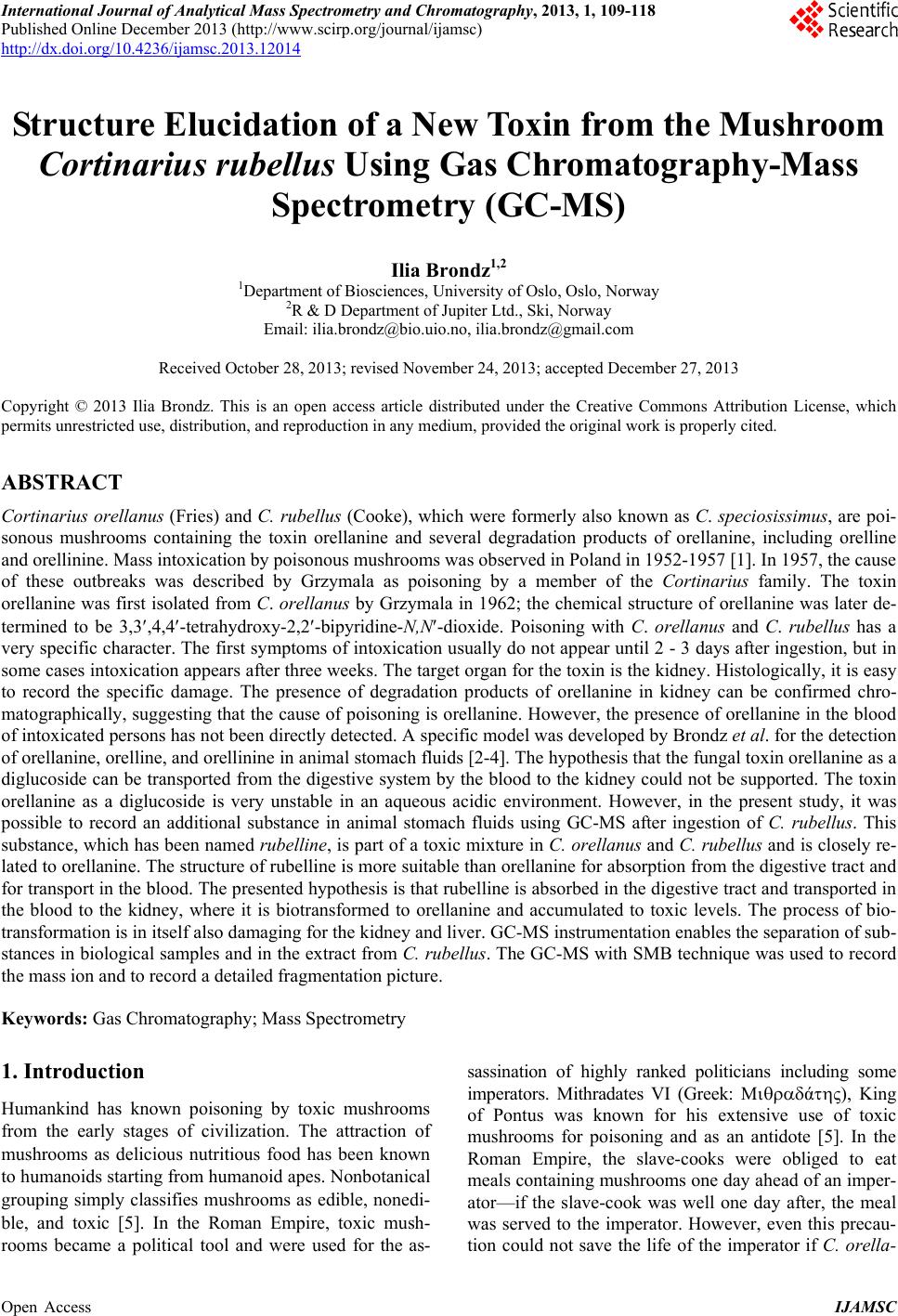 International Journal of Analytical Mass Spectrometry and Chromatography, 2013, 1, 109-118 Published Online December 2013 (http://www.scirp.org/journal/ijamsc) http://dx.doi.org/10.4236/ijamsc.2013.12014 Open Access IJAMSC Structure Elucidation of a New Toxin from the Mushroom Cortinarius rubellus Using Gas Chromatography-Mass Spectrometry (GC-MS) Ilia Brondz1,2 1Department of Biosciences, University of Oslo, Oslo, Norway 2R & D Department of Jupiter Ltd., Ski, Norway Email: ilia.brondz@bio.uio.no, ilia.brondz@gmail.com Received October 28, 2013; revised November 24, 2013; accepted December 27, 2013 Copyright © 2013 Ilia Brondz. This is an open access article distributed under the Creative Commons Attribution License, which permits unrestricted use, distribution, and reproduction in any medium, provided the original work is properly cited. ABSTRACT Cortinarius orellanus (Fries) and C. rubellus (Cooke), which were formerly also known as C. speciosissimus, are poi- sonous mushrooms containing the toxin orellanine and several degradation products of orellanine, including orelline and orellinine. Mass intoxication by poisonous mushrooms was observed in Poland in 1952-1957 [1]. In 1957, the cause of these outbreaks was described by Grzymala as poisoning by a member of the Cortinarius family. The toxin orellanine was first isolated from C. orellanus by Grzymala in 1962; the chemical structure of orellanine was later de- termined to be 3,3,4,4-tetrahydroxy -2,2-bipyridine-N,N-dioxide. Poisoning with C. orellanus and C. rubellus has a very specific character. The first symptoms of intoxication usually do not appear until 2 - 3 days after ingestion, but in some cases intoxication appears after three weeks. The target organ for the toxin is the kidney. Histologically, it is easy to record the specific damage. The presence of degradation products of orellanine in kidney can be confirmed chro- matographically, suggesting that the cause of poisoning is orellanine. However, the presence of orellanine in the blood of intoxicated persons has not been directly detected. A specific model was developed by Brondz et al. for the detection of orellanine, orelline, and orellinine in animal stomach fluids [2-4]. The hypothesis that the fungal toxin orellanine as a diglucoside can be transported from the digestive system by the blood to the kidney could not be supported. The toxin orellanine as a diglucoside is very unstable in an aqueous acidic environment. However, in the present study, it was possible to record an additional substance in animal stomach fluids using GC-MS after ingestion of C. rubellus. This substance, which has been named rubelline, is part of a toxic mixture in C. orellanus and C. rubellus and is closely re- lated to orellanine. The structure of rubelline is more suitable than orellanine for absorption from the digestive tract and for transport in the blood. The presented hypothesis is that rubelline is absorbed in the digestive tract and transported in the blood to the kidney, where it is biotransformed to orellanine and accumulated to toxic levels. The process of bio- transformation is in itself also damaging for the kidney and liver. GC-MS instrumentation enables the separation of sub- stances in biological samples and in the extract from C. rubellus. The GC-MS with SMB technique was used to record the mass ion and to record a detailed fragmentation picture. Keywords: Gas Chromatography; Mass Spectrometry 1. Introduction Humankind has known poisoning by toxic mushrooms from the early stages of civilization. The attraction of mushrooms as delicious nutritious food has been known to humanoids starting from humanoid apes. Nonbotanical grouping simply classifies mushrooms as edible, nonedi- ble, and toxic [5]. In the Roman Empire, toxic mush- rooms became a political tool and were used for the as- sassination of highly ranked politicians including some imperators. Mithradates VI (Greek: άς), King of Pontus was known for his extensive use of toxic mushrooms for poisoning and as an antidote [5]. In the Roman Empire, the slave-cooks were obliged to eat meals containing mushrooms one day ahead of an imper- ator—if the slave-cook was well one day after, the meal was served to the imperator. However, even this precau- tion could not save the life of the imperator if C. orella-
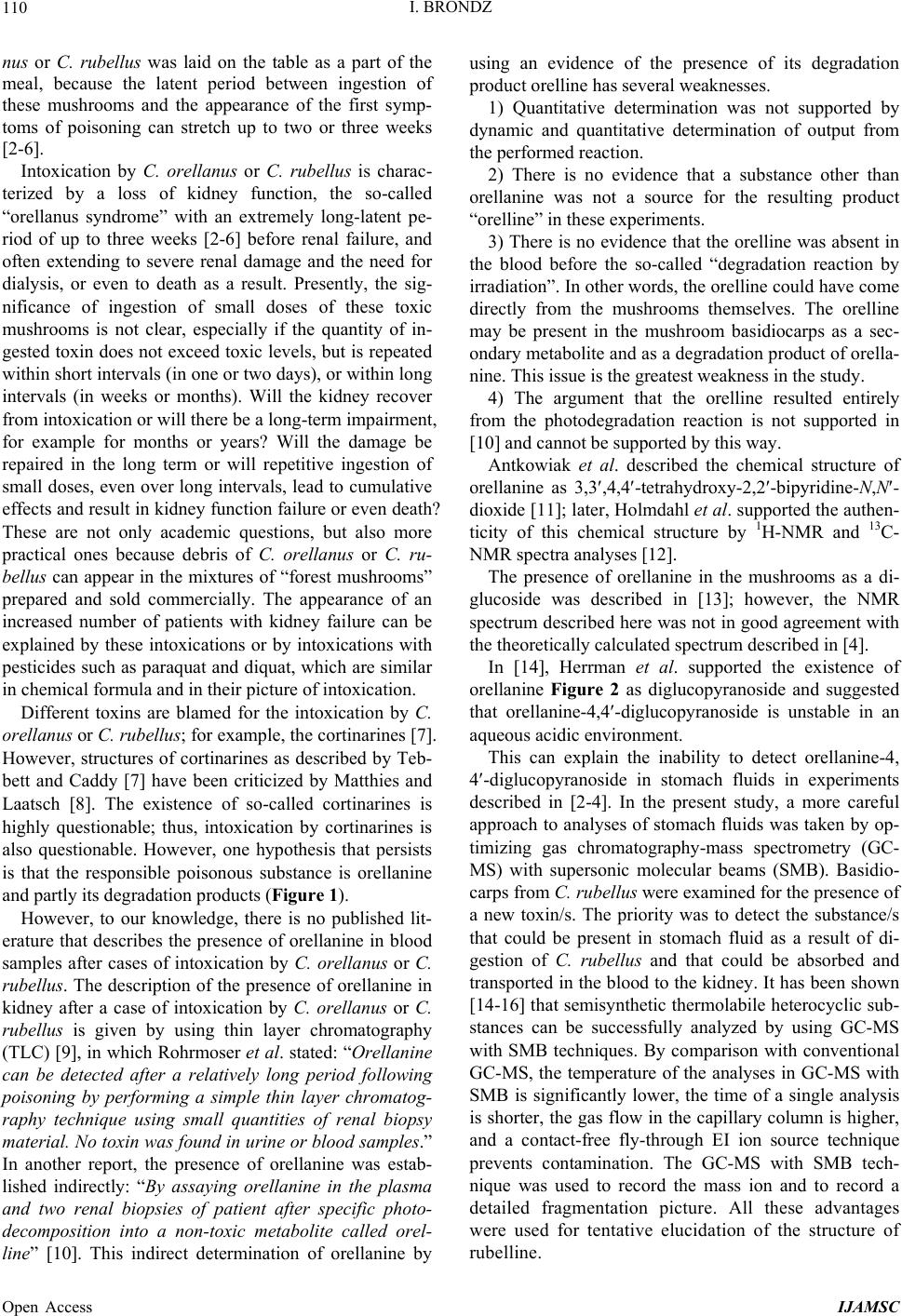 I. BRONDZ 110 nus or C. rubellus was laid on the table as a part of the meal, because the latent period between ingestion of these mushrooms and the appearance of the first symp- toms of poisoning can stretch up to two or three weeks [2-6]. Intoxication by C. orellanus or C. rubellus is charac- terized by a loss of kidney function, the so-called “orellanus syndrome” with an extremely long-latent pe- riod of up to three weeks [2-6] before renal failure, and often extending to severe renal damage and the need for dialysis, or even to death as a result. Presently, the sig- nificance of ingestion of small doses of these toxic mushrooms is not clear, especially if the quantity of in- gested toxin does not exceed toxic levels, but is repeated within short intervals (in one or two days), or within long intervals (in weeks or months). Will the kidney recover from intoxication or will there be a long-term impairment, for example for months or years? Will the damage be repaired in the long term or will repetitive ingestion of small doses, even over long intervals, lead to cumulative effects and result in kidney function failure or even death? These are not only academic questions, but also more practical ones because debris of C. orellanus or C. ru- bellus can appear in the mixtures of “forest mushrooms” prepared and sold commercially. The appearance of an increased number of patients with kidney failure can be explained by these intoxications or by intoxications with pesticides such as paraquat and diquat, which are similar in chemical formula and in their picture of intoxication. Different toxins are blamed for the intoxication by C. orellanus or C. rubellus; for example, the cortinarines [7]. However, structures of cortinarines as described by Teb- bett and Caddy [7] have been criticized by Matthies and Laatsch [8]. The existence of so-called cortinarines is highly questionable; thus, intoxication by cortinarines is also questionable. However, one hypothesis that persists is that the responsible poisonous substance is orellanine and partly its degradation products (Figure 1). However, to our knowledge, there is no published lit- erature that describes the presence of orellanine in blood samples after cases of intoxication by C. orellanus or C. rubellus. The description of the presence of orellanine in kidney after a case of intoxication by C. orellanus or C. rubellus is given by using thin layer chromatography (TLC) [9], in which Rohrmoser et al. stated: “Orellanine can be detected after a relatively long period following poisoning by performing a simple thin layer chromatog- raphy technique using small quantities of renal biopsy material. No toxin was found in urine or blood samples.” In another report, the presence of orellanine was estab- lished indirectly: “By assaying orellanine in the plasma and two renal biopsies of patient after specific photo- decomposition into a non-toxic metabolite called orel- line” [10]. This indirect determination of orellanine by using an evidence of the presence of its degradation product orelline has several weaknesses. 1) Quantitative determination was not supported by dynamic and quantitative determination of output from the performed reaction. 2) There is no evidence that a substance other than orellanine was not a source for the resulting product “orelline” in these experiments. 3) There is no evidence that the orelline was absent in the blood before the so-called “degradation reaction by irradiation”. In other words, the orelline could have come directly from the mushrooms themselves. The orelline may be present in the mushroom basidiocarps as a sec- ondary metabolite and as a degradation product of orella- nine. This issue is the greatest weakness in the study. 4) The argument that the orelline resulted entirely from the photodegradation reaction is not supported in [10] and cannot be supported by this way. Antkowiak et al. described the chemical structure of orellanine as 3,3,4,4-tetrahydroxy-2,2-bipyr idin e- N,N- dioxide [11]; later, Holmdahl et al. supported the authen- ticity of this chemical structure by 1H-NMR and 13C- NMR spectra analyses [12]. The presence of orellanine in the mushrooms as a di- glucoside was described in [13]; however, the NMR spectrum described here was not in good agreement with the theoretically calculated spectrum described in [4]. In [14], Herrman et al. supported the existence of orellanine Figure 2 as diglucopyranoside and suggested that orellanine-4,4-diglucopyranoside is unstable in an aqueous acidic environment. This can explain the inability to detect orellanine-4, 4-diglucopyranoside in stomach fluids in experiments described in [2-4]. In the present study, a more careful approach to analyses of stomach fluids was taken by op- timizing gas chromatography-mass spectrometry (GC- MS) with supersonic molecular beams (SMB). Basidio- carps from C. rubellus were examined for the presence of a new toxin/s. The priority was to detect the substance/s that could be present in stomach fluid as a result of di- gestion of C. rubellus and that could be absorbed and transported in the blood to the kidney. It has been shown [14-16] that semisynthetic thermolabile heterocyclic sub- stances can be successfully analyzed by using GC-MS with SMB techniques. By comparison with conventional GC-MS, the temperature of the analyses in GC-MS with SMB is significantly lower, the time of a single analysis is shorter, the gas flow in the capillary column is higher, and a contact-free fly-through EI ion source technique prevents contamination. The GC-MS with SMB tech- nique was used to record the mass ion and to record a detailed fragmentation picture. All these advantages were used for tentative elucidation of the structure of rubelline. Open Access IJAMSC
 I. BRONDZ Open Access IJAMSC 111 Figure 1. The poisonous substances in Cortinarius orellanus and C. rubellus are orellanine structures 1 and 4, and the degra- dation products orellinine structure 2 and orelline structure 3. Figure 2. The structure of orellanine diglucopyranoside as presented in [13]. 2. Materials and Methods 2.1. Biological Material, Standards, and Extracts for Biological Testing Mushrooms C. rubellus were kindly provided by Jupiter Ltd., Norway and freeze-dried to a constant weight as described in [4]. The procedure for extraction and isola- tion of their toxins was conducted as described in [17]. The orellanine standards were donated by Jupiter Ltd., Norway. Cells of Chlamydomonas reinhardtii were pre- pared as previously described in detail [18,19]. 2.2. Biological Testing of Toxicity The test model used in the experiment was as previously described [18,19]. 2.3. Instrumentation and Conditions GC-MS instrumentation enabling the separation of sub- stances in biological samples and in the extract from C. rubellus is described in detail in [16]. The extracted sub- stances were dissolved in methanol p.a. quality (Merck, Darmstadt, Germany). The capillary column used was an Rxi-5Sil MS low polarity phase; Crossbond 1,4-bis (di- methylsiloxy) phenylene dimethyl polysiloxane catalog no. 13620, 15 m, ID 0.25 mm, film thickness 0.25 m (Restek, Bellefonte, PA, USA). The column was reduced to about 4.5 m in laboratory. The helium column flow rate was 8 mL/min. Samples (1 L) at an approximate concentration of 20 ppm of dry mass were injected with a split ratio of 10:1 using a Varian 1079 injector operated at 150˚C. The GC oven was programmed from 130˚C to 330˚C at 20˚C/min. Ion source degradation was prevented by virtue of a contact-free fly-through EI ion source [16], and degrada- tion of substances in the sample was further avoided be- cause of the use of a short column and a high gas flow rate. The MS instrument was operated in an electron impact (EI) mode at 70 eV. The mass spectra between m/z 50 and m/z 700 were obtained at a scan speed of 200 m/z unit scan/s with a mass resolution corresponding to 1 unit at half peak height. Separation and detection of sub- stances in the stomach of Mus rattus L. are shown in the
 I. BRONDZ 112 reconstructed ion chromatogram (RIC) in Figure 3 and the substances isolated directly from C. rubellus in Fig- ure 4. The mass spectra of substances shown in Figure 3 are shown in Figures 5-8 . 3. Results and Discussion 3.1. Biological Testing of Toxicity The toxicological test is described in [18,19]. The thres- hold concentration of dry substance rubelline from C. rubellus used for inhibition of growth of Chlamydomo- nas reinhardtii cells was 10 ppm. Only qualitative results were recorded. Cell movement was abolished after ex- posure to the extract. This abolition of cell movement was the result of flagella blockage [20]; the authors of [20] noted: “The evidence is strongest for a role in triggering the shedding of flagella by the unicellular alga Chlamy- domonas reinhardtii under acid stress. Rapid kinetic Figure 3. An RIC of the analyzed stomach fluid is shown: peak A is rubelline, peak B is orelline, peak C is orellinine, and peak D is orellanine. Figure 4. An RIC of the rubelline. Open Access IJAMSC
 I. BRONDZ 113 Figure 5. Mass spectrum of rubelline. Figure 6. Mass spectrum of orellanine. Open Access IJAMSC
 I. BRONDZ 114 Figure 7. Mass spectrum of orelline. Figure 8. Mass spectrum of orellinine. Open Access IJAMSC
 I. BRONDZ Open Access IJAMSC 115 analysis indicates that phosphoinositide hydrolysis oc- curs within half a second and could trigger the rapid loss of flagella.” Most of the cells lost their chlorophyll and the cell lysis began as a result of exposure to the toxin. Figure 1 presents orellanine and its degradation prod- ucts. Degradation of orellanine occurs in the bodies of intoxicated persons and other organisms after ingestion of the mushrooms, in the basidiocarps of the mushrooms themselves, and during extraction. The diglucoside of orellanine is an unstable substance (Figure 2) and degrades to the monoglucoside and orellanine itself [13,14]. The diglucoside of orellanine is particularly unstable in aqueous solutions at acidic pH [14] and this is probably the reason that it was not de- tected in experiments described in [2-4]. However, if the molecular structure exists, there is little chance that it will be absorbed and transferred into the blood stream because of its extreme instability in the aqueous acidic conditions [14] in the stomach. 3.2. Analysis of Stomach Fluid from Mus rattus L. The time of digestion of a meal containing C. rubellus in the stomach of the mouse Mus rattus L. was reduced to 1 h. The injector temperature in GC was set at 150˚C (low by comparison with previous analyses) to minimize the degradation at the injector. The VF-5HT column, I.D. 0.25 mm, film thickness 0.1 m, and 4 m length (Varian, Middelburg, The Netherlands) was changed to an Rxi- 5Sil MS low polarity phase; Crossbond 1,4-bis (dime- thylsiloxy) phenylene dimethyl polysiloxane, catalog no. 13620.15 m, I.D. 0.25 mm, film thickness 0.25 m (Restek) column, which was reduced in length to about 4.5 m in the laboratory. A high flow rate in the capillary was used to reduce the time of analysis and avoid degra- dation of the analytes. An RIC of stomach fluid from Mus rattus L. is shown in Figure 3. An RIC of authentic rubelline donated by Jupiter Ltd. is shown in Figure 4. The retention time for the peak A in the chromatogram in Figure 3 is the same as the retention time of standard in Figure 4. The mass spectrum shown in Figure 5 is the mass spectrum of peak A shown in Figure 3 and it is similar to the mass spectrum derived from the standard shown in Figure 4. The m/z of rubelline is 4 units greater than the m/z of orellanine eluted under peak D; the mass spectrum of the substance eluted under peak D is shown in Figure 6. The mass spectrum shown in Figure 6 is characteristic of orellanine. The substance eluted under peak B yielded the mass spectrum shown in Figure 7. The substance eluted under peak C has the mass spec- trum shown in Figure 8. It appears as if the sequence of elution progresses from less polar and lower boiling substances to more polar and higher boiling substances as shown in Table 1. This was expected using the Rxi-5Sil column for GC-MS analyses. The toxicity from orelline to orellanine progressed from the low-molecular-weight orelline as nontoxic to the higher-molecular-weight orellanine as the most toxic in the sequence. Because of this, it is expected that rubelline, with the highest molecular weight, is most toxic, as was described for trivalent heterocyclic nitrogen and penta- valent nitrogen alkaloids [21,22]. The hypothesis is: the toxicity from orelline to rubelline should progress from the low-molecular-weight orelline as nontoxic to the higher-molecular-weight rubelline as the most toxic in the sequence. Examples of biological transformation of less polar alkaloids to polar-charged molecules are known. These polar-charged molecules have a higher boiling point. An example of this is the transformation of (S)-canadine to berberine Figure 9. The tentatively elucidated structural formula for rubel- line is shown in Figure 10. Orellanine and rubelline are similar and they are re- lated structurally to paraquat and diquat. Paraquat and diquat are nonselective bipyridyl herbicides; rubelline exhibits the herbicide cytotoxic effect. As stated by Jones and Vale [23] “Diquat is a potent redox cycler and is readily converted to a free radical which, in reaction with molecular o xygen, generates superoxide anions and subsequently other redox products. These products can induce lipid peroxidation in cell membranes, and poten- tially cause cell death.” The cytotoxic effect of rubelline was tested in a specific model [19] on unicellular algae Chlamydomonas reinhardtii. The model was developed to test the ability of primaquine and quinocide to inter- fere with the electron transport chain, to scavenge elec- trons, and to produce peroxides and radicals in the living cell. 4. Conclusions 1) The molecular weight of rubelline is 256 and the structure of rubelline was tentatively elucidated using GC-MS. 2) Rubelline is less polar than orellanine and is conse- quently more suitable for absorption in the stomach and digestive tract and transport in the blood to reach target organs such as the liver and the kidneys. 3) Rubelline is converted in mitochondria to orellanine and gradually concentrates in the kidney. 4) The biotransformation of rubelline to orellanine impairs kidney function by interference with electron transport, by production of radicals and peroxides, and
 I. BRONDZ 116 Table 1. The characteristics for: rubelline, orelline, orellinine and orellanine. Peak A B C D Rubelline: CAS No. Orelline: CAS No: 72016-31-0 Orellinine: CAS No.: 98726-96-6Orellanine: CAS No.: 37338-80-0 Molecular Weight: 256.1 Molecular Weight: 220.1815 Molecular Weight:236.1809 Molecular Weight:252.17 Boiling Point: not known Boiling Point: 257.4˚C at 760 mm HgBoiling Point: 282˚C at 760 mm HgBoiling Point: 834.615˚C at 760 mm Hg Formula: C10H12N2O6 Formula:C10H8N2O4 Formula: C10H8N2O5 Formula: C10H8N2O6 Retention time Rubelline Orelline Orellinine Orellanine 5.38 min 5.84 min 6.92 min 8.05 min Figure 9. The transformation of (S)-canadine to berberine. by direct toxicity. 5) Orellanine is a toxic substance that gradually con- centrates in the target organs until the concentration be- comes such that critical irreversible damage occurs. 6) The process of biotransformation from rubelline to orellanine takes time and this may explain the long-latent period of Cortinarius poisoning. Individual differences in enzyme activity in different patients may explain the difference in the latent period: in some patients this is 2 days, in others up to 3 weeks. 7) The discovery of a molecule with m/z 256 suggests that a molecule with m/z 580 may exist. A hypothetical molecule with m/z 580 should have the structure shown in Figure 11. 5. Acknowledgements The author is grateful to Jupiter Ltd., Norway, for finan- cial support, biologic material and standards, to Professor Aviv Amirav, School of Chemistry, Tel Aviv University, Tel Aviv, Israel for technical assistance with gas chro- matography-mass spectrometry and to Anton Brondz, Open Access IJAMSC
 I. BRONDZ 117 Figure 10. The possible biotransformation of rubelline to orellanine. Figure 11. The hypothetical molecule with m/z 580 possibly can be found in C. orellanus or C. rubellus. Department of Chemistry, Norwegian University of Sci- ence and Technology, Trondheim, Norway, for the pre- paration of the figures. REFERENCES [1] S. Grzymala, “Massenvergiftungen durch den Orangefuch- sigen Hautkopf,” Zeitschriftfür Pilzkunde, Vol. 23, 1957, pp. 139-142. [2] I. Brondz, E. Nevo, S. P. Wasser, K. Høiland, F. P. Størmer and A. C. Brondz, “Method Development for in Vivo Analyses of Toxin Orellanine from the Toad Mush- room Cortinarius orellanus,” Separation Science Europe 2011, 10-11 October 2011, The Royal Institution of Great Britain, London, Abstract 9. [3] I. Brondz, E. Nevo, S. Wasser and A. Brondz, “A Direct Gas Chromatography-Mass Spectrometry (GC-MS) Method for the Detection of Orellanine Present in Stomach Con- tent. Part I,” Journal of Biophysical Chemistry, Vol. 3, No. 1, 2012, pp. 29-34. http://dx.doi.org/10.4236/jbpc.2012.31003 [4] I. Brondz and A. Brondz, “A High Performance Liquid Chromatography-Mass Spectrometry (HPLC-MS) Quali- tative Detection Method Developed for in Vivo Analyses of Toxin Orellanine from the Cortinarius orellanus Fr. —Part II,” ISRN Chromatography, Vol. 2012, 2012, Ar- ticle ID 293830. [5] I. Brondz, “Fungi, Classification of the Basidiomycetes,” In: C. Batt and M. L. Tortorello, Eds., Encyclopedia of Food Microbiology, 2nd Edition, Elsevier, Oxford, 2014, in Press. [6] T. Schumacher and K. Høiland, “Review Mushroom Poisoning Caused by Species of the Genus Cortinarius Fries,” Archives of Toxicology, Vol. 53, 1983, pp. 87-106. [7] I. R. Tebbett and B. Caddy, “Mushroom Toxins of Genus Cortinarius,” Experientia, Vol. 40, No. 5, 1984, pp. 441- 446. http://dx.doi.org/10.1007/BF01952379 [8] L. Matthies and H. Laatsch, “Cortinarins in Cortinarius speciosissimus? A Critical Revision,” Experientia, Vol. 47, No. 6, 1991, pp. 634-640. http://dx.doi.org/10.1007/BF01949895 [9] M. Rohrmoser, M. Kirchmair, E. Feifel, A. Valli, R. Cor- radini, E. Pohanka, A. Rosenkranz and R. Pöder, “Orella- nine Poisoning: Rapid Detection of the Fun-Gal Toxin in Renal Biopsy Material,” Journal of Toxicology—Clinical Toxicology, Vol. 35, No. 1, 1997, pp. 63-66. http://dx.doi.org/10.3109/15563659709001167 [10] S. Rapior, N. Delpech, C. Andary and G. Huchhard, “In- toxication by Cortinarius orellanus: Detection and Assay of Orellanine in Biological Fluids and Renal Biopsies,” Mycopathologia, Vol. 108, No. 3, 1989, pp. 155-161. http://dx.doi.org/10.1007/BF00436220 [11] W. Z. Antkowiak, R. Antkowiak, E. Wyrzykiewicz and G. Czerwiński, “Mass Spectral Fragmentation of Orellanine and Its Tetramethyl Ether with Regard to Their Facile Thermal and Photochemical Deoxygenation,” Heterocy- cles, Vol. 39, No. 2, 1994, pp. 477-484. http://dx.doi.org/10.3987/COM-94-S(B)72 [12] J. Holmdahl, J. Ahlḿen, S. Bergek, S. Lundberg, S.-A. Persson, “Isolation and Nephrotoxic Studies of Orellanine from the Mushroom Cortinarius speciosissmus,” Toxicon, Vol. 25, No. 2, 1987, pp. 195-199. http://dx.doi.org/10.1016/0041-0101(87)90241-8 [13] P. Spiteller, M. Spiteller and W. Steglich, “Occurrence of the Fungal Toxin Orellanine as a Diglucoside and Inves- tigation of Its Biosynthesis,” Angewandte Chemie Inter- national Edition, Vol. 42, No. 25, 2003, pp. 2864-2867. http://dx.doi.org/10.1002/anie.200351066 [14] A. Herrmann, H. Hedman, J. Rosén, D. Jansson, B. Ha- raldsson and K.-E. Hellenæs, “Analysis of the Mashroom Nephrotoxin Orellanine and Its Glucosides,” Journal of Natural Products, Vol. 75, No. 10, 2012, pp. 1690-1696. http://dx.doi.org/10.1021/np300135k [15] I. Brondz, A. B. Fialkov and A. Amirav, “Analysis of Quinocide in Unprocessed Primaquine Diphosphate and Primaquine Diphosphate Tablets Using Gas Chromatog- Open Access IJAMSC
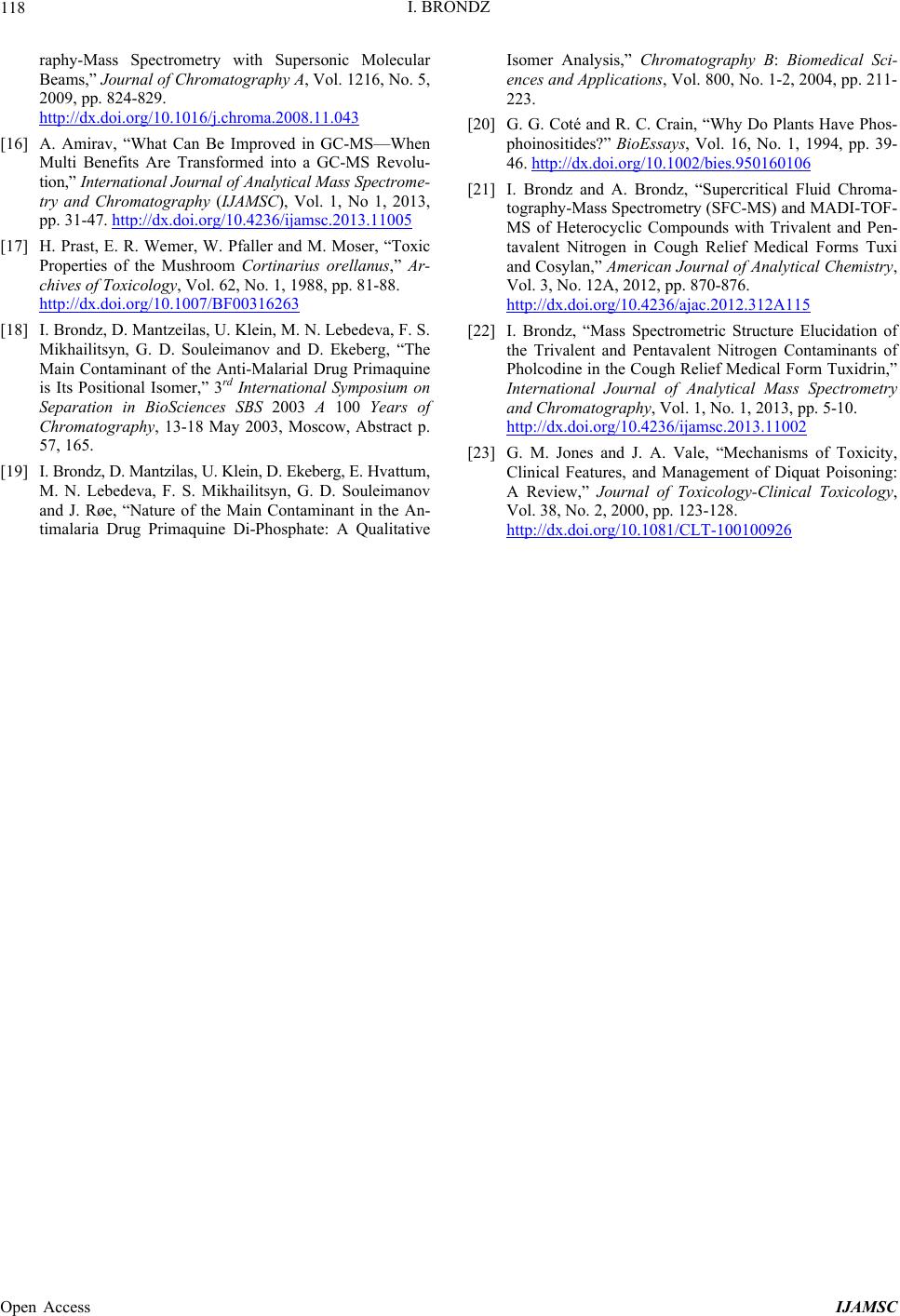 I. BRONDZ Open Access IJAMSC 118 raphy-Mass Spectrometry with Supersonic Molecular Beams,” Journal of Chromatography A, Vol. 1216, No. 5, 2009, pp. 824-829. http://dx.doi.org/10.1016/j.chroma.2008.11.043 [16] A. Amirav, “What Can Be Improved in GC-MS—When Multi Benefits Are Transformed into a GC-MS Revolu- tion,” International Journ al of Analytical Mass Spectr om e- try and Chromatography (IJAMSC), Vol. 1, No 1, 2013, pp. 31-47. http://dx.doi.org/10.4236/ijamsc.2013.11005 [17] H. Prast, E. R. Wemer, W. Pfaller and M. Moser, “Toxic Properties of the Mushroom Cortinarius orellanus,” Ar- chives of Toxicology, Vol. 62, No. 1, 1988, pp. 81-88. http://dx.doi.org/10.1007/BF00316263 [18] I. Brondz, D. Mantzeilas, U. Klein, M. N. Lebedeva, F. S. Mikhailitsyn, G. D. Souleimanov and D. Ekeberg, “The Main Contaminant of the Anti-Malarial Drug Primaquine is Its Positional Isomer,” 3rd International Symposium on Separation in BioSciences SBS 2003 A 100 Years of Chromatography, 13-18 May 2003, Moscow, Abstract p. 57, 165. [19] I. Brondz, D. Mantzilas, U. Klein, D. Ekeberg, E. Hvattum, M. N. Lebedeva, F. S. Mikhailitsyn, G. D. Souleimanov and J. Røe, “Nature of the Main Contaminant in the An- timalaria Drug Primaquine Di-Phosphate: A Qualitative Isomer Analysis,” Chromatography B: Biomedical Sci- ences and Applications, Vol. 800, No. 1-2, 2004, pp. 211- 223. [20] G. G. Coté and R. C. Crain, “Why Do Plants Have Phos- phoinositides?” BioEssays, Vol. 16, No. 1, 1994, pp. 39- 46. http://dx.doi.org/10.1002/bies.950160106 [21] I. Brondz and A. Brondz, “Supercritical Fluid Chroma- tography-Mass Spectrometry (SFC-MS) and MADI-TOF- MS of Heterocyclic Compounds with Trivalent and Pen- tavalent Nitrogen in Cough Relief Medical Forms Tuxi and Cosylan,” American Journal of Analytical Chemistry, Vol. 3, No. 12A, 2012, pp. 870-876. http://dx.doi.org/10.4236/ajac.2012.312A115 [22] I. Brondz, “Mass Spectrometric Structure Elucidation of the Trivalent and Pentavalent Nitrogen Contaminants of Pholcodine in the Cough Relief Medical Form Tuxidrin,” International Journal of Analytical Mass Spectrometry and Chromatography, Vol. 1, No. 1, 2013, pp. 5-10. http://dx.doi.org/10.4236/ijamsc.2013.11002 [23] G. M. Jones and J. A. Vale, “Mechanisms of Toxicity, Clinical Features, and Management of Diquat Poisoning: A Review,” Journal of Toxicology-Clinical Toxicology, Vol. 38, No. 2, 2000, pp. 123-128. http://dx.doi.org/10.1081/CLT-100100926
|