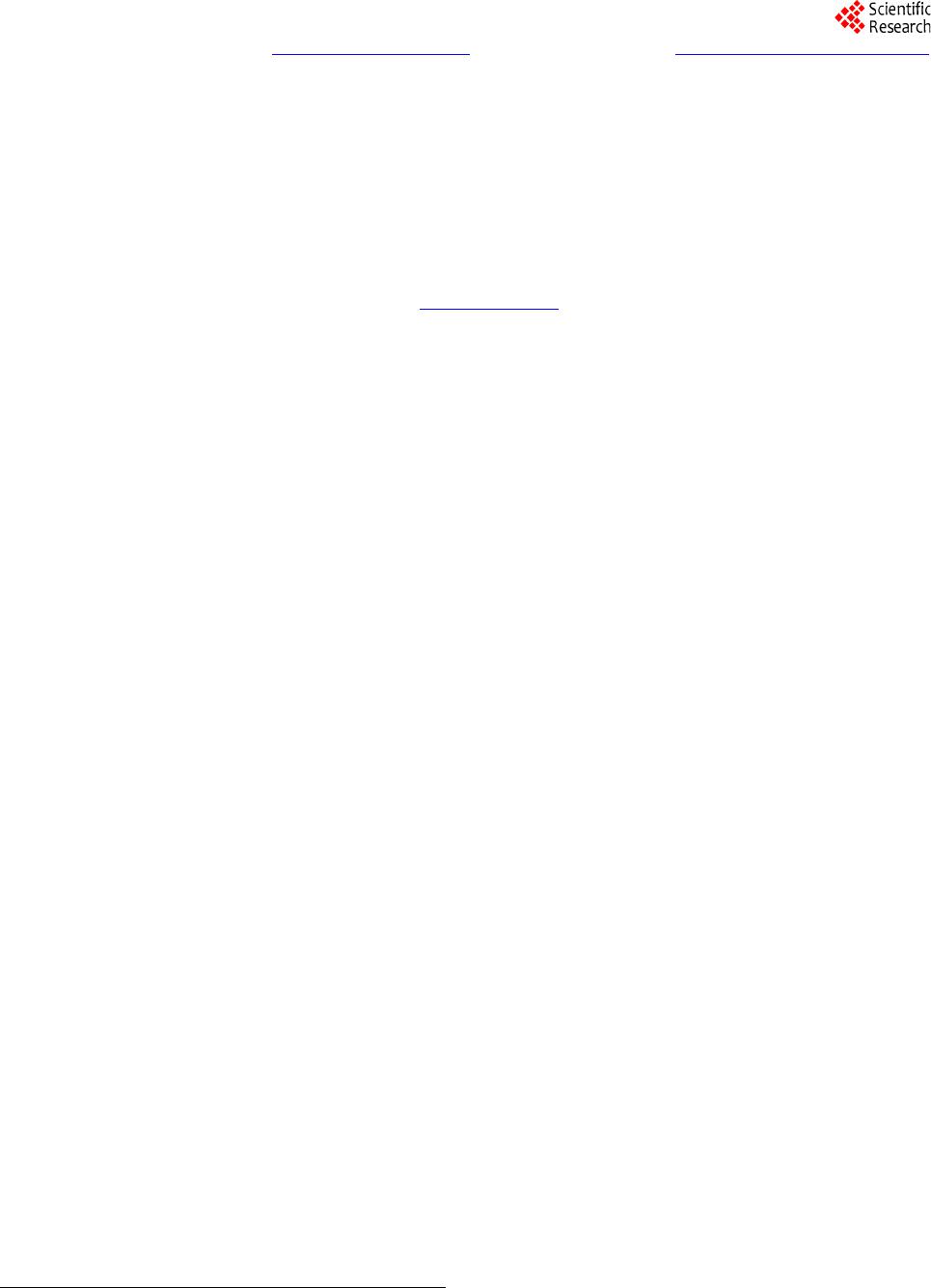 Open Journal of Forestry 2014. Vol.4, No.1, 28-33 Published Online January 2014 in SciRes (http://www.scirp.org/journal/ojf) http://dx.doi.org/10.4236/ojf.2014.41005 OPEN ACCESS Is Evidence-Based Conservation Applied in Urban Forestry? A Case Study from Toronto, Canada Adam R. Martin1,2, W. Eric Davies1, Danijela Puric-Mladenovic1,2, Sandy M. Smith1,2* 1Faculty of Forestry, University of Toronto, Earth Sciences Building, Toronto, Canada 2Information Management and Spatial Analysis, Southern Science and Information Section, Ministry of Natural Resources, Peterborough, Canada Email: *s.smith.a@utoronto.ca Received October 12th, 2013; revised November 19th, 2013; accepted December 6th, 2013 Copyright © 2014 Adam R. Martin et al. This is an open access article distributed u nder the Creative Commons Attribution License, which pe rmits unrestricted use, distrib ution, and reprodu ction in any medium, provided the original work is properly cited. In accordance of the Creative Commons Attribution License all Copyrights © 2014 are reserved for SCIRP and the owner of the in tellectual property Adam R. Martin et al. All Copyright © 2014 are guarded by law and by SCIRP as a guardian. Evidence-based conservation seeks to incorporate sound scientific information into environmental deci- sion making. The application of this concept in urban forest management has tremendous potential, but to date has been little applied, largely because existing scientific studies emphasize the importance of urban forests in large-scale ecological and anthropogenic processes, but in practice, scientific evidence is os- tensibly incorporated into North American urban forest management only when deciding the fate of indi- vidual trees. Even under these disjunctive conditions, the degree to which evidence influences tree-level decisions remains debatable. In analyzing preliminary data from a case study from Toronto, Canada, we sought to test if and how scientific evidence factored into the decision to remove or preserve 53 trees, lo- cated in close proximity to a provincially significant area of natural and scientific interest (ANSI). We found that by far the strongest tree-level correlate of the recommendation to remove or preserve trees was whether or not an individual tree was in conflict with proposed development. In comparison, species identity, tree condition, and suitability for conservation were statistically unrelated to the final recom- mendation. Our findings provide the basis to expand our analysis to multiple case studies across Canada, and internationally. Furthermore, when interpreted with available research and policy, our preliminary (and future) analysis highlights clear opportunities where scientific evidence can and should be readily incorporated into urban forestry management and policy. Keywords: ANSI; Arboriculture; Black Oak; Evidence-Based Conservation; Land Use Planning; Toronto; Tree Bylaw; Urban Forestry; Urban Forest Policy Introduction In managed ecosystems, conservation policy and practice have traditionally been based on expert knowledge or qualita- tive information, as opposed to systematic evaluation of empir- ical scientific evidence and/or data (Sutherland et al., 2004). Inspired by changing practices in medical sciences, over the past decade, conservation biologists have sought to reverse this trend by promoting the concept of “evidence-based conserva- tion” (Pullin & Knight, 2001). Generally, evidence-based con- servation is a framework that suggests that environmental man- agement decisions should build upon rigorous scientific infor- mation stemming from well-designed experiments or other ob- jective and quantitative data (Pullin & Knight, 2003; Sutherland et al., 2004). To date, evidence-based conservation has been more applied to management of “traditional” systems such as operational forests and agricultural complexes (e.g. Dicks et al., 2013), or certain taxonomic groups such as birds and pollinators (e.g. Williams et al., 2012). Reviewing the scientific evidence asso- ciated with management activities in these systems has pro- vided insights into how management may achieve or fail to reach certain environmental goals. Systematically assessing scientific evidence associated with management has also re- vealed important gaps in our ability to evaluate and ultimately modify management practices in relation to environmental goals. For example, in managed North American forests biodi- versity conservation is a well-recognized goal, but due to a lack of information and/or scientific evidence there is considerable uncertainty as to how well current forest management practices are meeting biodiversity targets (Boutin et al., 2009). With respect to urban forestry (sensu Konijnendijk et al., 2006), the concept of evidence-based conservation has a com- plex and multi-scale application. On one hand, some of the strongest evidence in support of conserving and increasing urban green spaces is at larger spatial scales, such as individual parks or greenspaces, or entire urban forests as defined by mu- nicipal boundaries. For instance, the potential for urban forests to mitigate pollution and sequester CO2 has been well docu- mented for a number of cities globally (e.g. Nowak, 2006; Pataki et al., 2006). Similarly, biodiversity and greenspace size/ *Corresponding author.
A. R. MARTIN ET AL. OPEN ACCESS proximity metrics have been identified as correlates of human psychological and physical well-being (e.g. Full er et al., 2007), while total tree canopy was found to correlate with socio-eco- nomic patterns within cities (Donovan & Butry, 2010) and hu- man mortality rates across states (Donovan et al., 2013). The effects of urban forests have traditionally been cumula- tively measured in order to provide solid scientific evidence of the ecological and socio-economic benefits at relatively large spatial scales. However, policy makers have generally neg- lected the idea that these positive cumulative effects of urban forests are highly dependent on the ecological value of individ- ual trees: the organisms that are most affected by existing land development and/or conservation policies, or lack thereof. One reason for this is that critical laws and policies pertaining to urban forest planning and conservation have been historical, and remain commonly, at the individual tree level (Kon- ijnendijk et al., 2006; Ordóñez & Duinker, 2013); this, despite the benefits of urban forests, and evidence in support of their conservation, are largely understood at the municipal level. An early example of this disparity is the “tree warden laws” passed between 1896-1901 in the northeastern United States: laws explicitly permitting municipalities to appoint tree wardens to oversee the planting, care, and removal of individual trees (Ricard, 2005). Similarly in Canada, typical urban forest management goals (e.g. species diversity, tree size distribution targets) are based on, and achieved through, individual tree-level maintenance and planting programs (e.g. Ordóñez & Duinker, 2013); wheth- er or not these goals are met is then in turn highly contingent on urban forest policies (Barker & Kenney, 2012; Kenney et al., 2011). One example is the City of Toronto’s municipal private tree bylaw (City of Toronto, 2013), which places strict limita- tions on the removal of any trees ≥ 30 cm diameter at 1.3 m above ground (“dbh”), and requires the replacement of any trees that are removed. By comparison, ecosystem-level target s such as wildlife habitat provisioning and climate change miti- gation are very poorly defined in Canadian urban forestry management plans (Ordóñez & Duinker, 2013), despite consi- derable scientific evidence demonstrating the role urban forests can play in these ecological processes (e.g. Chace & Walsh, 2006; Nowak, 2006). Urban forest by-laws and their specific details related to sin- gle trees have critical implications to decision making and evi- dence-based conservation in Canadian urban forests. Often, the final decision for or against tree removal is obtained through a legal process, where a consulting arborist’s opinion about the consequences of tree removal is taken as rigorous scientific evidence. Decision making should therefore be informed by bylaws and tree-level information including species identity, tree diameter at breast height (dbh), tree age, and tree condition (City of Toronto 2013). From a technical perspective, these lines of evidence should be straightforward to measure because they are objective, quantitative, and prone to little error and interpretation relative to ecosystem-level metrics. From an ecological perspective, these metrics should reliably inform an objective opinion on the consequences of tree removal, as a number of scientific studies point to the ecological importance of these relatively simple tree-level characteristics such as dbh and species identity (e.g. Lacey, 1986; Lindenmayer et al., 2013). Even tree condition, arguably the most subjective and error-prone of tree-level measurements, can be objectively as- sessed with standard, unbiased and repeatable inventory proto- cols, or other technologies (e.g. Moore, 1999). However, when competitive interests exist for valuable urban space, it can be debatable how single tree-level biological evidence is incorpo- rated into tree assessments, and ultimately, into the advisement for or against tree preservation in urban forests. In this prelimi- nary analysis we present one such example. Here, we present an analysis of the evidence used in a recent decision to remove or preserve 53 individual trees in close proximity to a provincially significant ecological area, in To- ronto, Canada, in advance of an ongoing high-density residen- tial development. Using publically available court documents, we sought to provide the first stage of a larger analysis that evaluates the extent to which scientific evidence is used when recommending the removal or preservation of urban trees. In doing so, we also sought to identify areas and opportunities where scientific evidence can and must be entered into urban forest conservation policies and practices. A Case Study from Toronto Study Site Our preliminary study was based at the site of an ongoing residential development located north of High Park, a 161-ha greenspace in central T oronto, Canada (43˚38'47''N, 7 9˚27'47''W; Figure 1). The park itself (High Park) maintains approximately 60% canopy cover and is bound by streets to the north (Bloor Street), east (Parkside Drive), and south (Queensway), and by both a street (Ellis Park Drive) and 14.2-ha pond (Grenadier Pond) to the west (Figure 1; Kidd et al., 2000). In addition to being a popular recreational site, High Park contains a rare remnant patch of oak savannah that prior to European settle- ment was once part of a savannah ecosystem that remains in less than 1% of its original distribution in southern Ontario. The oak savannah canopy is dominated by black oak (Quer- cus velutina), and maintains a high-diversity understorey com- prised of several grass and forb species, many of which are provincially rare (Kidd, et al., 2000). Since 1999, the savannah ecosystem in High Park has been actively restored and ma- naged through a combination of periodic controlled burns and removal of exotic species (Kidd et al., 2000). The City of To- ronto has designated High Park as an “Ecologically Sensitive Area” (ESA), and has incorporated the park into its natural heritage system. Due to the rarity of the oak savannah ecosys- tem at a provincial scale, large portions of High Park have also been designated by the government of Ontario as an “Area of Natural and Scientific Interest” (ANSI; Figure 1; Kidd et al., 2000). Our study was based at a 0.65-ha development site located approximately 25 m north of the northern boundary of High Park (43˚39'13''N, 79˚27'46''W; Figure 1). The site is the loca- tion of an ongoing high-rise development, which was pre- viously a high-density area occupied by 13 two-to-three-storey residences. The site is slated to be replaced by a single 14-storey mixed residential-retail building (Ages Consultants Limited, 2013). The primary ecological concern prior to com- mencing development was determining whether or not several of the large trees on the site were to be conserved, based on their ecological value and condition. Specifically, it was re- quired that an impact study be conducted that would make ex- plicit recommendations to preserve or remove 53 individual
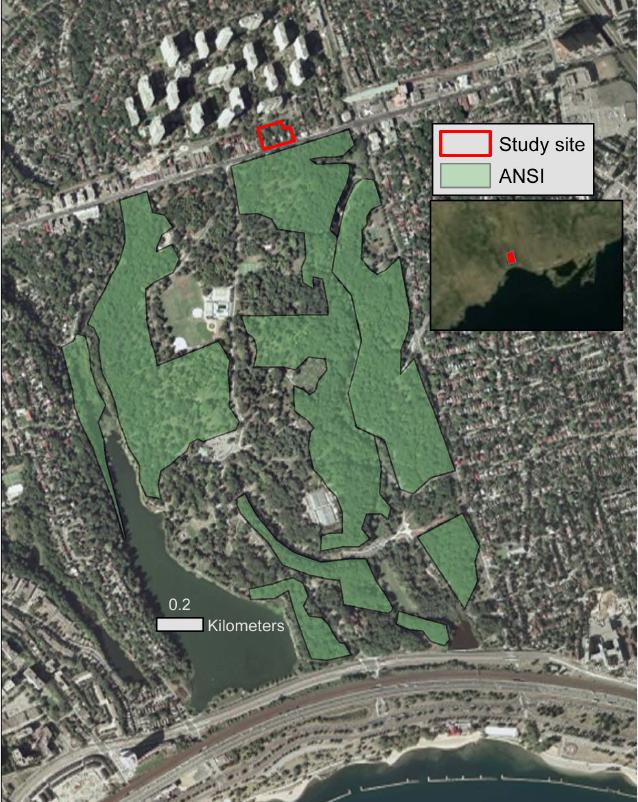 A. R. MARTIN ET AL. OPEN ACCESS Figure 1. Location of case study site in Toronto, Canada (red outline), relative to the area of natural and scientific interest (ANSI) in High Park (g reen shaded areas), as delinetated by Ontario’s Minis try of Natural Resources. trees, based on visual examinations performed following stan- dardized arboricultural criteria (Tree Care Industry Association, 2012). Tree-level evaluations were conducted in the summer of 2012 at which time data from an arboricultural assessment were presented within a report provided by a consulting arborist (Ages Consultants Limited, 2013, their Appendix 3). Within the report several qualitative and quantitative tree-level measure- ments were presented: 1) tree species identity; 2) tree size ex- pressed as diameter at breast height (dbh); 3) tree condition, assessed as “poor”, “fair”, “good”, “excellent”, or “hazardous” (following the Council of Tree and Landscape Appraisers, 2000); 4) Tree by-law policy requirements for tree protection determined by location and dbh (e.g. “Trees with diameters of 30 cm or more, situated on private property on the subject site.”); 5) suitability for conservation, categorized as “poor”, “moderate”, or “good”, which was in turn derived based on the criteria: a) tree health, b) structural integrity, c) species re- sponse, and d) tree age and longevity (following standards of Tree Care Industry Association, 2012); and 6) generalized qua- litative comments on tree status. In the final category, the only information presented for each individual tree assessed is whether or not it was “in conflict with proposed construction” versus “clear of proposed construction”. Based on the set of these six tree-level attributes, an explicit recommendation was made for each tree as “remove”, “preserve”, or “transplant”. Data Analysis Our analyses were designed to examine the relationship be- tween tree-level attributes measured and assessed, and the final tree-level recommendations (“remove”, “preserve”, or “trans- plant”) of the impact study (Ages Consultants Limited, 2013). For all analyses, we treated the final recommendation as a bi- nary response variable as being either “preserve” or “remove” (coded for analysis as 1 and 0 respectively); the single tree recommended for a “transplant” was classified as “preserve”.
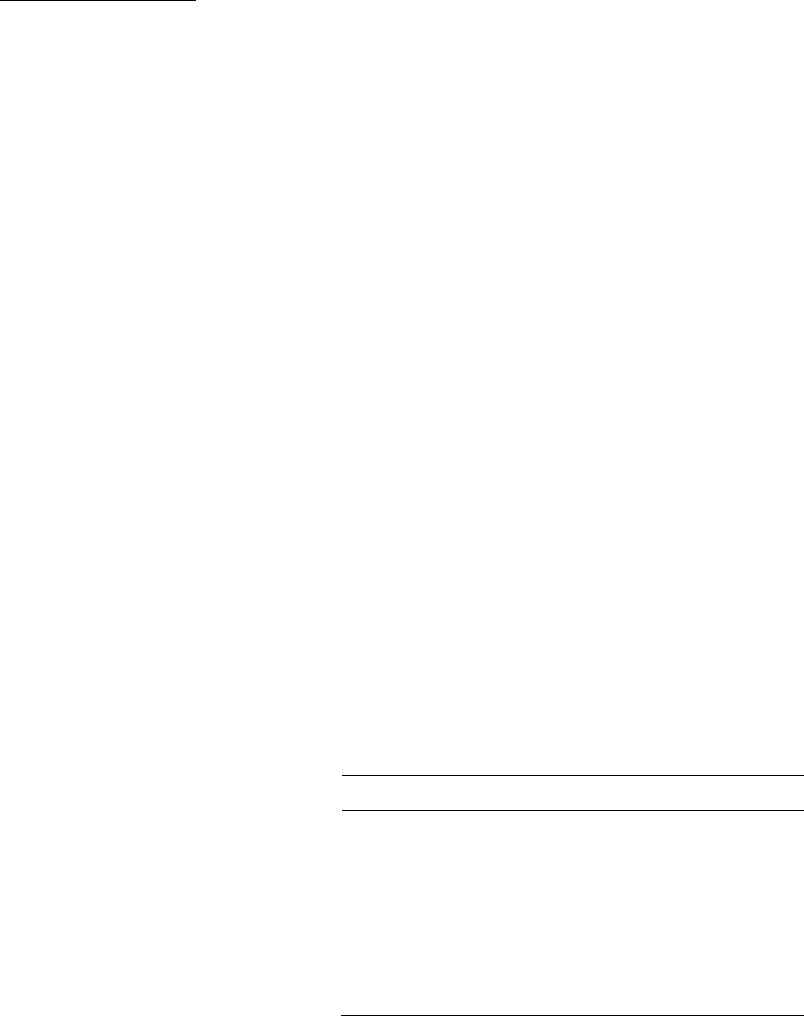 A. R. MARTIN ET AL. OPEN ACCESS We first used Chi-square tests to examine if the number of trees falling into each “tree condition” category were significantly related to “final recommendation”; the same analysis was per- formed to examine the relationship between “tree suitability for conservation” and “final recommendation”. For these analyses, due to several expected frequencies that were very low, all Chi-square test statistics and associated P-values were calcu- lated using Monte Carlo simulations with 2000 replicates used. We then used a logistic regression model to examine what tree-level variables best predicted the final recommendation. The logistic regression was performed as a generalized linear model with a binomial error distribution, and was of the form: ( ) 01234 5 Sp dbh Cd St Cf 1 1e p ββ βββ β −+ +++ + =+ (1) where p represents the probability that a tree will be recom- mended for preservation, ßo represents the intercept, ß1 represents the coefficient for species (“Sp”), ß2 represents the coefficient for tree dbh, ß3 represents the coefficient for tree condition (“Cd”), ß4 represents the coefficient for suitability for conservation (“St”), and ß5 represents the coefficient for whether or not the tree was in conflict with development (“Cf”). We then used a backward stepwise regression procedure on the full model (Equation (1)) to identify which set of predictor variables most parsimoniously explained the recommended outcome. Models were compared using Akaike’s Information Criteria (AIC) with the lowest AIC indicating the most parsi- monious prediction of recommended outcomes. All data ana- lyses were performed using R v. 2.10.1 (R Foundation for Sta- tistical Computing, Vienna, Austria). Results Of the 30 total trees deemed to be of “good” suitability for conservation, 5 were recommended for preservation while 25 were recommended for removal. Trees deemed to be of “mod- erate” suitability were largely recommended for removal (n = 7) as opposed to preservation (n = 1). All trees of “poor” conser- vation suitability (n = 15) were recommended for removal. The number of trees falling into each suitability-by-fina l-recom- mendation category did not differ significantly from a random expectation (χ2 = 2.78, P = 0.206), suggesting suitability for conservation was statistically unrelated to the final recommen- dation. Similarly, we found that tree condition and final recommen- dation were statistically unrelated, with the distribution of indi- viduals falling into each condition-by-recommendation group not differing significantly from a random expectation (χ2 = 5.33, P = 0.176). Of the trees considered to be of “fair” condition (n = 35), 85.7% were recommended for removal (n = 30) while 14.3% were recommended for preservation (n = 5). All trees in the “poor” (n = 14) and “hazardous” (n = 2) condition catego- ries were recommended for removal, while the two trees deemed to be of “good” quality were split evenly between re- moval and preservation recommendations. No trees evaluated were deemed to be of “excellent” quality. We found that the most parsimonious explanation of recom- mended outcomes was predicted by a combination of whether or not the tree was in conflict with the proposed development, and tree dbh (AIC = 9.6 vs. AIC = 46 in the full model, Table 1). Of these two variables, tree conflict was a much more im- portant variable: the AIC value of a model including only the conflict term (AIC = 13.72) was considerably lower than that from a model with only tree dbh as a predictor (AIC = 36.73; Table 1). This was supported by qualitative evaluation of the data. Of the total trees recommended for preservation (n = 6), only one was in conflict with development (though this tree was in fact recommended for transplantation). All other trees in the dataset that were in conflict with construction (n = 47) were recommended for removal. Recommendations for preservation or removal based on dbh were less systematic: of the six trees slated for preservation dbh ranged widely from 2 - 41 cm dbh, with a mean preserved tree dbh of 24 ± 17.7 (s.d.) cm dbh. Information on tree species identity, tree condition, and tree suitability for conservation were not retained as important pre- dictors of final recommendations (Table 1). Discussion In urban forestry, conservation and management decisions, or recommendations on the fate of individual trees, should be based on quantitative information such as: tree-level characte- ristics (i.e. species, age, and tree size); objective and repeatable evaluations of tree condition; and importance of urban trees at different spatial scales of urban forests (i.e. across land use types, municipalities, neighborhoods, etc.). This is ostensibly the purpose of mandating standard tree evaluations prior to development, and is ultimately critical if we are to incorporate urban forest conservation into land use planning. Given that urban forestry is an applied science (Konijnendijk et al., 2006) and interacts with other disciplines (e.g. land use planning), it is tempting to think that evidence-based urban forest conservation, management and planning are implicitly incorporated into the decision making process. The results from our preliminary analysis raise questions as to the validity of this underlying assumption. In our preliminary case, we found no empirical evidence that tree-level metrics or ecological considerations had any influ- ence on the final recommendations about the removal or con- servation of the 53 trees. Recommendations on tree removal or conservation, prior to development were almost wholly deter- mined by whether or not an individual tree was in conflict with the planned development. Tree level measurements were not Table 1. Aikaikes information criteria (AIC) values for six competing logistic regression models used to predict the final recommendation to remove or preserve 53 individual trees in Toronto , Canada. The functional f orm of the full logistic regression model is presented in Equation (1), where predictor variables include species (“Sp”), tree dbh, tree condition (“Cd”), suitability for conservation (“St”), and whether or not the tree was in conflict wit h dev elo p ment (“Cf ”). The most parsimonious model fit is highlighted in bold. Model parameters AIC Sp + dbh + Cd + St + Cf 46 dbh + Cd + St + Cf 16 dbh + Cd + Cf 12 dbh + Cf 9.6 Cf 9.72 dbh 32.73
 A. R. MARTIN ET AL. OPEN ACCESS taken into account, nor were 1) the fact that the site and trees are in close proximity (~25 m) to a provincially significant ANSI (Figure 1), and 2) the fact that these trees contribute to overall urban canopy of the area. Whether or not this finding is supported in other cases, both in Canada and elsewhere, will be the focus of our future research. To further motivate the importance of this type of research, it should also be noted that our analysis here was restricted to only those empirical and qualitative data available for trees in our analyzed impact assessment report (Ages Consultants Limited, 2013). However, a cursory review of other informa- tion (as well as associated policy guidelines such as the City of Toronto tree bylaw (2013)) raises additional questions as to whether any ecological evidence is being incorporated into the actual land development decision-making. For example, one of the six trees recommended for preservation in our study was a 22-cm dbh black ash tree (Fraxinus nigra), a tree that is highly likely to be killed by the invasive emerald ash borer (EAB) (Agrilus planipennis) within a very short timeframe (Kovacs et al., 2010). In fact, in North American cities where EAB is present, preemptive ash removal is a recognized strategy for managing this rapidly spreading invasive species (Vannatta et al., 2012). Similarly, seven of the total trees recommended for removal had the added notation of “irreversible decline with limited lifespan”. These observations were based on qualitative assessments of tree crown dieback made in summer in 2012; one of the hottest and driest years on record for Toronto (Environment Canada, 2013). Therefore, observations of di- eback may well reflect short-term leaf and crown responses to extreme weather, as opposed to “irreversible decline” (e.g. Filewod, 2011); in larger trees crown thinning could also reflect natural ontogenic changes in leaf area index (Nock et al., 2008). Additionally to our knowledge, there are no existing studies directly linking crown dieback to prospective tree lifespan; for example, one study analyzing > 7500 A. saccharum trees across Ontario found no evidence for a relationship between crown condition and short-term (1 - 2 years) mortality rates (Tomina- ga et al., 2008). To support evidence-based decision making, tree lifespan could potentially be predi cted t o within a ~15-year span using dendrochronological information such as relative growth rates and short-term growth trends (Bigler & Bugmann, 2004). A more extensive analysis of arborist reports such as the one analyzed here is needed to determine whether urban forest practitioners have adopted such scientifically-based methods; such criteria were certainly not incorporated into the arborist’s speculation on prospective tree lifespan in our preliminary study . Dendrochronological information could also be easily incor- porated into tree age estimates, which can strongly influence the decision making process. For example, in our case study all black oaks >100 years old would predate the original housing development, and hence represent a genetic remnant of the ad- jacent black oaks and a remnant of provincially significant oak savannah habitat (Figure 1). However, ages for the black oaks were only qualitatively estimated from visual tree assessments, and were ultimately never precisely quantified prior to removal (Ages Consultants Limited, 2013). In comparison, our prelimi- nary dendrochronological analysis, based on photographs of the tree cross-sections taken while they were being transported to a dumpsite, suggested that the trees were at minimum 120 years of age. In the absence of definitive age data, all trees on the study site were considered ornamental landscape trees with no natural heritage value, and could therefore be simply re- placed with new individuals (Ages Consultants Limited, 2013). This lack of definitive tree ages and exclusion of natural herit- age value ultimately led decision makers to overlook the larg- er-scale ecological and historical importance of the trees at our site. For example, removal of the “ornamental” black oaks was suggested to have no impact on the genetic viability of the nearby oak population (Ages Consultants Limited, 2013)—a statement that is not at all supported by scientific evidence giv- en a) the complete lack of dendrochronological data employed when dating trees (Ages Consulting Limited, 2013), and b) a poor scientific understanding of minimum viable populations needed to conserve genetic variability in black oaks, and trees more generally (Koskela et al., 2013). Qualitatively ageing trees and the resultant dissociation of trees at our study site from those at the nearby ANSI (Figure 1), also likely influ- enced an undervaluing of the ecological role the trees at our site play in terms of wildlife habitat provisioning (Chace & Walsh, 2006)—a point that deserves considerably more attention than is permitted in our preliminary analysis. Over the past decade, urban forestry has emerged as an im- portant field of study owing to the work of scientists demon- strating many environmental, social and economic benefits urban forests provide to built-up areas (Bowler et al., 2010; Chace & Walsh, 2006; Donovan & Butry, 2010; Nowak, 2006; Schipperijn et a l., 2013). Unfortunately, these lines of evi dence do not factor heavily into the existing legalities related to land development, land use planning, urban forest and tree preserva- tion: a disconnect that has resulted in the loss of countless trees, many of which are keystone large old trees (Lindenmayer et al., 2013), due to development within and of the edge of the cities. In light of the existing urban development decisions, single tree assessments, which are often heavily based on an arboricultural approach, provide information for tree maintenance, but are simply not enough for impact assessment. Our intent in this preliminary analysis was to gain insights into the potential to further study the degree to which scientific evidence is incorporated into urban forest conservation and land development policy and practice. Prior to completion of our comprehensive analysis, our hope is that the case study pre- sented in this preliminary analysis will serve as a cautionary tale that data collection and evaluation during impact studies need to be quantitative and repeatable. We also caution that decision makers need adequate tree-level and ecological data to rigorously evaluate impact study reports. Therefore, in moving towards an evidence-based approach, it is necessary to ensure that sound scientific data and information are incorporated into urban forest management and land use planning. Such a rigor- ous approach to urban forest management and conservation represents a cross-disciplinary and long-term transformation, but examples from the field of conservation biology are instruc- tive in showing that it is possible, timely, and critically impor- tant. REFERENCES Ages Consulting Limited (2013). Natural heritage impact study. High Park: Daniels Corporation. Barker, E. J., & Kenney, W. A. (2012). Urban forest management in small Ontario municipalities. The Forestry Chronicle, 88, 118-123. Bigler, C., & Bugmann, H. (2004). Predicting the time of tree death using dendrochronological data. Ecological Applications, 14, 902- 914. http://dx.doi.org/10.1890/03-5011 Boutin, S., Haughland, D. L., Schieck, J., Herbers, J., & Bayne, E.
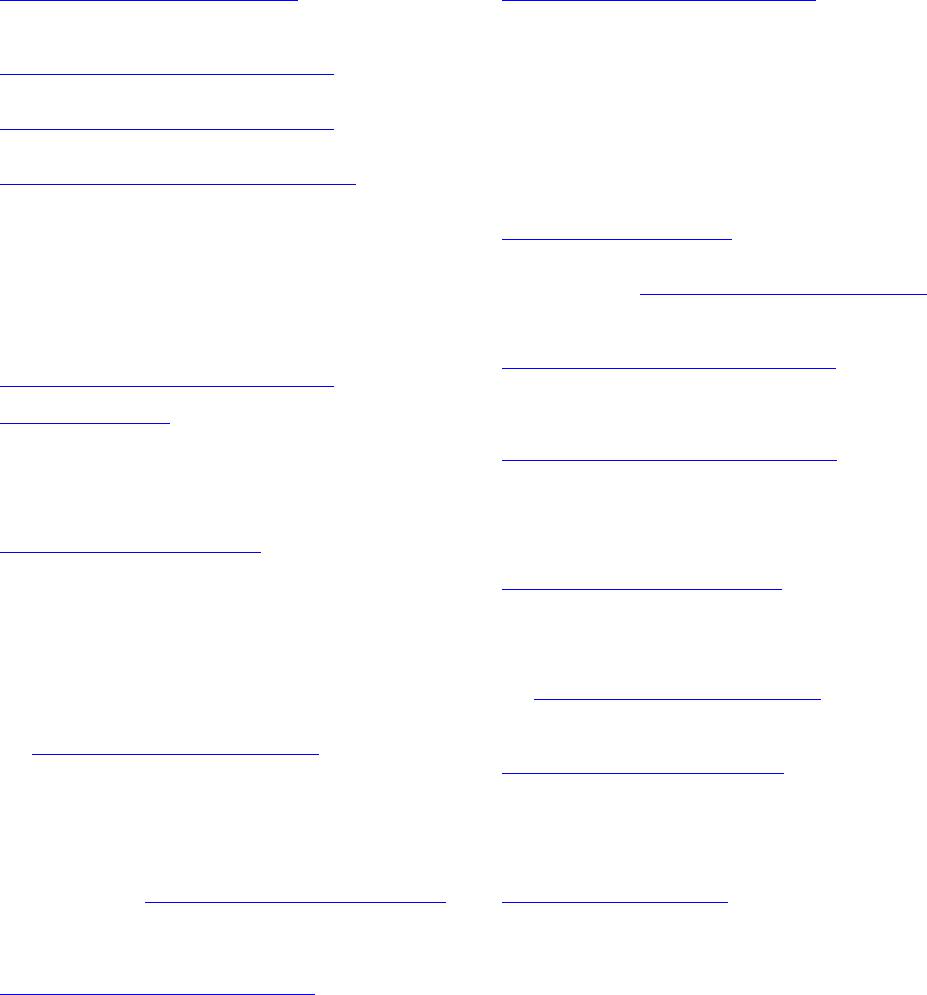 A. R. MARTIN ET AL. OPEN ACCESS (2009). A new approach to forest biodiversity monitoring in Canada. Forest Ecol ogy and Management, 258, S168-S175. http://dx.doi.org/10.1016/j.foreco.2009.08.024 Bowler, D. E., Buyung-Ali, L., Knight, T. M., & Pullin, A. S. (2010). Urban greening to co ol towns and cities: A systematic review of the empirical evidence. Landscape and Urban Planning, 97, 147-155. http://dx.doi.org/10.1016/j.landurbplan.2010.05.006 Chace, J. F., & Walsh, J. J . (2006). Urb an effects on native avifau na: A review. Landscape and Urban Pl anning, 74, 46-69. http://dx.doi.org/10.1016/j.landurbplan.2004.08.007 City of Toronto (2013). Toronto private tree by-law (Article III of Chapter 813). http://www.toronto.ca/legdocs/municode/1184_813.pdf Council of Tree and Landscape Appraisers (2000). Guide for plant appraisal (9th ed.). Champaign, IL: Council of Tree and Landscape Appraisers. Dicks, L. V., Ashpole, J. E., Dä nhardt, J., James, K ., Jö nsson, A., Ran- dall, N., Showler, D. A., Smith, R. K., Turpie, S., Williams, D., & Sutherland, W. J. (2013). Farmland conservation: Evidence for the effects of interventions in northern Europe (Volume 3). Exeter: Pe- lagic Publishing. Donovan, G. H., & Butry, D. T. (201 0). Trees in th e city: Valuing stree t trees in Portland, Oregon. Landscape and Urban Planning, 94, 77-83. http://dx.doi.org/10.1016/j.landurbplan.2009.07.019 Environment Canada (2013). Climate change science and research. http://www.ec.gc.ca/sc-cs/ Filewod, B. (2 011). Effects of an early season heat wave on ecophysio- logical parameters related to productivity in Sugar Maple (Acer saccharum Marsh.). Toronto: University of Toronto. Fuller, R. A., Irvine, K. N., Devine-Wright, P., Warren, P. H., & Gaston, K. J. (2007). Psychological b enefits of greenspace increase with bio- diversity. Biology Letters, 3, 390-394. http://dx.doi.org/10.1098/rsbl.2007.0149 Kenney, W. A., va n Wassenaer, P. J. E., & Satel , A. L. (201 1). Criteri a and indicators for strategic urban forest planning and management. Arboriculture & Urban Forestry, 37, 108-117. Kidd, J., LURA Consulting, Christensen, T., & McEwen, B. (2000). High Park: Restoring a jewel of Toronto’s park system. Restoration strategies for High Park. Toronto: City of Toronto, Department of Forestry, Parks and Recreation. Konijnendijk, C. C., Ricard, R. M., Kenney, A., & Randrup, T. B. (2006). Defining urban forestry—A comparative perspective o f Nor- th America and Europe. Urban Forestry & Urban Greening, 4, 93- 103. http://dx.doi.org/10.1016/j.ufug.2005.11.003 Koskela, J., Lefèvre, F ., Schueler, S., Kraigher, H. , Olrik , D. C. , Hubert , J., Longauer, R., Bozzano, M., Yrjänä, L., Alizoti, P., Rotach, P., Vietto, L., Bordács, S., Myking, T., Eysteinsson, T., Souvannavong, O., Fady, B., De Cuyper, B., Heinze, B., Von Wühlisch, G., Du- cousso, A., & Ditlevsen, B. (201 3). Translatin g co n servatio n gen etics into management: Pan-European minimum requirements for dynamic conservation units of forest tree genetic diversity. Biological Con- servation, 157, 39-49. http://dx.doi.org/10.1016/j.biocon.2012.07.023 Kovacs, K. F., Haight, R. G., McCullough, D. G., Mercader, R. J., Siegert, N. W., & Liebho ld, A. M. (2010). Cost of potential emerald ash borer damage in US communities, 2009-2019. Ecological Eco- nomics, 69, 569-578. http://dx.doi.org/10.1016/j.ecolecon.2009.09.004 Lacey, E. P. (1986). Onset of reproduction in plants—Size-versus age- dependency. Trends in Ecology & Evolution, 1, 72-75. http://dx.doi.org/10.1016/0169-5347(86)90021-2 Lindenmayer, D. B., Laurance, W. F., Franklin, J. F., Likens, G. E., Banks, S. C., Blanchard, W., Gibbons, P., Ikin, K., Blair, D., McBurney, L., Manning, A. D., & Stein, J. A. R. (2013). New poli- cies for old trees: averting a global crisis in a keystone ecological structure. Conservation Letters, Early Online. Moore, W. (1999). The combined use of the resistograph and the shi- gometer for the accurate mapping and diagnosis of the internal con- dition of woody support organs of trees. Arboricultural Journal: The International J ournal of Urban Forestry, 23, 237-287. Nock, C. A., Caspersen, J. P., & Thomas, S. C. (2008). Large ontoge- netic declines in intra-crown leaf area index in two temperate deci- duous tree species. Ecology, 89, 744-753. http://dx.doi.org/10.1890/07-0531.1 Nowak, D. J. (2006). Institutio nalizing urban forestry as a “biotechnol- ogy” to improve environmental quality. Urban Forestry & Urban Greening, 5, 93-100. http://dx.doi.org/10.1016/j.ufug.2006.04.002 Ordóñez, C., & Duinker, P. N. (2013). An analysis of urban forest management plans in Canada: Implications for urban forest man- agement. Landscape and Urban Planning, 116, 36-47. http://dx.doi.org/10.1016/j.landurbplan.2013.04.007 Pataki, D. E., Alig, R. J ., Fung, A. S. , Golubiewski, N. E., Kennedy, C. A., McPherson, E. G., No wak, D. J., Pouyat, R. V., & Lankao, P. R. (2006). Urban ecosystems and the North American carbon cycle. Global Change B iology, 12, 2092-2102. http://dx.doi.org/10.1111/j.1365-2486.2006.01242.x Pullin, A. S., & Knight, T. M. (2001). Effectiveness in conservation practice: Pointers fro m medicine and public health . Conservation Bi- ology, 15, 50-54. Pullin, A. S., & Knight, T. M. (2003). Support for decision making in conservation practice: An evidence-based approach. Journal for Na- ture Conservati on, 11, 83-90. http://dx.doi.org/10.1078/1617-1381-00040 Ricard, R. M. (2005). Shade trees and tree wardens: Revising the his- tory of urban, forestry. Journal of Forestry, 103, 230-233. Schipperijn, J., Bentsen, P., Troelsen, J., Tof tager, M., & Stigsd o tter, U. K. (2013). Associations between physical activity and characteristics of urban green space. Urban Forestry & Urban Greening, 12, 109- 116. http://dx.doi.org/10.1016/j.ufug.2012.12.002 Sutherland , W. J., P ulli n, A. S., Do l man, P. M., & Kni gh t, T. M. (20 04 ). The need for evidence-based conservation. Trends in Ecology & Evolution, 19, 305-308. http://dx.doi.org/10.1016/j.tree.2004.03.018 Tree Care Industry Association (2012). Trees, shrubs, and other woody plant maintenance—Standard practice. Washington, DC: American National Standards Institute, Inc. Vannatta, A. R., H auer, R. H., & Schuettpelz, N. M. (2012). Econo mic analysis of Emerald Ash Borer (Coleoptera: Buprestidae) manage- ment options. Journal of Economic Ent omology, 105, 196-206. http://dx.doi.org/10.1603/EC11130 Williams, D. R., P ople, R. G., Showler, D. A., Dicks, L . V., Child, M. F., Zu Ermgassen, E. K. H. J. , & Sutherlan d, W. J. (201 2). Bird con- servation: Global evidence for the ef fects of interventions (Volume 2). Exeter: Pelagic Publishing.
|