 Neuroscience & Medicine, 2013, 4, 299-318 Published Online December 2013 (http://www.scirp.org/journal/nm) http://dx.doi.org/10.4236/nm.2013.44044 Open Access NM 299 Involvement of CRH Receptors in the Neuroprotective Action of R-Apomorphine in the Striatal 6-OHDA Rat Model Mustafa Varçin1, Eduard Bentea1, Steven Roosens1, Yvette Michotte1, Sophie Sarre1,2* 1Department of Pharmaceutical Chemistry and Drug Analysis, Center for Neurosciences, Vrije Universiteit Brussel, Faculty of Medicine and Pharmacy, Brussels, Belgium; 2Belgian Pharmacists Association, Medicines Control Laboratory, Brussels, Belgium. Email: *Sophie.Sarre@apb.be Received October 21st, 2013; revised November 20th, 2013; accepted December 10th, 2013 Copyright © 2013 Mustafa Varçin et al. This is an open access article distributed under the Creative Commons Attribution License, which permits unrestricted use, distribution, and reproduction in any medium, provided the original work is properly cited. ABSTRACT The dopamine D1-D2 receptor agonist, R-apomorphine, has been shown to be neuroprotective in different models of Parkinson’s disease. Different mechanisms of action for this effect have been proposed, but not verified in the striatal 6-hydroxydopamine rat model. In this study, the expression of a set of genes involved in 1) signaling, 2) growth and differentiation, 3) neuronal regeneration and survival, 4) apoptosis and 5) inflammation in the striatum was measured after a subchronic R-apomorphine treatment (10 mg/kg/day, subcutaneously, during 11 days) in the striatal 6-hydroxy- dopamine rat model. The expression of 84 genes was analysed by using the rat neurotrophins and receptors RT2 Pro- filer™ PCR array. The neuroprotective effects of R-apomorphine in the striatal 6-hydroxydopamine model were con- firmed by neurochemical and behavioural analysis. The expression data suggest the observed neuroprotection involved the alteration of the gene and the protein expression levels of the anti-inflammatory corticotropin releasing hormone receptor (CRHR) 1 and the pro-inflammatory CRHR2 receptor confirming its potential anti-inflammatory action. Keywords: Apomorphine; Gene Expression; Inflammation; Neuroprotection; Parkinson’s Disease; Striatal 6-Hydroxydopamine Rat Model 1. Introduction Until today, the available treatments for Parkinson’s dis- ease do not stop or slow down the progressive nature of the disease and are based on dopamine (DA) replacement strategies, such as the use of DA agonists and/or the DA precursor, levodopa (L-DOPA) [1]. Due to the long pre- symptomatic phase of the disease, therapeutic intervene- tions that result in the protection, restoration and/or res- cuing of the dopaminergic neurons are of extreme im- portance to improve the quality of life of the patients [1,2]. Although R-apomorphine has been introduced as a drug a certain time ago, it is still available as treatment for patients with Parkinson’s disease and with advanced Parkinson’s disease and for the treatment of persistent and disabling motor fluctuations which do not respond to L-DOPA [3-7]. It activates D1-like (D1, D5) and D2-like (D2, D3, D4) receptors, serotonin receptors (5HT1A, 5HT2A, 5HT2B and 5HT2C) and α-adrenergic receptors (α1B, α1D, α2A, α2B, α2C) [8]. R-apomorphine has been shown to be neuroprotective both in vitro and in vivo, including the 1-methyl-4-phenyl- 1,2,3,6-tetrahydropyridine (MPTP) [9-12], 6-hydroxy- dopamine (6-OHDA) [13-15] and methamphetamine (METH) [12,16] rodent models of Parkinson’s disease and more recently, a mouse model of Alzheimer’s dis- ease [17]. The in vivo neuroprotective properties of R- apomorphine have been shown to be dose dependent, and mainly linked to the administration of high doses of R- apomorphine. These findings also suggest that the neu- roprotective action may involve other pathways rather than activation of DA receptors. Among the proposed mechanisms of action are its radical scavenging activity [13,15,18,19] and iron chelating properties [20], inhibi- *Corresponding author.
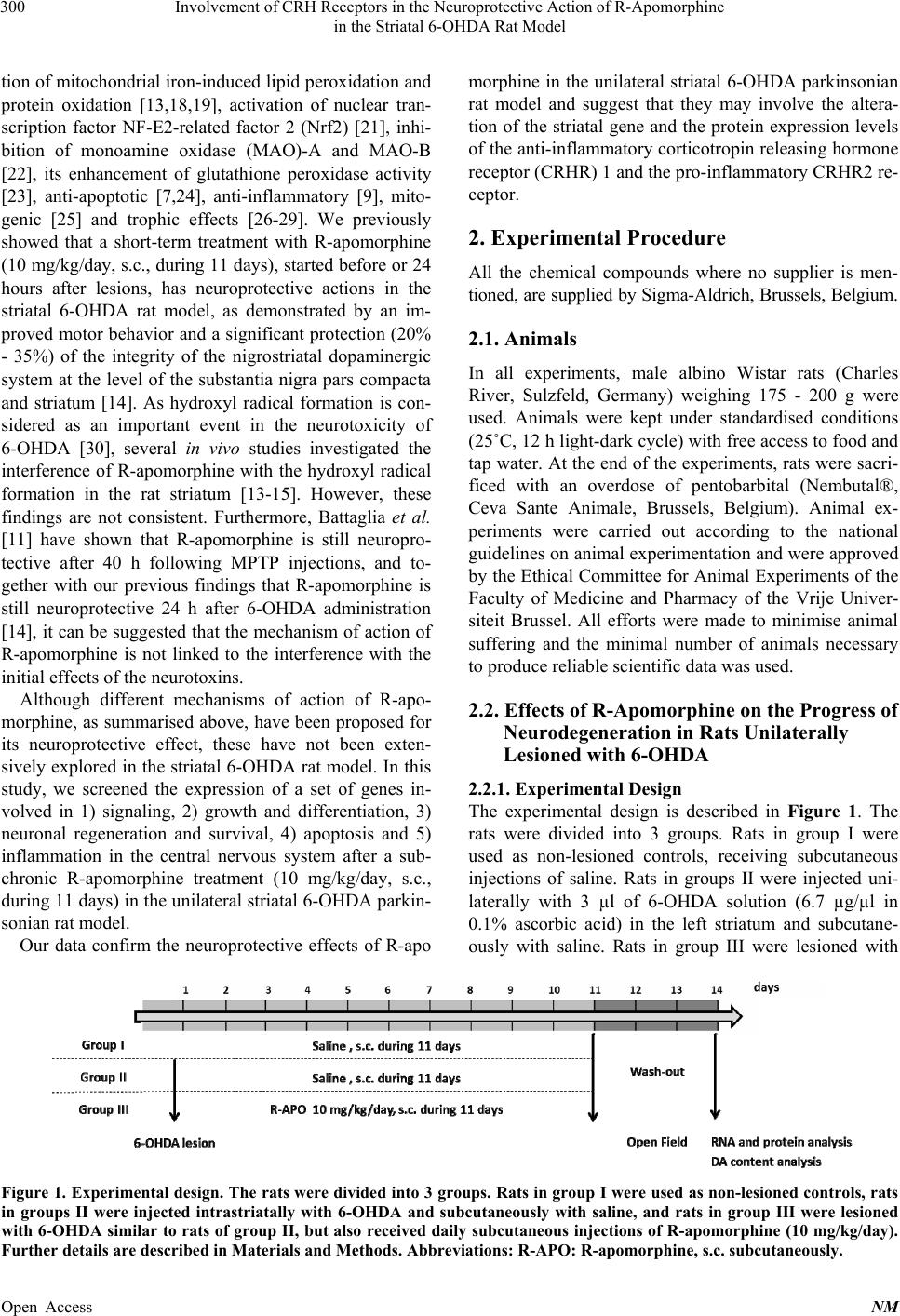 Involvement of CRH Receptors in the Neuroprotective Action of R-Apomorphine in the Striatal 6-OHDA Rat Model 300 tion of mitochondrial iron-induced lipid peroxidation and protein oxidation [13,18,19], activation of nuclear tran- scription factor NF-E2-related factor 2 (Nrf2) [21], inhi- bition of monoamine oxidase (MAO)-A and MAO-B [22], its enhancement of glutathione peroxidase activity [23], anti-apoptotic [7,24], anti-inflammatory [9], mito- genic [25] and trophic effects [26-29]. We previously showed that a short-term treatment with R-apomorphine (10 mg/kg/day, s.c., during 11 days), started before or 24 hours after lesions, has neuroprotective actions in the striatal 6-OHDA rat model, as demonstrated by an im- proved motor behavior and a significant protection (20% - 35%) of the integrity of the nigrostriatal dopaminergic system at the level of the substantia nigra pars compacta and striatum [14]. As hydroxyl radical formation is con- sidered as an important event in the neurotoxicity of 6-OHDA [30], several in vivo studies investigated the interference of R-apomorphine with the hydroxyl radical formation in the rat striatum [13-15]. However, these findings are not consistent. Furthermore, Battaglia et al. [11] have shown that R-apomorphine is still neuropro- tective after 40 h following MPTP injections, and to- gether with our previous findings that R-apomorphine is still neuroprotective 24 h after 6-OHDA administration [14], it can be suggested that the mechanism of action of R-apomorphine is not linked to the interference with the initial effects of the neurotoxins. Although different mechanisms of action of R-apo- morphine, as summarised above, have been proposed for its neuroprotective effect, these have not been exten- sively explored in the striatal 6-OHDA rat model. In this study, we screened the expression of a set of genes in- volved in 1) signaling, 2) growth and differentiation, 3) neuronal regeneration and survival, 4) apoptosis and 5) inflammation in the central nervous system after a sub- chronic R-apomorphine treatment (10 mg/kg/day, s.c., during 11 days) in the unilateral striatal 6-OHDA parkin- sonian rat model. Our data confirm the neuroprotective effects of R-apo morphine in the unilateral striatal 6-OHDA parkinsonian rat model and suggest that they may involve the altera- tion of the striatal gene and the protein expression levels of the anti-inflammatory corticotropin releasing hormone receptor (CRHR) 1 and the pro-inflammatory CRHR2 re- ceptor. 2. Experimental Procedure All the chemical compounds where no supplier is men- tioned, are supplied by Sigma-Aldrich, Brussels, Belgium. 2.1. Animals In all experiments, male albino Wistar rats (Charles River, Sulzfeld, Germany) weighing 175 - 200 g were used. Animals were kept under standardised conditions (25˚C, 12 h light-dark cycle) with free access to food and tap water. At the end of the experiments, rats were sacri- ficed with an overdose of pentobarbital (Nembutal®, Ceva Sante Animale, Brussels, Belgium). Animal ex- periments were carried out according to the national guidelines on animal experimentation and were approved by the Ethical Committee for Animal Experiments of the Faculty of Medicine and Pharmacy of the Vrije Univer- siteit Brussel. All efforts were made to minimise animal suffering and the minimal number of animals necessary to produce reliable scientific data was used. 2.2. Effects of R-Apomorphine on the Progress of Neurodegeneration in Rats Unilaterally Lesioned with 6-OHDA 2.2.1. Experimental Design The experimental design is described in Figure 1. The rats were divided into 3 groups. Rats in group I were used as non-lesioned controls, receiving subcutaneous injections of saline. Rats in groups II were injected uni- laterally with 3 µl of 6-OHDA solution (6.7 µg/µl in 0.1% ascorbic acid) in the left striatum and subcutane- ously with saline. Rats in group III were lesioned with Figure 1. Experimental design. The rats were divided into 3 groups. Rats in group I were used as non-lesioned controls, rats in groups II were injected intrastriatally with 6-OHDA and subcutaneously with saline, and rats in group III were lesioned with 6-OHDA similar to rats of group II, but also received daily subcutaneous injections of R-apomorphine (10 mg/kg/day). Further details are described in Materials and Methods. Abbreviations: R-APO: R-apomorphine, s.c. subcutaneously. Open Access NM
 Involvement of CRH Receptors in the Neuroprotective Action of R-Apomorphine in the Striatal 6-OHDA Rat Model 301 6-OHDA similar to rats of group II, but also received daily subcutaneous injections of R-apomorphine (10 mg/ kg/day). The treatment with R-apomorphine started 15 min before the intrastriatal injection of 6-OHDA and continued for another 10 days. The dose of the R-apo- morphine was based on previous findings [10,11,14]. Briefly, Grünblatt et al had already demonstrated that a low dose (0.5, 1, 2, and 2.5 mg/kg) of R-apomorphine does not protect against MPTP induced DA loss in mice, whereas the higher dose (10 mg/kg) restored the values to those of control mice [10]. Similar findings were re- ported in 2001 [22]. Furthermore, Fornai et al demon- strated a dose dependent neuroprotective effect of R- apomorphine in methamphetamine induced DA depletion. Based on these findings, we decided to use 10 mg/kg R- apomorphine in the 6-OHDA striatal rat model. Animals were visually checked at regular intervals and weighed daily before any manipulation until the end of the ex- periment (day 14). For all the groups there was a wash- out period of 3 days. Different sets of rats were used for the initial screening of the relative striatal gene expres- sion levels on the one hand, and behavioural testing, as- sessment of DA and DOPAC content, and protein ex- pression analysis on the other. mRNA of high quality was used during the gene expression studies, and there- fore the purity and integrity of all mRNA samples were checked with advanced methods, such as UV spectro- photometry and a microfluidics based electrophoresis system. The specificity of the PCR reaction has been evaluated by analysing the PCR product (the amplicon) by conducting at the end of each RTqPCR experiment a melt curve analysis for each amplicon. The web based software of the company (SA Biosciences, MD) also automatically performed a quality control for each PCR run. The quality of the reference genes has been evalu- ated by the software program that was provided by the supplier (SA Biosciences, MD) of the PCR arrays. The selection of the potential candidate genes for the protein expression analysis was based on experimental and lit- erature findings. To mitigate biological and technical variabilities during a gene expression study, the recom- mended number of biological replicates is minimum three, and for technical replicates minimum two [31]. We managed to have the minimum number of biological rep- licates for the gene expression study, but unfortunately technical replicates were not possible due to the limited sample volume. Despite a lower number of animals for the gene expression studies, our slightly higher number of animals for the protein expression favours our obser- vations at the gene level. 2.2.2. Local Administration of 6-OHDA by Striatal Stereotaxic Microinjection Rats were anaesthesized with a mixture of ketamine (50 mg/kg i.p.; Ketamine 1000 Ceva®, Ceva Sante Animale, Brussels, Belgium) and diazepam (5 mg/kg i.p.; Valium®, Roche Brussels, Belgium) and placed on a Kopf stereo- taxic frame (David Kopf Instruments, Tujunga, Califor- nia, USA). The skull was exposed and a burr hole was drilled to introduce a syringe for a single injection of the 6-OHDA solution [containing 6.7 µg 6-OHDA per µl in 0.1% ascorbic acid, pH 5.0]. To minimize variability due to degradation of the toxin, the 6-OHDA solution was freshly prepared, kept on ice, and protected from expo- sure to light. The solution was injected in the left stria- tum at the following coordinates relative to the bregma L: −3.0, A: +1.0 and V: +5.0, according to the atlas of Pax- inos and Watson [32]. A total volume of 3 µl 6-OHDA was injected at a flow rate of 1 µl/min. After injection, the syringe was left in place for 5 min and then slowly removed over a 1 - 2 min time period. The skin was su- tured, the animals received ketoprofen (4 mg/kg i.p.; Ketofen®, Merial, Brussels, Belgium) as analgesic and were allowed to recover before returning to the animal housing facilities [14]. 2.3. Behavioral Analysis Locomotor activity in Open Field The spontaneous locomotor activity was monitored in a Plexiglas box (sides, 60 cm; height, 60 cm). At least 1 h prior to testing, rats were acclimated to the testing room. The recording started immediately after placing animals in the open field and continued for 60 min. To neutralise odor formation, the arena was disinfected and cleaned with 70% ethanol before each rat was tested. Experi- ments were performed between 09:00 AM and 06:00 PM. The rat was placed carefully in the center of the arena, and allowed to explore the field for 60 min. Each per- formance was automatically analysed using a video tracking system and Ethovision 3.0 tracking software (Noldus, the Netherlands). The following parameters were recorded: 1) distance moved, 2) movement time, 3) immobility, 4) velocity, 5) rearing and 6) relative mean- der. The assessment of spontaneous locomotor behavior was carried out during the wash-out period of the proto- col, in order to avoid a possible modulatory effect of the DA agonist R-apomorphine on motor function, as previ- ous studies have shown that following DA agonists sig- nificant alteration of spontaneous motor behavior occurs [33,34]. We preferred to use the open field test in this study to evaluate the animals in a drug-free state. Indeed, amphetamine induced rotation might influence the ex- pression of genes, and our previous results already indi- cate that a subchronic R-apomorphine treatment of 6- OHDA striatally lesioned rats reduced the amphetamine induced ipsilateral rotations [14]. Open Access NM
 Involvement of CRH Receptors in the Neuroprotective Action of R-Apomorphine in the Striatal 6-OHDA Rat Model 302 2.4. Sacrifice and Brain Prelevation Seventy-two hours (wash-out period) after the last inject- tion of R-apomorphine (day 14), the rats were killed with an overdose of pentobarbital (Nembutal®, Ceva Sante Animale, Brussels, Belgium) and the brains were quickly removed without perfusion. From the rostral part (10 mm from front) of the brain the left and right striatum were dissected out on an ice cold chilled petri-dish, immedi- ately snap frozen in dry-ice cooled isopentane and stored separately at −80˚C until homogenisation and analysis of 1) striatal DA and DOPAC content, 2) striatal gene ex- pression and 3) protein expression of CRHR1, CRHR2, and neuropeptide Y (NPY) receptors 1 and 2. 2.5. Neurochemical Determination of the Striatal DA and DOPAC Content To establish the extent of DA and DOPAC depletion in the striatum after 6-OHDA lesioning, the liquid chroma- tography (LC) method previously described was used [14], with slight modifications. The supernatant obtained during protein expression analysis (4.7) was diluted 5 times in antioxidant (0.05 M HCl, 0.5% Na2S2O5 and 0.05% Na2EDTA). 20 µl of samples were injected and analysed directly for DA and DOPAC content on a narrowbore (C18 column: 15 m, 150 mm × 2.1 mm; Altima; Grace; Lokeren; Belgium) LC system. The mobile phase consisted of 0.1 M sodium acetate trihydrate, 20 mM citric acid monohydrate, 1 mM 1-octane sulfonic acid, 0.1 mM Na2EDTA and 1 mM dibutylamine, adjusted to pH 3.7. Methanol 3% (v/v) was added as organic modifier. The flow rate was set at 0.2 ml/min. The electrochemical detection (Antec, The Netherlands) potential was +700 mV versus the reference electrode (Ag/AgCl). Sensitivity was set at 1 nA full scale. All samples were injected via a high precision auto-injector equipped with a cooling system (Kontron, San Diego, CA, USA). The integration of the chroma- tograms was done with the Data Apex Clarity software program (Antec). The tissue DA and DOPAC content were calculated and expressed as µg/g wet weight of tissue. 2.6. Gene Expression Analysis 2.6.1. RNA Extraction Total RNA was extracted from the striatal tissue using the RNeasy® Lipid Tissue Mini Kit according to the manufacturer’s protocol (Qiagen, Venlo, The Nether- lands). The concentration and purity of RNA were de- termined by measuring the absorbance using the Nano- drop 1000 (Thermo Scientific). The quality of total RNA was assessed using the Agilent® Bioanalyzer RNA 6000 Nano Labchip® (Agilent Technologies, Palo Alto, CA). For each RNA sample the presence of sharp bands/peaks present for both the 18S and 28S ribosomal RNAs were verified and only the samples with an RNA Integrity Number of 7 or higher were used. One microgram of total RNA was subjected to first strand cDNA synthesis using the RT2 First Strand Kit (SA Biosciences, MD). For real-time PCR, a PCR com- ponents mix consisting of 2× RT2 SYBR Green Fluor qPCR Mastermix (1350 µl) (SA Biosciences), cDNA synthesis reaction (102 µl) and RNase-free water (1248 µl) was prepared. The final volume was 2700 µl, provid- ing an excess volume to perform pipetting steps as pre- cisely as possible to ensure that each well of the array receives the required volume. 25 µl of the mixture was added into each of the wells of the RT2 Profiler PCR Ar- rays (PARN-031, SA Biosciences, MD) 2.6.2. Quantitative Real-Time PCR Real-time quantifications were performed using the Bio- Rad® iCycler® Real-time PCR system (Bio-Rad) using the recommended cycling conditions for the RT2 Profiler PCR arrays (SA Biosciences, MD). Each PCR array contained 84 transcripts, a set of five housekeeping genes as internal controls and additional controls for efficiency of reverse transcription, PCR and the absence of con- taminating genomic DNA. Relative expression was determined with the ∆∆ CT method using the PCR Array Data Analysis Web Portal (www.SABiosciences.com/pcrarraydataanalysis.php) and the web based software automatically performed quanti- fication, including the quality control for each PCR run/ array. For normalization of the expression levels of the genes of interest, the average CT value of the five house- keeping genes: Ribosomal protein, large, P1 (Rplp1); hypoxanthine phosphoribosyltransferase 1 (Hprt1); ribo- somal protein L13A (Rpl13a); lactate dehydrogenase A (Ldha) and β-actin (Actb) was chosen. If the fold-change was greater than 1, the result is reported as a fold up- regulation. If the fold-change was less than 1, the nega- tive inverse of the results is reported as a fold downregu- lation. 2.7. Protein Expression Analysis To measure CRHR1, CRHR2, NPY1R and NPY2R pro- tein levels, the striatal tissue was homogenised in an ice- cold PBS-solution (0.02 M, pH 7.0 - 7.2) containing 2% protease inhibitor cocktail (Sigma, St-Louis, MO, USA) (25 mg tissue/ml) and subsequently sonicated for 1 min (Branson Sonifier 250). After centrifugation at 10,000 g at 4˚C during 10 minutes (Sorvall RC5B refrigated su- perspeed centrifuge, Dupont Instruments), the super- natants were collected and stored at −20˚C until use. Af- ter decantation, the protein content of the supernatant Open Access NM
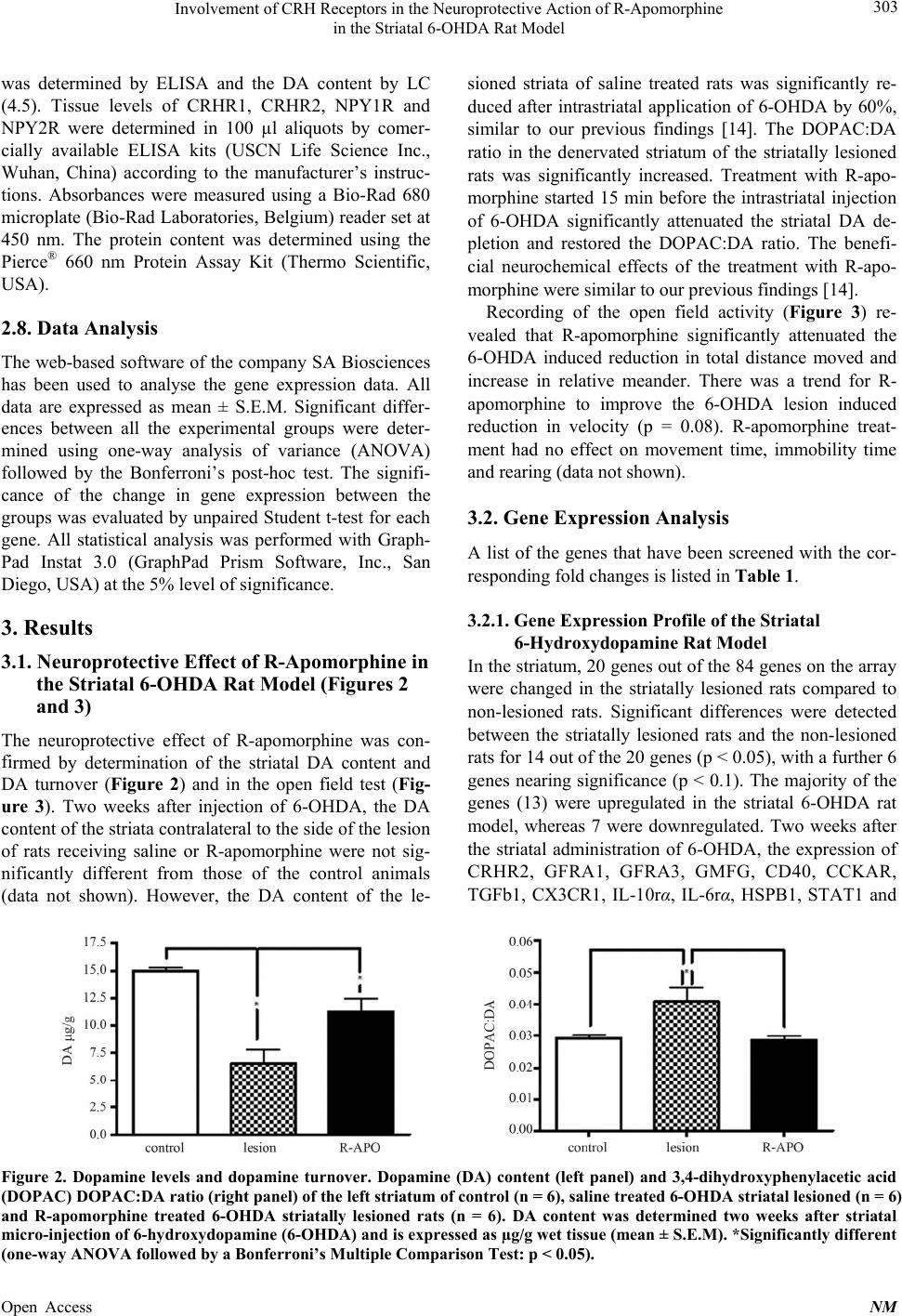 Involvement of CRH Receptors in the Neuroprotective Action of R-Apomorphine in the Striatal 6-OHDA Rat Model Open Access NM 303 was determined by ELISA and the DA content by LC (4.5). Tissue levels of CRHR1, CRHR2, NPY1R and NPY2R were determined in 100 µl aliquots by comer- cially available ELISA kits (USCN Life Science Inc., Wuhan, China) according to the manufacturer’s instruc- tions. Absorbances were measured using a Bio-Rad 680 microplate (Bio-Rad Laboratories, Belgium) reader set at 450 nm. The protein content was determined using the Pierce® 660 nm Protein Assay Kit (Thermo Scientific, USA). sioned striata of saline treated rats was significantly re- duced after intrastriatal application of 6-OHDA by 60%, similar to our previous findings [14]. The DOPAC:DA ratio in the denervated striatum of the striatally lesioned rats was significantly increased. Treatment with R-apo- morphine started 15 min before the intrastriatal injection of 6-OHDA significantly attenuated the striatal DA de- pletion and restored the DOPAC:DA ratio. The benefi- cial neurochemical effects of the treatment with R-apo- morphine were similar to our previous findings [14]. Recording of the open field activity (Figure 3) re- vealed that R-apomorphine significantly attenuated the 6-OHDA induced reduction in total distance moved and increase in relative meander. There was a trend for R- apomorphine to improve the 6-OHDA lesion induced reduction in velocity (p = 0.08). R-apomorphine treat- ment had no effect on movement time, immobility time and rearing (data not shown). 2.8. Data Analysis The web-based software of the company SA Biosciences has been used to analyse the gene expression data. All data are expressed as mean ± S.E.M. Significant differ- ences between all the experimental groups were deter- mined using one-way analysis of variance (ANOVA) followed by the Bonferroni’s post-hoc test. The signifi- cance of the change in gene expression between the groups was evaluated by unpaired Student t-test for each gene. All statistical analysis was performed with Graph- Pad Instat 3.0 (GraphPad Prism Software, Inc., San Diego, USA) at the 5% level of significance. 3.2. Gene Expression Analysis A list of the genes that have been screened with the cor- responding fold changes is listed in Table 1. 3.2.1. Gene Expression Profile of the Striatal 6-Hydroxydopamine Rat Model 3. Results 3.1. Neuroprotective Effect of R-Apomorphine in the Striatal 6-OHDA Rat Model (Figures 2 and 3) In the striatum, 20 genes out of the 84 genes on the array were changed in the striatally lesioned rats compared to non-lesioned rats. Significant differences were detected between the striatally lesioned rats and the non-lesioned rats for 14 out of the 20 genes (p < 0.05), with a further 6 genes nearing significance (p < 0.1). The majority of the genes (13) were upregulated in the striatal 6-OHDA rat model, whereas 7 were downregulated. Two weeks after the striatal administration of 6-OHDA, the expression of CRHR2, GFRA1, GFRA3, GMFG, CD40, CCKAR, TGFb1, CX3CR1, IL-10rα, IL-6rα, HSPB1, STAT1 and The neuroprotective effect of R-apomorphine was con- firmed by determination of the striatal DA content and DA turnover (Figure 2) and in the open field test (Fig- ure 3). Two weeks after injection of 6-OHDA, the DA content of the striata contralateral to the side of the lesion of rats receiving saline or R-apomorphine were not sig- nificantly different from those of the control animals data not shown). However, the DA content of the le- ( Figure 2. Dopamine levels and dopamine turnover. Dopamine (DA) content (left panel) and 3,4-dihydroxyphenylacetic acid (DOPAC) DOPAC:DA ratio (right panel) of the left striatum of control (n = 6), saline treated 6-OHDA striatal lesioned (n = 6) and R-apomorphine treated 6-OHDA striatally lesioned rats (n = 6). DA content was determined two weeks after striatal micro-injection of 6-hydroxydopamine (6-OHDA) and is expressed as µg/g wet tissue (mean ± S.E.M). *Significantly different (one-way ANOVA followed by a Bonferroni’s Multiple Comparison Test: p < 0.05).
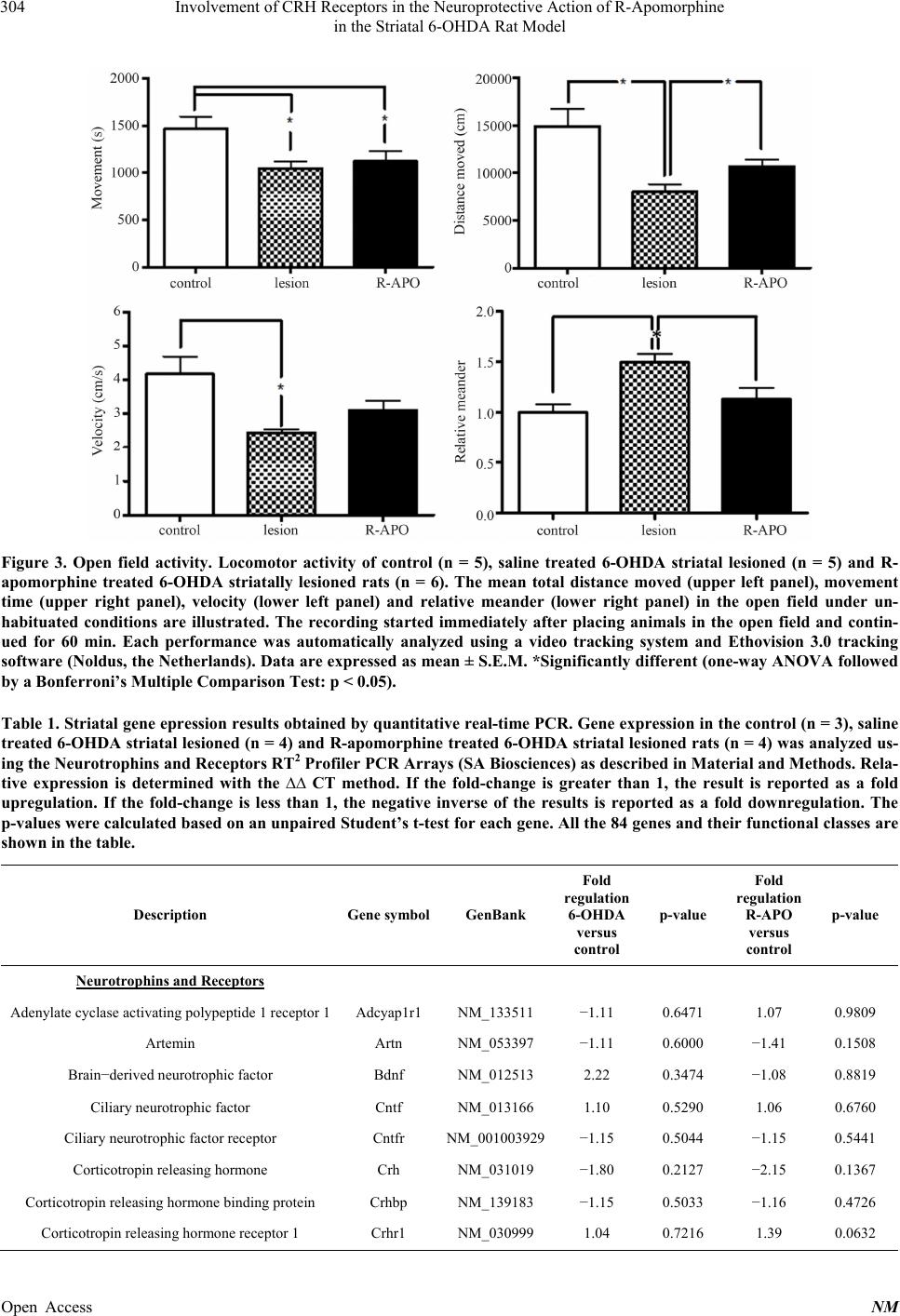 Involvement of CRH Receptors in the Neuroprotective Action of R-Apomorphine in the Striatal 6-OHDA Rat Model 304 Figure 3. Open field activity. Locomotor activity of control (n = 5), saline treated 6-OHDA striatal lesioned (n = 5) and R- apomorphine treated 6-OHDA striatally lesioned rats (n = 6). The mean total distance moved (upper left panel), movement time (upper right panel), velocity (lower left panel) and relative meander (lower right panel) in the open field under un- habituated conditions are illustrated. The recording started immediately after placing animals in the open field and contin- ued for 60 min. Each performance was automatically analyzed using a video tracking system and Ethovision 3.0 tracking software (Noldus, the Netherlands). Data are expressed as mean ± S.E.M. *Significantly different (one-way ANOVA followed by a Bonferroni’s Multiple Comparison Test: p < 0.05). Table 1. Striatal gene epression results obtained by quantitative real-time PCR. Gene expression in the control (n = 3), saline treated 6-OHDA striatal lesioned (n = 4) and R-apomorphine treated 6-OHDA striatal lesioned rats (n = 4) was analyzed us- ing the Neurotrophins and Receptors RT2 Profiler PCR Arrays (SA Biosciences) as described in Material and Methods. Rela- tive expression is determined with the ∆∆ CT method. If the fold-change is greater than 1, the result is reported as a fold upregulation. If the fold-change is less than 1, the negative inverse of the results is reported as a fold downregulation. The p-values were calculated based on an unpaired Student’s t-test for each gene. All the 84 genes and their functional classes are shown in the table. Description Gene symbolGenBank Fold regulation 6-OHDA versus control p-value Fold regulation R-APO versus control p-value Neurotrophins and Receptors Adenylate cyclase activating polypeptide 1 receptor 1 Adcyap1r1 NM_133511 −1.11 0.6471 1.07 0.9809 Artemin Artn NM_053397 −1.11 0.6000 −1.41 0.1508 Brain−derived neurotrophic factor Bdnf NM_012513 2.22 0.3474 −1.08 0.8819 Ciliary neurotrophic factor Cntf NM_013166 1.10 0.5290 1.06 0.6760 Ciliary neurotrophic factor receptor Cntfr NM_001003929−1.15 0.5044 −1.15 0.5441 Corticotropin releasing hormone Crh NM_031019 −1.80 0.2127 −2.15 0.1367 Corticotropin releasing hormone binding protein Crhbp NM_139183 −1.15 0.5033 −1.16 0.4726 Corticotropin releasing hormone receptor 1 Crhr1 NM_030999 1.04 0.7216 1.39 0.0632 Open Access NM
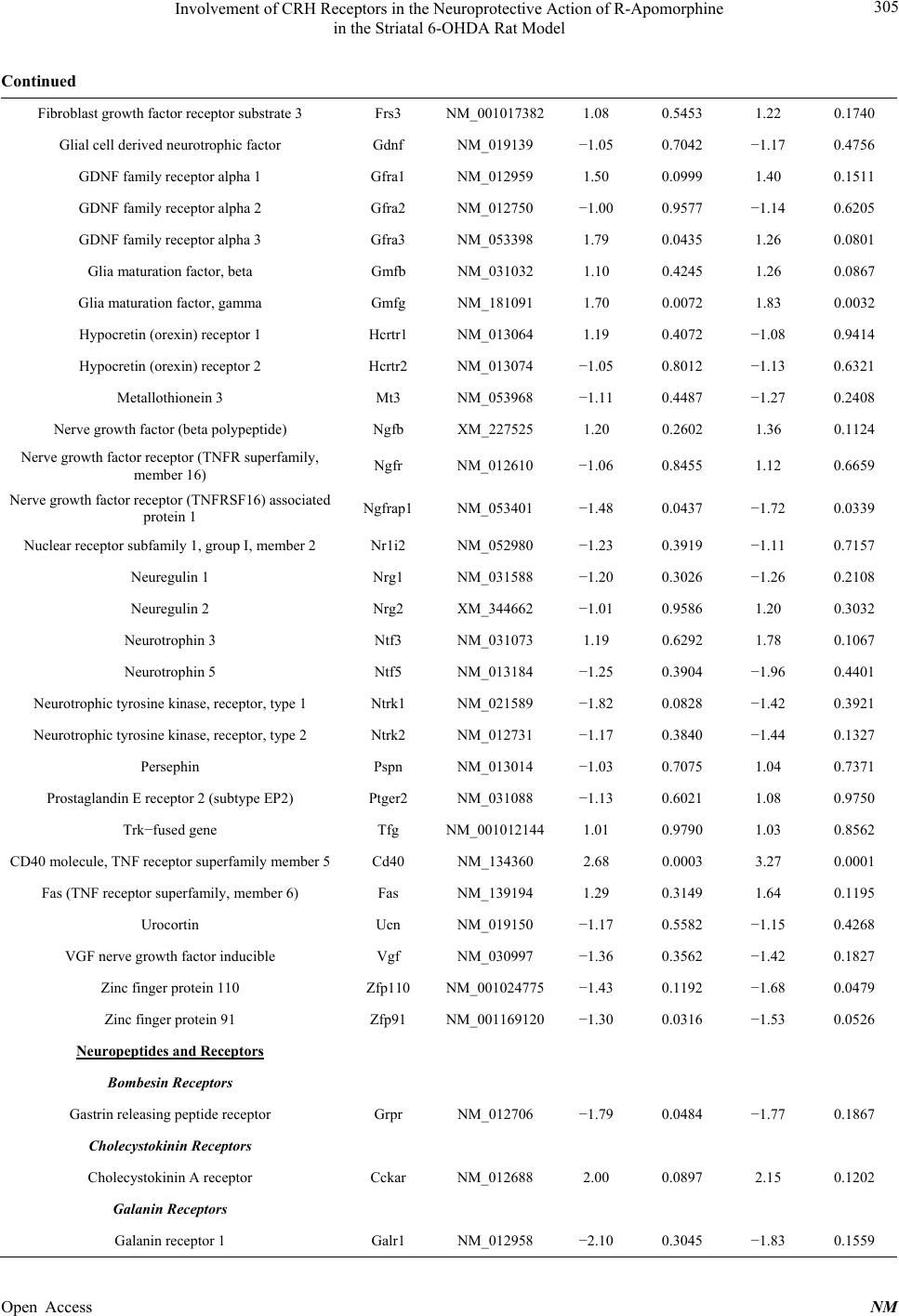 Involvement of CRH Receptors in the Neuroprotective Action of R-Apomorphine in the Striatal 6-OHDA Rat Model 305 Continued Fibroblast growth factor receptor substrate 3 Frs3 NM_0010173821.08 0.5453 1.22 0.1740 Glial cell derived neurotrophic factor Gdnf NM_019139 −1.05 0.7042 −1.17 0.4756 GDNF family receptor alpha 1 Gfra1 NM_012959 1.50 0.0999 1.40 0.1511 GDNF family receptor alpha 2 Gfra2 NM_012750 −1.00 0.9577 −1.14 0.6205 GDNF family receptor alpha 3 Gfra3 NM_053398 1.79 0.0435 1.26 0.0801 Glia maturation factor, beta Gmfb NM_031032 1.10 0.4245 1.26 0.0867 Glia maturation factor, gamma Gmfg NM_181091 1.70 0.0072 1.83 0.0032 Hypocretin (orexin) receptor 1 Hcrtr1 NM_013064 1.19 0.4072 −1.08 0.9414 Hypocretin (orexin) receptor 2 Hcrtr2 NM_013074 −1.05 0.8012 −1.13 0.6321 Metallothionein 3 Mt3 NM_053968 −1.11 0.4487 −1.27 0.2408 Nerve growth factor (beta polypeptide) Ngfb XM_227525 1.20 0.2602 1.36 0.1124 Nerve growth factor receptor (TNFR superfamily, member 16) Ngfr NM_012610 −1.06 0.8455 1.12 0.6659 Nerve growth factor receptor (TNFRSF16) associated protein 1 Ngfrap1 NM_053401 −1.48 0.0437 −1.72 0.0339 Nuclear receptor subfamily 1, group I, member 2 Nr1i2 NM_052980 −1.23 0.3919 −1.11 0.7157 Neuregulin 1 Nrg1 NM_031588 −1.20 0.3026 −1.26 0.2108 Neuregulin 2 Nrg2 XM_344662 −1.01 0.9586 1.20 0.3032 Neurotrophin 3 Ntf3 NM_031073 1.19 0.6292 1.78 0.1067 Neurotrophin 5 Ntf5 NM_013184 −1.25 0.3904 −1.96 0.4401 Neurotrophic tyrosine kinase, receptor, type 1 Ntrk1 NM_021589 −1.82 0.0828 −1.42 0.3921 Neurotrophic tyrosine kinase, receptor, type 2 Ntrk2 NM_012731 −1.17 0.3840 −1.44 0.1327 Persephin Pspn NM_013014 −1.03 0.7075 1.04 0.7371 Prostaglandin E receptor 2 (subtype EP2) Ptger2 NM_031088 −1.13 0.6021 1.08 0.9750 Trk−fused gene Tfg NM_0010121441.01 0.9790 1.03 0.8562 CD40 molecule, TNF receptor superfamily member 5 Cd40 NM_134360 2.68 0.0003 3.27 0.0001 Fas (TNF receptor superfamily, member 6) Fas NM_139194 1.29 0.3149 1.64 0.1195 Urocortin Ucn NM_019150 −1.17 0.5582 −1.15 0.4268 VGF nerve growth factor inducible Vgf NM_030997 −1.36 0.3562 −1.42 0.1827 Zinc finger protein 110 Zfp110 NM_001024775−1.43 0.1192 −1.68 0.0479 Zinc finger protein 91 Zfp91 NM_001169120−1.30 0.0316 −1.53 0.0526 Neuropeptides and Receptors Bombesin Receptors Gastrin releasing peptide receptor Grpr NM_012706 −1.79 0.0484 −1.77 0.1867 Cholecystokinin Receptors Cholecystokinin A receptor Cckar NM_012688 2.00 0.0897 2.15 0.1202 Galanin Receptors Galanin receptor 1 Galr1 NM_012958 −2.10 0.3045 −1.83 0.1559 Open Access NM
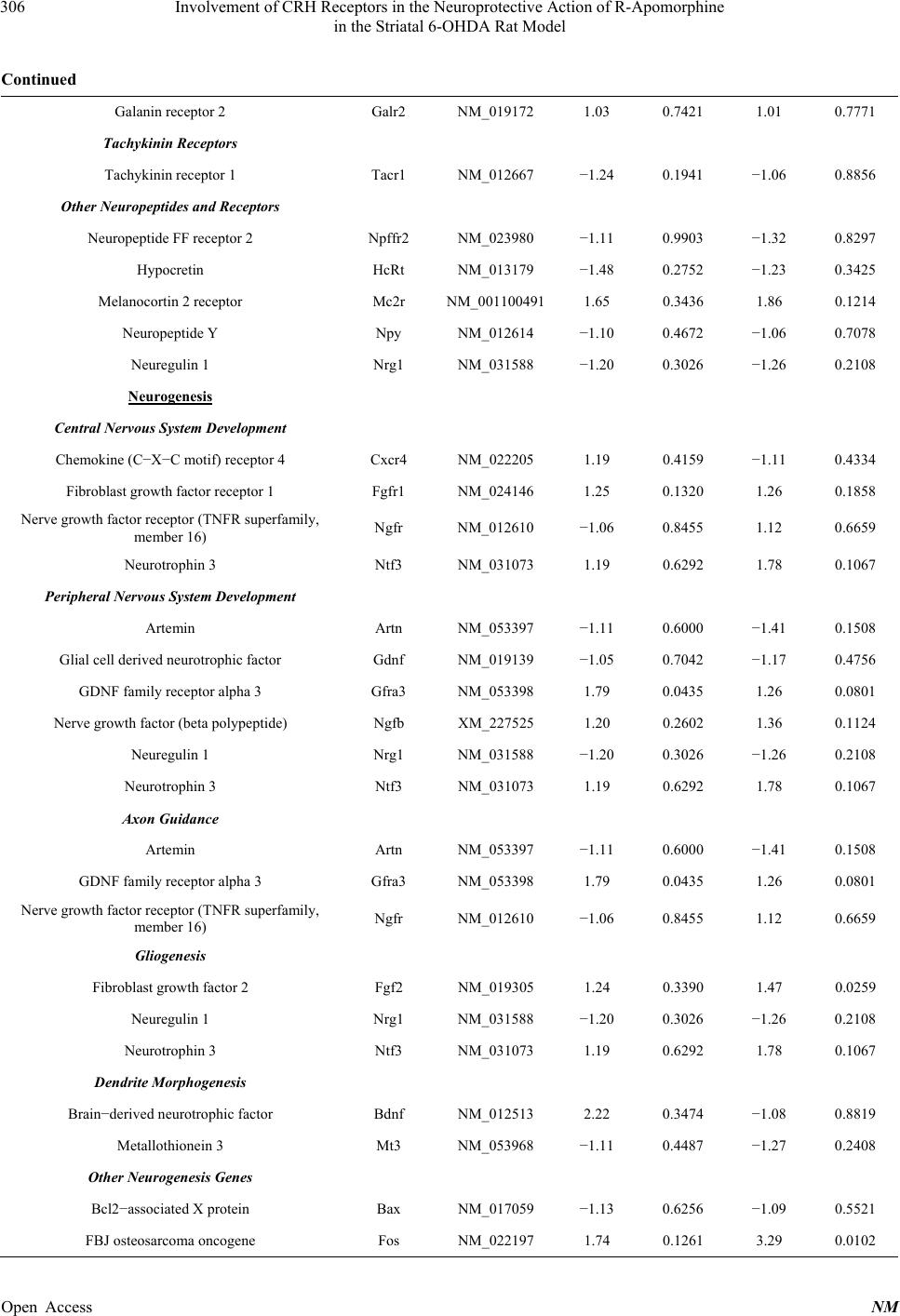 Involvement of CRH Receptors in the Neuroprotective Action of R-Apomorphine in the Striatal 6-OHDA Rat Model 306 Continued Galanin receptor 2 Galr2 NM_019172 1.03 0.7421 1.01 0.7771 Tachykinin Receptors Tachykinin receptor 1 Tacr1 NM_012667 −1.24 0.1941 −1.06 0.8856 Other Neuropeptides and Receptors Neuropeptide FF receptor 2 Npffr2 NM_023980 −1.11 0.9903 −1.32 0.8297 Hypocretin HcRt NM_013179 −1.48 0.2752 −1.23 0.3425 Melanocortin 2 receptor Mc2r NM_0011004911.65 0.3436 1.86 0.1214 Neuropeptide Y Npy NM_012614 −1.10 0.4672 −1.06 0.7078 Neuregulin 1 Nrg1 NM_031588 −1.20 0.3026 −1.26 0.2108 Neurogenesis Central Nervous System Development Chemokine (C−X−C motif) receptor 4 Cxcr4 NM_022205 1.19 0.4159 −1.11 0.4334 Fibroblast growth factor receptor 1 Fgfr1 NM_024146 1.25 0.1320 1.26 0.1858 Nerve growth factor receptor (TNFR superfamily, member 16) Ngfr NM_012610 −1.06 0.8455 1.12 0.6659 Neurotrophin 3 Ntf3 NM_031073 1.19 0.6292 1.78 0.1067 Peripheral Nervous System Development Artemin Artn NM_053397 −1.11 0.6000 −1.41 0.1508 Glial cell derived neurotrophic factor Gdnf NM_019139 −1.05 0.7042 −1.17 0.4756 GDNF family receptor alpha 3 Gfra3 NM_053398 1.79 0.0435 1.26 0.0801 Nerve growth factor (beta polypeptide) Ngfb XM_227525 1.20 0.2602 1.36 0.1124 Neuregulin 1 Nrg1 NM_031588 −1.20 0.3026 −1.26 0.2108 Neurotrophin 3 Ntf3 NM_031073 1.19 0.6292 1.78 0.1067 Axon Guidance Artemin Artn NM_053397 −1.11 0.6000 −1.41 0.1508 GDNF family receptor alpha 3 Gfra3 NM_053398 1.79 0.0435 1.26 0.0801 Nerve growth factor receptor (TNFR superfamily, member 16) Ngfr NM_012610 −1.06 0.8455 1.12 0.6659 Gliogenesis Fibroblast growth factor 2 Fgf2 NM_019305 1.24 0.3390 1.47 0.0259 Neuregulin 1 Nrg1 NM_031588 −1.20 0.3026 −1.26 0.2108 Neurotrophin 3 Ntf3 NM_031073 1.19 0.6292 1.78 0.1067 Dendrite Morphogenesis Brain−derived neurotrophic factor Bdnf NM_012513 2.22 0.3474 −1.08 0.8819 Metallothionein 3 Mt3 NM_053968 −1.11 0.4487 −1.27 0.2408 Other Neurogenesis Genes Bcl2−associated X protein Bax NM_017059 −1.13 0.6256 −1.09 0.5521 FBJ osteosarcoma oncogene Fos NM_022197 1.74 0.1261 3.29 0.0102 Open Access NM
 Involvement of CRH Receptors in the Neuroprotective Action of R-Apomorphine in the Striatal 6-OHDA Rat Model 307 Continued Galanin receptor 2 Galr2 NM_019172 1.03 0.7421 1.01 0.7771 GDNF family receptor alpha 1 Gfra1 NM_012959 1.50 0.0999 1.40 0.1511 GDNF family receptor alpha 2 Gfra2 NM_012750 −1.00 0.9577 −1.14 0.6205 NEL−like 1 (chicken) Nell1 NM_031069 −1.08 0.5581 1.01 0.9296 Neurotrophin 5 Ntf5 NM_013184 −1.25 0.3904 −1.96 0.4401 Neurotrophic tyrosine kinase, receptor, type 1 Ntrk1 NM_021589 −1.82 0.0828 −1.42 0.3921 Neurotrophic tyrosine kinase, receptor, type 2 Ntrk2 NM_012731 −1.17 0.3840 −1.44 0.1327 Synaptic Transmission Cerebellin 1 precursor Cbln1 NM_001109127−1.38 0.9382 −1.64 0.6224 Growth and Differentiation Growth Factors and Receptors Artemin Artn NM_053397 −1.11 0.6000 −1.41 0.1508 Brain−derived neurotrophic factor Bdnf NM_012513 2.22 0.3474 −1.08 0.8819 Fibroblast growth factor 2 Fgf2 NM_019305 1.24 0.3390 1.47 0.0259 Fibroblast growth factor 9 Fgf9 NM_012952 1.24 0.4508 1.17 0.5779 Fibroblast growth factor receptor 1 Fgfr1 NM_024146 1.25 0.1320 1.26 0.1858 Glial cell derived neurotrophic factor Gdnf NM_019139 −1.05 0.7042 −1.17 0.4756 Glia maturation factor, beta Gmfb NM_031032 1.10 0.4245 1.26 0.0867 Glia maturation factor, gamma Gmfg NM_181091 1.70 0.0072 1.83 0.0032 Interleukin 10 Il10 NM_012854 1.35 0.4768 −1.02 0.7271 Interleukin 1 beta Il1b NM_031512 2.50 0.1685 3.16 0.0035 Interleukin 6 Il6 NM_012589 −1.21 0.3849 1.46 0.6407 Leukemia inhibitory factor Lif NM_022196 1.57 0.3027 2.17 0.0687 Metallothionein 3 Mt3 NM_053968 −1.11 0.4487 −1.27 0.2408 Nerve growth factor (beta polypeptide) Ngfb XM_227525 1.20 0.2602 1.36 0.1124 Neuregulin 2 Nrg2 XM_344662 −1.01 0.9586 1.20 0.3032 Neurotrophin 3 Ntf3 NM_031073 1.19 0.6292 1.78 0.1067 Neurotrophin 5 Ntf5 NM_013184 −1.25 0.3904 −1.96 0.4401 Persephin Pspn NM_013014 −1.03 0.7075 1.04 0.7371 Transforming growth factor alpha Tgfa NM_012671 1.23 0.1619 1.22 0.1567 Transforming growth factor, beta 1 Tgfb1 NM_021578 2.90 0.0029 3.36 0.0001 Transforming growth factor beta 1 induced transcript 1 Tgfb1i1 XM_341934 1.21 0.2067 1.20 0.3595 Tumor protein p53 Tp53 NM_030989 −1.10 0.5051 1.10 0.6728 VGF nerve growth factor inducible Vgf NM_030997 −1.36 0.3562 −1.42 0.1827 Cell cycle Fibroblast growth factor 2 Fgf2 NM_019305 1.24 0.3390 1.47 0.0259 Fibroblast growth factor 9 Fgf9 NM_012952 1.24 0.4508 1.17 0.5779 Open Access NM
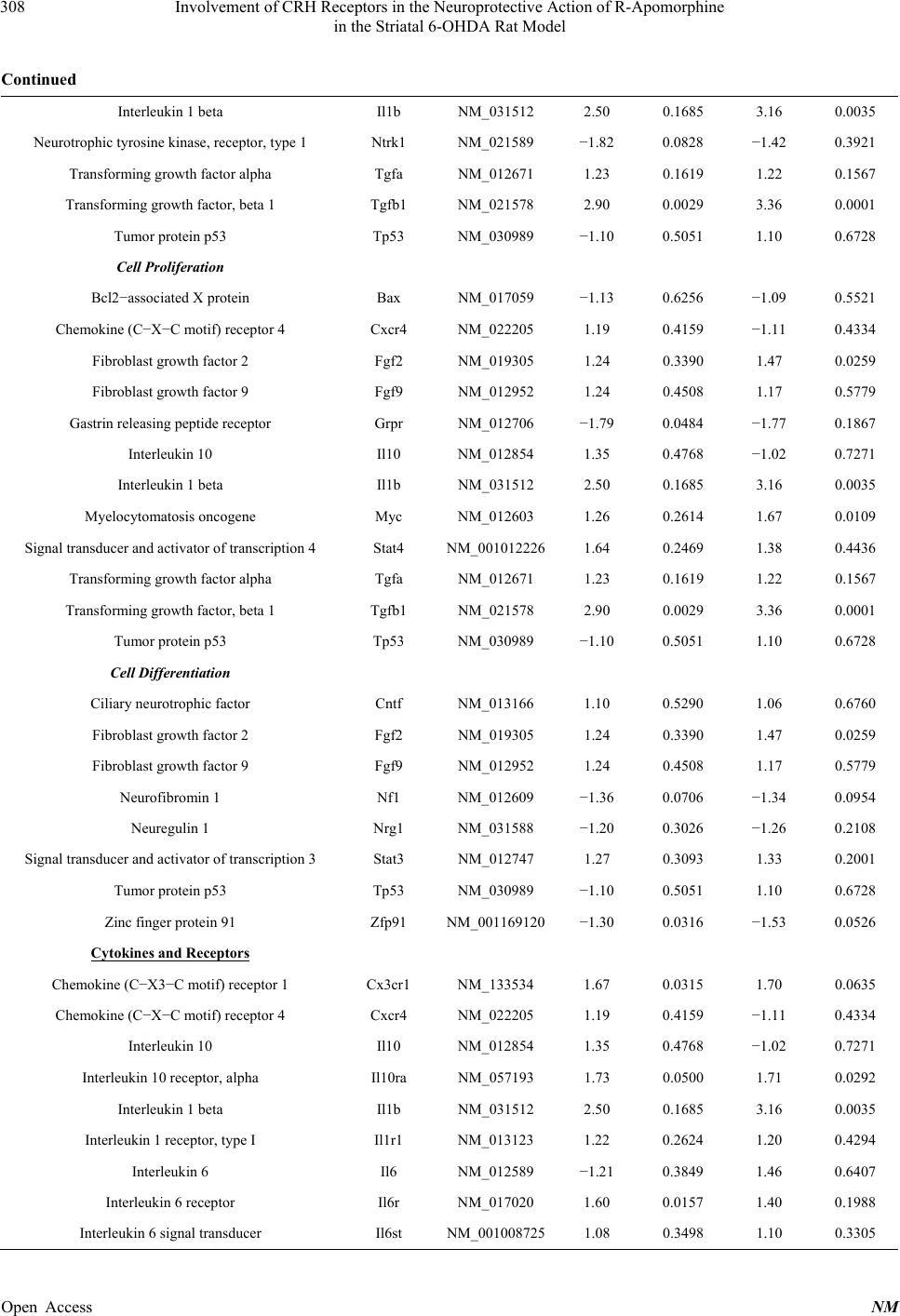 Involvement of CRH Receptors in the Neuroprotective Action of R-Apomorphine in the Striatal 6-OHDA Rat Model 308 Continued Interleukin 1 beta Il1b NM_031512 2.50 0.1685 3.16 0.0035 Neurotrophic tyrosine kinase, receptor, type 1 Ntrk1 NM_021589 −1.82 0.0828 −1.42 0.3921 Transforming growth factor alpha Tgfa NM_012671 1.23 0.1619 1.22 0.1567 Transforming growth factor, beta 1 Tgfb1 NM_021578 2.90 0.0029 3.36 0.0001 Tumor protein p53 Tp53 NM_030989 −1.10 0.5051 1.10 0.6728 Cell Proliferation Bcl2−associated X protein Bax NM_017059 −1.13 0.6256 −1.09 0.5521 Chemokine (C−X−C motif) receptor 4 Cxcr4 NM_022205 1.19 0.4159 −1.11 0.4334 Fibroblast growth factor 2 Fgf2 NM_019305 1.24 0.3390 1.47 0.0259 Fibroblast growth factor 9 Fgf9 NM_012952 1.24 0.4508 1.17 0.5779 Gastrin releasing peptide receptor Grpr NM_012706 −1.79 0.0484 −1.77 0.1867 Interleukin 10 Il10 NM_012854 1.35 0.4768 −1.02 0.7271 Interleukin 1 beta Il1b NM_031512 2.50 0.1685 3.16 0.0035 Myelocytomatosis oncogene Myc NM_012603 1.26 0.2614 1.67 0.0109 Signal transducer and activator of transcription 4 Stat4 NM_0010122261.64 0.2469 1.38 0.4436 Transforming growth factor alpha Tgfa NM_012671 1.23 0.1619 1.22 0.1567 Transforming growth factor, beta 1 Tgfb1 NM_021578 2.90 0.0029 3.36 0.0001 Tumor protein p53 Tp53 NM_030989 −1.10 0.5051 1.10 0.6728 Cell Differentiation Ciliary neurotrophic factor Cntf NM_013166 1.10 0.5290 1.06 0.6760 Fibroblast growth factor 2 Fgf2 NM_019305 1.24 0.3390 1.47 0.0259 Fibroblast growth factor 9 Fgf9 NM_012952 1.24 0.4508 1.17 0.5779 Neurofibromin 1 Nf1 NM_012609 −1.36 0.0706 −1.34 0.0954 Neuregulin 1 Nrg1 NM_031588 −1.20 0.3026 −1.26 0.2108 Signal transducer and activator of transcription 3 Stat3 NM_012747 1.27 0.3093 1.33 0.2001 Tumor protein p53 Tp53 NM_030989 −1.10 0.5051 1.10 0.6728 Zinc finger protein 91 Zfp91 NM_001169120−1.30 0.0316 −1.53 0.0526 Cytokines and Receptors Chemokine (C−X3−C motif) receptor 1 Cx3cr1 NM_133534 1.67 0.0315 1.70 0.0635 Chemokine (C−X−C motif) receptor 4 Cxcr4 NM_022205 1.19 0.4159 −1.11 0.4334 Interleukin 10 Il10 NM_012854 1.35 0.4768 −1.02 0.7271 Interleukin 10 receptor, alpha Il10ra NM_057193 1.73 0.0500 1.71 0.0292 Interleukin 1 beta Il1b NM_031512 2.50 0.1685 3.16 0.0035 Interleukin 1 receptor, type I Il1r1 NM_013123 1.22 0.2624 1.20 0.4294 Interleukin 6 Il6 NM_012589 −1.21 0.3849 1.46 0.6407 Interleukin 6 receptor Il6r NM_017020 1.60 0.0157 1.40 0.1988 Interleukin 6 signal transducer Il6st NM_0010087251.08 0.3498 1.10 0.3305 Open Access NM
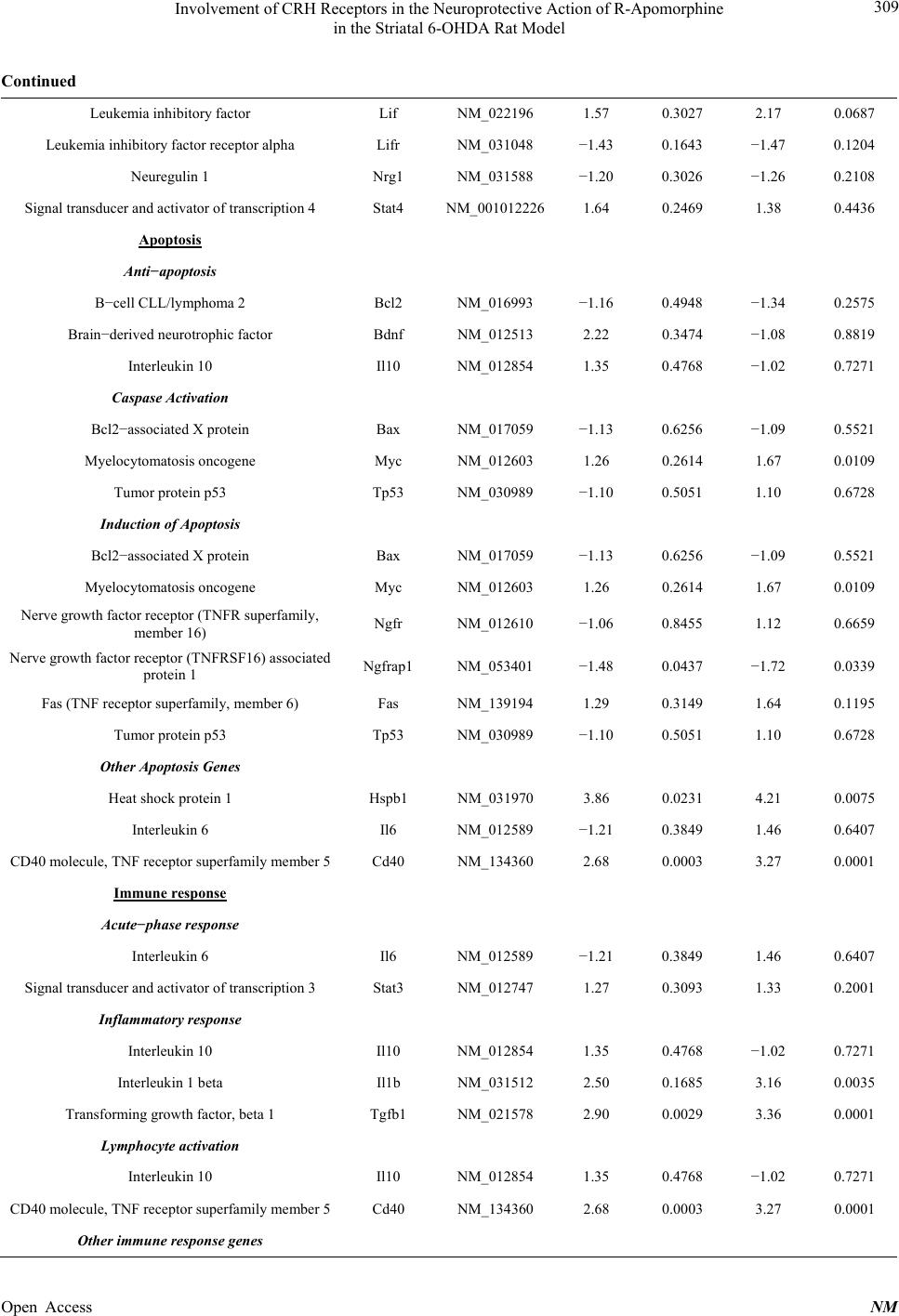 Involvement of CRH Receptors in the Neuroprotective Action of R-Apomorphine in the Striatal 6-OHDA Rat Model 309 Continued Leukemia inhibitory factor Lif NM_022196 1.57 0.3027 2.17 0.0687 Leukemia inhibitory factor receptor alpha Lifr NM_031048 −1.43 0.1643 −1.47 0.1204 Neuregulin 1 Nrg1 NM_031588 −1.20 0.3026 −1.26 0.2108 Signal transducer and activator of transcription 4 Stat4 NM_0010122261.64 0.2469 1.38 0.4436 Apoptosis Anti−apoptosis B−cell CLL/lymphoma 2 Bcl2 NM_016993 −1.16 0.4948 −1.34 0.2575 Brain−derived neurotrophic factor Bdnf NM_012513 2.22 0.3474 −1.08 0.8819 Interleukin 10 Il10 NM_012854 1.35 0.4768 −1.02 0.7271 Caspase Activation Bcl2−associated X protein Bax NM_017059 −1.13 0.6256 −1.09 0.5521 Myelocytomatosis oncogene Myc NM_012603 1.26 0.2614 1.67 0.0109 Tumor protein p53 Tp53 NM_030989 −1.10 0.5051 1.10 0.6728 Induction of Apoptosis Bcl2−associated X protein Bax NM_017059 −1.13 0.6256 −1.09 0.5521 Myelocytomatosis oncogene Myc NM_012603 1.26 0.2614 1.67 0.0109 Nerve growth factor receptor (TNFR superfamily, member 16) Ngfr NM_012610 −1.06 0.8455 1.12 0.6659 Nerve growth factor receptor (TNFRSF16) associated protein 1 Ngfrap1 NM_053401 −1.48 0.0437 −1.72 0.0339 Fas (TNF receptor superfamily, member 6) Fas NM_139194 1.29 0.3149 1.64 0.1195 Tumor protein p53 Tp53 NM_030989 −1.10 0.5051 1.10 0.6728 Other Apoptosis Genes Heat shock protein 1 Hspb1 NM_031970 3.86 0.0231 4.21 0.0075 Interleukin 6 Il6 NM_012589 −1.21 0.3849 1.46 0.6407 CD40 molecule, TNF receptor superfamily member 5 Cd40 NM_134360 2.68 0.0003 3.27 0.0001 Immune response Acute−phase response Interleukin 6 Il6 NM_012589 −1.21 0.3849 1.46 0.6407 Signal transducer and activator of transcription 3 Stat3 NM_012747 1.27 0.3093 1.33 0.2001 Inflammatory response Interleukin 10 Il10 NM_012854 1.35 0.4768 −1.02 0.7271 Interleukin 1 beta Il1b NM_031512 2.50 0.1685 3.16 0.0035 Transforming growth factor, beta 1 Tgfb1 NM_021578 2.90 0.0029 3.36 0.0001 Lymphocyte activation Interleukin 10 Il10 NM_012854 1.35 0.4768 −1.02 0.7271 CD40 molecule, TNF receptor superfamily member 5 Cd40 NM_134360 2.68 0.0003 3.27 0.0001 Other immune response genes Open Access NM
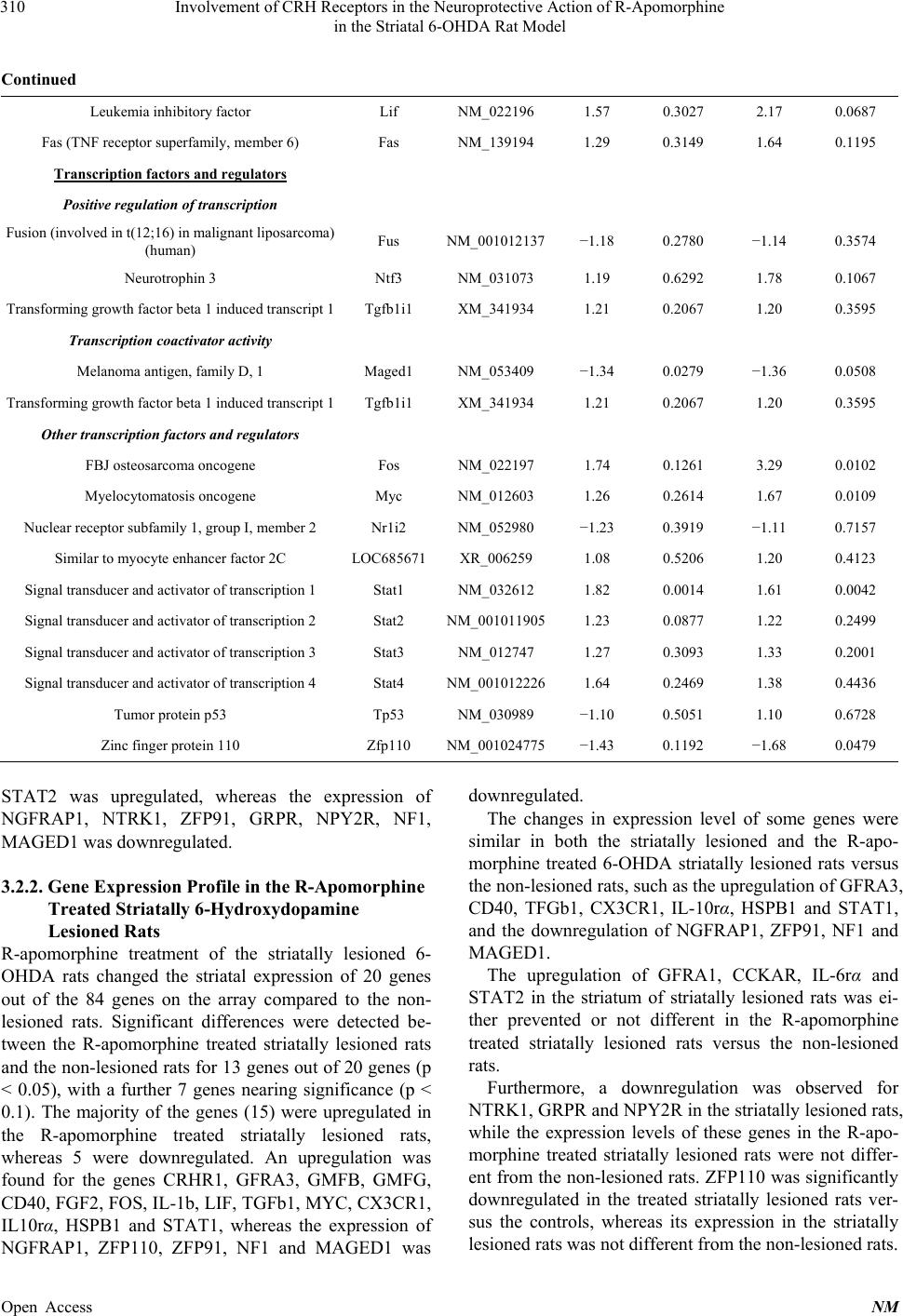 Involvement of CRH Receptors in the Neuroprotective Action of R-Apomorphine in the Striatal 6-OHDA Rat Model Open Access NM 310 Continued Leukemia inhibitory factor Lif NM_022196 1.57 0.3027 2.17 0.0687 Fas (TNF receptor superfamily, member 6) Fas NM_139194 1.29 0.3149 1.64 0.1195 Transcription factors and regulators Positive regulation of transcription Fusion (involved in t(12;16) in malignant liposarcoma) (human) Fus NM_001012137−1.18 0.2780 −1.14 0.3574 Neurotrophin 3 Ntf3 NM_031073 1.19 0.6292 1.78 0.1067 Transforming growth factor beta 1 induced transcript 1 Tgfb1i1 XM_341934 1.21 0.2067 1.20 0.3595 Transcription coactivator activity Melanoma antigen, family D, 1 Maged1 NM_053409 −1.34 0.0279 −1.36 0.0508 Transforming growth factor beta 1 induced transcript 1 Tgfb1i1 XM_341934 1.21 0.2067 1.20 0.3595 Other transcription factors and regulators FBJ osteosarcoma oncogene Fos NM_022197 1.74 0.1261 3.29 0.0102 Myelocytomatosis oncogene Myc NM_012603 1.26 0.2614 1.67 0.0109 Nuclear receptor subfamily 1, group I, member 2 Nr1i2 NM_052980 −1.23 0.3919 −1.11 0.7157 Similar to myocyte enhancer factor 2C LOC685671XR_006259 1.08 0.5206 1.20 0.4123 Signal transducer and activator of transcription 1 Stat1 NM_032612 1.82 0.0014 1.61 0.0042 Signal transducer and activator of transcription 2 Stat2 NM_0010119051.23 0.0877 1.22 0.2499 Signal transducer and activator of transcription 3 Stat3 NM_012747 1.27 0.3093 1.33 0.2001 Signal transducer and activator of transcription 4 Stat4 NM_0010122261.64 0.2469 1.38 0.4436 Tumor protein p53 Tp53 NM_030989 −1.10 0.5051 1.10 0.6728 Zinc finger protein 110 Zfp110 NM_001024775−1.43 0.1192 −1.68 0.0479 STAT2 was upregulated, whereas the expression of NGFRAP1, NTRK1, ZFP91, GRPR, NPY2R, NF1, MAGED1 was downregulated. 3.2.2. Gene Expression Profile in the R-Apomorphine Treated Striatally 6-Hydroxydopamine Lesioned Rats R-apomorphine treatment of the striatally lesioned 6- OHDA rats changed the striatal expression of 20 genes out of the 84 genes on the array compared to the non- lesioned rats. Significant differences were detected be- tween the R-apomorphine treated striatally lesioned rats and the non-lesioned rats for 13 genes out of 20 genes (p < 0.05), with a further 7 genes nearing significance (p < 0.1). The majority of the genes (15) were upregulated in the R-apomorphine treated striatally lesioned rats, whereas 5 were downregulated. An upregulation was found for the genes CRHR1, GFRA3, GMFB, GMFG, CD40, FGF2, FOS, IL-1b, LIF, TGFb1, MYC, CX3CR1, IL10rα, HSPB1 and STAT1, whereas the expression of NGFRAP1, ZFP110, ZFP91, NF1 and MAGED1 was downregulated. The changes in expression level of some genes were similar in both the striatally lesioned and the R-apo- morphine treated 6-OHDA striatally lesioned rats versus the non-lesioned rats, such as the upregulation of GFRA3, CD40, TFGb1, CX3CR1, IL-10rα, HSPB1 and STAT1, and the downregulation of NGFRAP1, ZFP91, NF1 and MAGED1. The upregulation of GFRA1, CCKAR, IL-6rα and STAT2 in the striatum of striatally lesioned rats was ei- ther prevented or not different in the R-apomorphine treated striatally lesioned rats versus the non-lesioned rats. Furthermore, a downregulation was observed for NTRK1, GRPR and NPY2R in the striatally lesioned rats, while the expression levels of these genes in the R-apo- morphine treated striatally lesioned rats were not differ- ent from the non-lesioned rats. ZFP110 was significantly downregulated in the treated striatally lesioned rats ver- sus the controls, whereas its expression in the striatally lesioned rats was not different from the non-lesioned rats.
 Involvement of CRH Receptors in the Neuroprotective Action of R-Apomorphine in the Striatal 6-OHDA Rat Model 311 Based on the global overview of the gene expression alterations, we chose to focus on the neuropeptide recap- tors CRHR1, CRHR2, NPY1R and NPY2R. These 4 receptors are all G protein coupled receptors (GPCRs) and have been implicated as potential drug targets in the treatment of Parkinson’s disease, and are more exten- sively described in the discussion section. Regarding these neuropeptide receptors the following findings were observed. The expression level of CRHR1 was not sig- nificantly different between non-lesioned and striatally lesioned rats. However, in the R-apomorphine treated striatally lesioned rats an upregulation of the expression of CRHR1 (p = 0.06) was observed when compared to non-lesioned rats. Although this upregulation was near- ing significance, there was no difference when compared to striatally lesioned rats (Figure 4). In the same way, a significant upregulation was found for CRHR2 in the striatally lesioned rats versus the non- lesioned and the treated striatally lesioned rats, whereas no significant difference was found between the R-apo- morphine treated striatally lesioned rats and the non- lesioned rats, suggesting that the treatment normalized or prevented the upregulation of CRHR2 (Figure 4). Regarding the NPY1R, there was a downregulation in the striatally lesioned rats versus the non-lesioned rats, but this was not statistically significant. However, a sig- nificant downregulation was observed in the striatally lesioned rats compared to the R-apomorphine treated striatally lesioned rats. There was no significant differ- ence between non-lesioned and R-apomorphine treated striatally lesioned rats, suggesting that R-apomorphine normalized or prevented changes in the expression level of NPY1R (Figure 5). NPY2R was significantly downregulated in the stri- atally lesioned rats when compared to the non-lesioned rats. This downregulation was either prevented or nor- malized by R-apomorphine, as no significant differences were observed between the R-apomorphine treated stri- atally lesioned and non-lesioned rats (Figure 5). 3.3. Protein Expression of CRHR1, CRHR2, NPY1R and NPY2R CRHR1 and CRHR2 protein levels, quantified with ELISA, confirmed the results of the gene expression analysis. The CRHR1 protein content in the denervated Figure 4. CRHR1 and CRHR2 mRNA and protein expression. CRHR1 (upper left panel) and CRHR2 (lower left panel) mRNA expression in the left striatum of control (n = 3), saline treated 6-OHDA striatal lesioned (n = 4) and R-apomorphine treated 6-OHDA striatally lesioned rats (n = 4) was determined using quantitative real-time PCR as described in the Methods. A fold-change greater than 1 is reported as up regulation, while lower than 1 is reported as down regulation. The protein expression of CRHR1 (upper right panel) and CRHR2 (lower right panel) in the left striatum in all the groups [(control (n = 6), saline treated 6-OHDA striatal lesioned (n = 6) and R-apomorphine treated 6-OHDA striatally lesioned rats (n = 6)] was determined using ELISA as described in the Methods. For each protein, the average control was set to 100%. Data are ex- pressed as mean ± S.E.M. *Significantly different (one-way ANOVA followed by a Bonferroni’s Multiple Comparison Test: p < 0.05). Open Access NM
 Involvement of CRH Receptors in the Neuroprotective Action of R-Apomorphine in the Striatal 6-OHDA Rat Model 312 Figure 5. NPY1R and NPY2R mRNA expression. NPYR1 (left panel) and NPYR2 (right panel) mRNA expression in the left striatum of control (n = 3), saline treated 6-OHDA striatal lesioned (n = 4) and R-apomorphine treated 6-OHDA striatally lesioned rats (n = 4) was determined using quantitative real-time PCR as described in the Methods. A fold-change greater than 1 is reported as up regulation, while lower than 1 is reported as down regulation. Data are expressed as mean ± S.E.M. *Significantly different (one-way ANOVA followed by a Bonferroni’s Multiple Comparison Test: p < 0.05). striata of the R-apomorphine treated striatally lesioned rats was significantly higher when compared to the left striata of the non-lesioned and the denervated striata of the striatally lesioned rats, while the CRHR1 protein content in the striata of non-lesioned rats and the dener- vated striata of striatally lesioned rats was not signifi- cantly different (Figure 4). CRHR2 protein levels of the denervated striata of the striatally lesioned rats were sig- nificantly higher when compared to the left striata of non-lesioned rats and the denervated striata of the R- apomorphine treated striatally lesioned rats, with no sta- tistical difference between the R-apomorphine group and the control group (Figure 4). No significant changes in NPY1R and NPY2R protein levels were detected in the left striata between the three groups (data not shown), suggesting that these receptors might not be involved in the biological action of R- apomorphine. However, this method does not monitor post-transcriptional and post-translational modifications, and it provides only a partial picture of the biological events [35]. 4. Discussion Our data show that R-apomorphine treatment (10 mg/kg/ day, s.c., during 11 days) of rats striatally lesioned with 6-OHDA is associated with a partial restoration of the DA levels and the DOPAC:DA ratios in the ipsilateral striatum. Furthermore, in the open field test an improve- ment in distance moved and attenuation of the relative meander was observed. The decreased locomotor activity, such as in the lesioned rats, has been attributed to the loss of DA neurotransmission [36]. The reason that not all the investigated behavioural parameters assessed during the open field test normalised or improved, might be due to the fact that R-apomorphine does not fully restore the neuronal degeneration caused by 6-OHDA, which is in line with the observed partial neuroprotection. These data confirm our previous findings where the same treatment with R-apomorphine significantly reduced the ampheta- mine-induced rotations, attenuated the DA levels and the DOPAC:DA ratios, and subsequently suggest that the functionality and the integrity of the nigrostriatal dopa- minergic system have at least partially been preserved by a treatment with R-apomorphine [14]. The gene expression analysis demonstrated that dif- ferent genes are altered in the lesioned striatum of the saline treated striatally lesioned rats and the R-apomor- phine treated striatally lesioned rats. Genes that were altered in the saline treated striatally lesioned rats, are related to the regulation of neurotrophins, neuropeptides, immune response, neurogenesis, growth and differentia- tion, cell cycle, cell proliferation, apoptosis and tran- scription factors and regulators, suggesting that different major events occur which might be involved in the mo- lecular pathways of the pathogenesis of Parkinson’s dis- ease and confirming the complexity of the disorder. Treatment with R-apomorphine of the striatally lesioned rats did not reverse all the gene alterations that were ob- served in the saline treated striatally lesioned rats. A role for inflammation in the striatal 6-OHDA rat model can be suggested from our data as several inflam- matory-related genes were upregulated, such as CX3CR1, IL6R, IL10RA, TGFB1, and CD40. The concept of neuroinflammation as a primary response to 6-OHDA in both the striatum and the substantia nigra has been con- firmed by Na et al., 2010 [37]. Furthermore, the DA- ergic neuronal loss within the striatal 6-OHDA rat model is preceded by microglial activation [38,39], suggesting a temporal relationship between neurodegeneration and neuroinflammation [39]. Similarly, in vivo and postmor- tem observations from Parkinson’s disease patients show that indeed activated microglia are present and that the Open Access NM
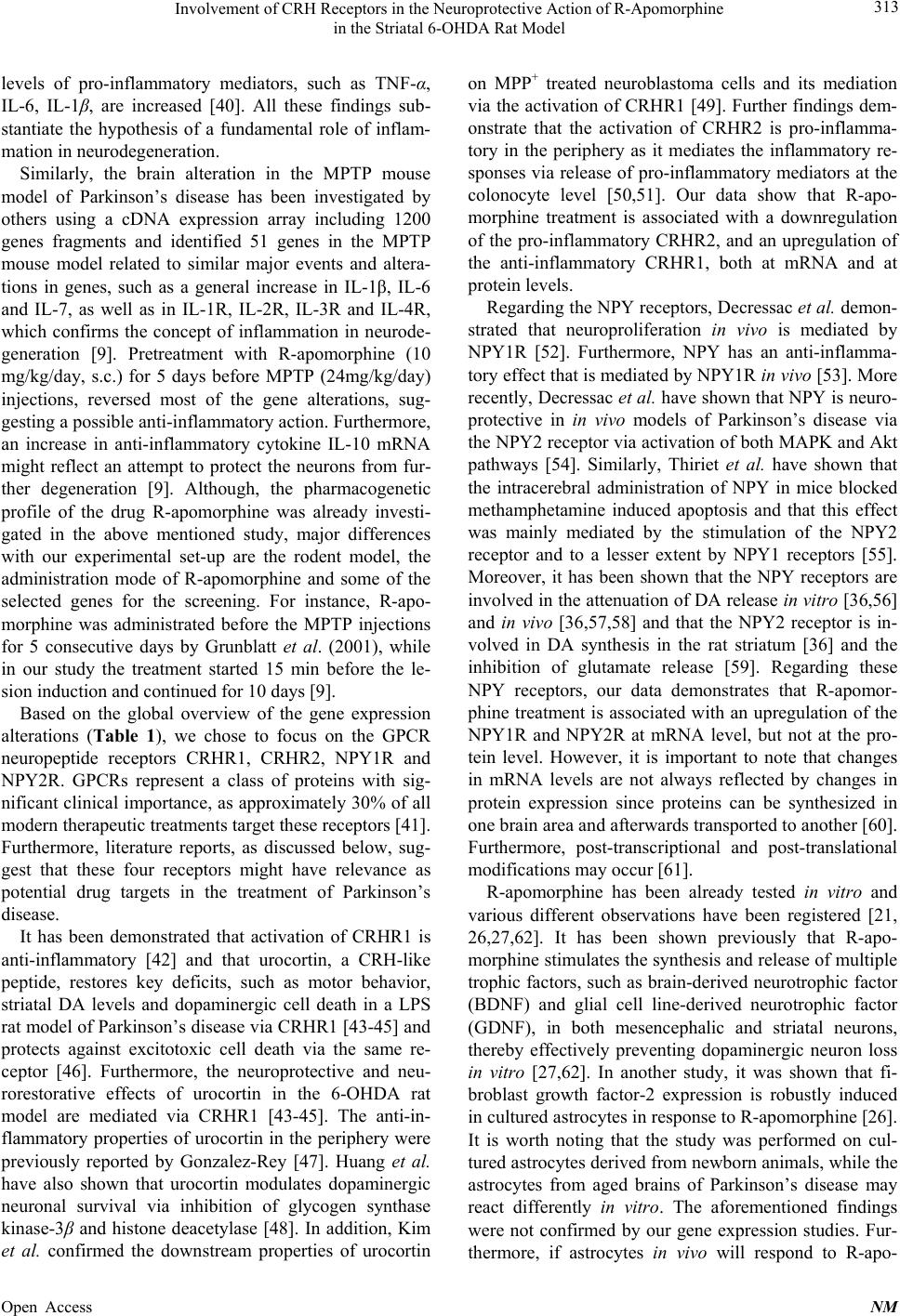 Involvement of CRH Receptors in the Neuroprotective Action of R-Apomorphine in the Striatal 6-OHDA Rat Model 313 levels of pro-inflammatory mediators, such as TNF-α, IL-6, IL-1β, are increased [40]. All these findings sub- stantiate the hypothesis of a fundamental role of inflam- mation in neurodegeneration. Similarly, the brain alteration in the MPTP mouse model of Parkinson’s disease has been investigated by others using a cDNA expression array including 1200 genes fragments and identified 51 genes in the MPTP mouse model related to similar major events and altera- tions in genes, such as a general increase in IL-1β, IL-6 and IL-7, as well as in IL-1R, IL-2R, IL-3R and IL-4R, which confirms the concept of inflammation in neurode- generation [9]. Pretreatment with R-apomorphine (10 mg/kg/day, s.c.) for 5 days before MPTP (24mg/kg/day) injections, reversed most of the gene alterations, sug- gesting a possible anti-inflammatory action. Furthermore, an increase in anti-inflammatory cytokine IL-10 mRNA might reflect an attempt to protect the neurons from fur- ther degeneration [9]. Although, the pharmacogenetic profile of the drug R-apomorphine was already investi- gated in the above mentioned study, major differences with our experimental set-up are the rodent model, the administration mode of R-apomorphine and some of the selected genes for the screening. For instance, R-apo- morphine was administrated before the MPTP injections for 5 consecutive days by Grunblatt et al. (2001), while in our study the treatment started 15 min before the le- sion induction and continued for 10 days [9]. Based on the global overview of the gene expression alterations (Table 1), we chose to focus on the GPCR neuropeptide receptors CRHR1, CRHR2, NPY1R and NPY2R. GPCRs represent a class of proteins with sig- nificant clinical importance, as approximately 30% of all modern therapeutic treatments target these receptors [41]. Furthermore, literature reports, as discussed below, sug- gest that these four receptors might have relevance as potential drug targets in the treatment of Parkinson’s disease. It has been demonstrated that activation of CRHR1 is anti-inflammatory [42] and that urocortin, a CRH-like peptide, restores key deficits, such as motor behavior, striatal DA levels and dopaminergic cell death in a LPS rat model of Parkinson’s disease via CRHR1 [43-45] and protects against excitotoxic cell death via the same re- ceptor [46]. Furthermore, the neuroprotective and neu- rorestorative effects of urocortin in the 6-OHDA rat model are mediated via CRHR1 [43-45]. The anti-in- flammatory properties of urocortin in the periphery were previously reported by Gonzalez-Rey [47]. Huang et al. have also shown that urocortin modulates dopaminergic neuronal survival via inhibition of glycogen synthase kinase-3β and histone deacetylase [48]. In addition, Kim et al. confirmed the downstream properties of urocortin on MPP+ treated neuroblastoma cells and its mediation via the activation of CRHR1 [49]. Further findings dem- onstrate that the activation of CRHR2 is pro-inflamma- tory in the periphery as it mediates the inflammatory re- sponses via release of pro-inflammatory mediators at the colonocyte level [50,51]. Our data show that R-apo- morphine treatment is associated with a downregulation of the pro-inflammatory CRHR2, and an upregulation of the anti-inflammatory CRHR1, both at mRNA and at protein levels. Regarding the NPY receptors, Decressac et al. demon- strated that neuroproliferation in vivo is mediated by NPY1R [52]. Furthermore, NPY has an anti-inflamma- tory effect that is mediated by NPY1R in vivo [53]. More recently, Decressac et al. have shown that NPY is neuro- protective in in vivo models of Parkinson’s disease via the NPY2 receptor via activation of both MAPK and Akt pathways [54]. Similarly, Thiriet et al. have shown that the intracerebral administration of NPY in mice blocked methamphetamine induced apoptosis and that this effect was mainly mediated by the stimulation of the NPY2 receptor and to a lesser extent by NPY1 receptors [55]. Moreover, it has been shown that the NPY receptors are involved in the attenuation of DA release in vitro [36,56] and in vivo [36,57,58] and that the NPY2 receptor is in- volved in DA synthesis in the rat striatum [36] and the inhibition of glutamate release [59]. Regarding these NPY receptors, our data demonstrates that R-apomor- phine treatment is associated with an upregulation of the NPY1R and NPY2R at mRNA level, but not at the pro- tein level. However, it is important to note that changes in mRNA levels are not always reflected by changes in protein expression since proteins can be synthesized in one brain area and afterwards transported to another [60]. Furthermore, post-transcriptional and post-translational modifications may occur [61]. R-apomorphine has been already tested in vitro and various different observations have been registered [21, 26,27,62]. It has been shown previously that R-apo- morphine stimulates the synthesis and release of multiple trophic factors, such as brain-derived neurotrophic factor (BDNF) and glial cell line-derived neurotrophic factor (GDNF), in both mesencephalic and striatal neurons, thereby effectively preventing dopaminergic neuron loss in vitro [27,62]. In another study, it was shown that fi- broblast growth factor-2 expression is robustly induced in cultured astrocytes in response to R-apomorphine [26]. It is worth noting that the study was performed on cul- tured astrocytes derived from newborn animals, while the astrocytes from aged brains of Parkinson’s disease may react differently in vitro. The aforementioned findings were not confirmed by our gene expression studies. Fur- thermore, if astrocytes in vivo will respond to R-apo- Open Access NM
 Involvement of CRH Receptors in the Neuroprotective Action of R-Apomorphine in the Striatal 6-OHDA Rat Model 314 morphine treatment in the same way as reported in the aforementioned study needs to be investigated, as in vitro and in vivo findings do not always correlate. The absence of sham lesioned rats within our experi- mental design may be considered by others as a study limitation. However, various publications indicate that sham lesioning of rodents has no effect on the main pathological hallmarks of Parkinson’s disease, such as DA loss [30,37,43,63,64]. Furthermore, Na et al. [37] assessed the number of gene expression changes in vehi- cle and 6-OHDA lesioned striatum and substantia nigra at various time points by using cDNA microarray. They found that three days after vehicle administration there are profound changes in relative striatal gene expression levels (<50 genes). However, this gene expression pat- tern began to wane as a function of time, and fourteen days after vehicle administration, the number of altered genes was almost equal to zero. A similar pattern was observed for 6-OHDA striatally lesioned rats. However, within this group the number of altered genes was much higher after 3 days (>150 genes) and after 14 days (>50 genes) compared to the vehicle lesioned rats. These findings suggest that sham lesioning has an effect on mRNA expression, but that it disappears very quickly as a function of time [37]. In addition, needle insertion and vehicle injection does not alter functional protein levels of BDNF and CNTF [63,65]. However, some astrocytic reaction in the neostriatum of solvent injected rats close to the needle track has been observed, while in the neu- rotoxin lesioned animals this reaction was much more widespread [66-68]. Our own findings also show that neurodegeneration caused by a unilateral injection of 6-OHDA in the medial forebrain bundle or the striatum, has no effect on the striatal protein expression of GDNF at different time points after lesion in the intact and the denervated striata of the lesioned animals and that of control animals [60]. The aforementioned findings sug- gest that the insertion of the needle for lesioning does not cause significant alterations in terms of striatal neuro- transmitters and behaviour. However, some acute, minor and temporary effects have been observed at the gene and protein level, but this is limited to the needle tract. In our study, we assessed the gene and protein expression fourteen days after surgery. Even though, there would be an effect of the needle insertion, the combination with the neurotoxin is much more invasive and destructive. Similarly, we did not include intact rats which have been treated with R-apomorphine, as we wanted to in- vestigate the effects of R-apomorphine in the striatum of diseased, parkinsonian animals. Previously, we have shown that intact rats treated with R-apomorphine do not have any significant differences in striatal DA and DOPAC content, and DA turnover when compared to intact rats that didn’t receive any treatment [14]. Fur- thermore, the number of tyrosine hydroxylase immuno- reactive neurons in the SNpc were not different between intact rats and intact rats treated with R-apomorphine. 5. Conclusion Our data confirm the neuroprotective effects of R-apo- morphine in the unilateral striatal 6-OHDA parkinsonian rat model and suggest that they involve the alteration of the striatal gene and the protein expression levels of the anti-inflammatory CRHR1 receptor and the pro-inflam- matory CRHR2 receptor. Furthermore, treatment with R- apomorphine led to an upregulation of the NPY1R and NPY2R at the mRNA level. These results provide a bet- ter insight for understanding our previously observed neuroprotective effects of R-apomorphine in the unilat- eral striatal 6-OHDA parkinsonian rat model and confirm its potential anti-inflammatory action. However, addi- tional investigations, e.g. functional studies, are required in order to confirm these findings. Despite the value of the 6-OHDA rat model, as illustrated in this work, one should be aware that the mechanism of 6-OHDA proba- bly only reflects a small fraction of the events occurring in human Parkinson’s disease. 6-OHDA induces rather acute effects, which differ significantly from the slowly progressive pathology of human Parkinson’s disease. Moreover, Lewy body pathology is not present in the surviving neurons, and no other brain areas involved in Parkinson’s disease are affected, such as the olfactory bulb and the locus coeruleus [69-71]. Therefore, further work would be valuable in understanding the potential therapeutic value of targeting the CRH and NPY recap- tors in Parkinson’s disease. 6. Acknowledgements The authors wish to acknowledge the animal care by Mrs M. Cresens and technical assistance of Mrs. P. Verdood, Mr. E. Questier, Mr. S. Branson, Mrs R. Berckmans, Mr G. De Smet, Mrs C. De Rijck, and Mrs R.-M. Geens. We also thank Prof. Dr. Pharm. V. Rogiers, Prof. Dr. H. Heimberg and Prof. Dr. R Kooijman for the use of their laboratory facilities. This work was conducted with fi- nancial support of the Institute for the promotion of In- novation by Science and Technology in Flanders (IWT) (IWT420), National Fund for Scientific Research (FWO- Vlaanderen) (G.0071.05) and of the Research Council of the Vrije Universiteit Brussel. M. Varçin is holder of a research grant from the IWT (IWT420). E. Bentea is a research fellow of the FWO Vlaanderen. REFERENCES [1] A. H. Schapira, “Neurobiology and Treatment of Parkin- Open Access NM
 Involvement of CRH Receptors in the Neuroprotective Action of R-Apomorphine in the Striatal 6-OHDA Rat Model 315 son’s Disease,” Trends in Pharmacological Sciences, Vol. 30, No. 1, 2009, pp. 41-47. http://dx.doi.org/10.1016/j.tips.2008.10.005 [2] M. B. Youdim, W. J. Geldenhuys and C. J. Van der Schyf, “Why Should We Use Multifunctional Neuroprotective and Neurorestorative Drugs for Parkinson’s Disease?” Parkinsonism & Related Disorders, Vol. 13, No. Suppl 3, 2007, pp. S281-S291. http://dx.doi.org/10.1016/S1353-8020(08)70017-8 [3] P. A. LeWitt, “Subcutaneously Administered Apomor- phine: Pharmacokinetics and Metabolism,” Neurology, Vol. 62, No. 6, 2004, pp. S8-S11. http://dx.doi.org/10.1212/WNL.62.6_suppl_4.S8 [4] I. U. Haq, P. A. Lewitt and H. H. Fernandez, “Apomor- phine Therapy in Parkinson’s Disease: A Review,” Expert Opinion on Pharmacotherapy, Vol. 8, No. 16, 2007, pp. 2799-2809. http://dx.doi.org/10.1517/14656566.8.16.2799 [5] P. A. LeWitt, et al., “Open-Label Study Assessment of Safety and Adverse Effects of Subcutaneous Apomor- phine Injections in Treating ‘off’ Episodes in Advanced Parkinson Disease,” Clinical Neuropharmacology, Vol. 32, No. 2, 2009, pp. 89-93. http://dx.doi.org/10.1097/WNF.0b013e31816d91f9 [6] F. Stocchi, “Use of Apomorphine in Parkinson’s Dis- ease,” Neurological Sciences, Vol. 29, No. Suppl 5, 2008, pp. S383-S386. http://dx.doi.org/10.1007/s10072-008-1053-8 [7] M. Kyriazis, “Neuroprotective, Anti-Apoptotic Effects of Apomorphine,” Journal of Anti-Aging Medicine, Vol. 6, No. 1, 2003, pp. 21-28. http://dx.doi.org/10.1089/109454503765361551 [8] S. Ribaric, “The Pharmacological Properties and Thera- peutic Use of Apomorphine,” Molecules, Vol. 17, No. 5, 2012, pp. 5289-5309. http://dx.doi.org/10.3390/molecules17055289 [9] E. Grunblatt, et al., “Gene Expression Analysis in N- methyl-4-phenyl-1,2,3,6-tetrahydropyridine Mice Model of Parkinson’s Disease Using cDNA Microarray: Effect of R-Apomorphine,” Journal of Neurochemistry, Vol. 78, No. 1, 2001, pp. 1-12. http://dx.doi.org/10.1046/j.1471-4159.2001.00397.x [10] E. Grunblatt, et al., “Apomorphine Protects against MPTP- Induced Neurotoxicity in Mice,” Movement Disorders, Vol. 14, No. 4, 1999, pp. 612-618. http://dx.doi.org/10.1002/1531-8257(199907)14:4<612:: AID-MDS1010>3.0.CO;2-6 [11] G. Battaglia, et al., “Continuous Subcutaneous Infusion of Apomorphine Rescues Nigro-Striatal Dopaminergic Terminals Following MPTP Injection in Mice,” Neuro- pharmacology, Vol. 42, No. 3, 2002, pp. 367-373. http://dx.doi.org/10.1016/S0028-3908(01)00178-2 [12] G. Battaglia, et al., “Morphological and Biochemical Evidence That Apomorphine Rescues Striatal Dopamine Terminals and Prevents Methamphetamine Toxicity,” Annals of the New York Academy of Sciences, Vol. 965, 2002, pp. 254-266. http://dx.doi.org/10.1111/j.1749-6632.2002.tb04167.x [13] E. E. Sam and N. Verbeke, “Free Radical Scavenging Properties of Apomorphine Enantiomers and Dopamine: Possible Implication in Their Mechanism of Action in Parkinsonism,” Journal of Neural Transmission Parkin- son’s Disease and Dementia Section, Vol. 10, No. 2-3, 1995, pp. 115-27. http://dx.doi.org/10.1007/BF02251227 [14] H. Yuan, et al., “Neuroprotective and Neurotrophic Effect of Apomorphine in the Striatal 6-OHDA-Lesion Rat Mo- del of Parkinson’s Disease,” Brain Research, Vol. 1026, No. 1, 2004, pp. 95-107. http://dx.doi.org/10.1016/j.brainres.2004.08.015 [15] Y. K. Chen, et al., “Potent, Hydroxyl Radical-Scavenging Effect of Apomorphine with Iron and Dopamine Perfu- sion in Rat Striatum,” Brain Research, Vol. 896, No. 1-2, 2001, pp. 165-168. http://dx.doi.org/10.1016/S0006-8993(01)02081-9 [16] F. Fornai, et al., “Dose-Dependent Protective Effects of Apomorphine against Methamphetamine-Induced Nigro- striatal Damage,” Brain Research, Vol. 898, No. 1, 2001, pp. 27-35. http://dx.doi.org/10.1016/S0006-8993(01)02125-4 [17] E. Himeno, et al., “Apomorphine Treatment in Alzheimer Mice Promoting Amyloid-Beta Degradation,” Annals of Neurology, Vol. 69, No. 2, 2011, pp. 248-256. http://dx.doi.org/10.1002/ana.22319 [18] M. Gassen, et al., “Apomorphine Is a Highly Potent Free Radical Scavenger in Rat Brain Mitochondrial Fraction,” European Journal of Pharmacology, Vol. 308, No. 2, 1996, pp. 219-225. http://dx.doi.org/10.1016/0014-2999(96)00291-9 [19] G. Williamson, K. Faulkner and G. W. Plumb, “Glucosi- nolates and Phenolics as Antioxidants from Plant Foods,” European Journal of Cancer Prevention, Vol. 7, No. 1, 1998, pp. 17-21. [20] A. Ubeda, et al., “Iron-Reducing and Free-Radical- Scavenging Properties of Apomorphine and Some Relat- ed Benzylisoquinolines,” Free Radical Biology & Medi- cine, Vol. 15, No. 2, 1993, pp. 159-167. http://dx.doi.org/10.1016/0891-5849(93)90055-Y [21] H. Hara, M. Ohta and T. Adachi, “Apomorphine Protects against 6-Hydroxydopamine-Induced Neuronal Cell Death through Activation of the Nrf2-ARE Pathway,” Journal of Neuroscience Research, Vol. 84, No. 4, 2006, pp. 860-866. http://dx.doi.org/10.1002/jnr.20974 [22] E. Grunblatt, et al., “Effects of R- and S-Apomorphine on MPTP-Induced Nigro-Striatal Dopamine Neuronal Loss,” Journal of Neurochemistry, Vol. 77, No. 1, 2001, pp. 146-156. http://dx.doi.org/10.1046/j.1471-4159.2001.t01-1-00227. x [23] L. Ma, et al., “Activation of Glutathione Peroxidase and Inhibition of p53-Related Apoptosis by Apomorphine,” Journal of Alzheimer’s Disease, Vol. 27, No. 1, 2011, pp. 225-237. [24] O. Weinreb, S. Mandel and M. B. Youdim, “Gene and Protein Expression Profiles of Anti- and Pro-Apoptotic Actions of Dopamine, R-Apomorphine, Green Tea Poly- phenol (-)-Epigallocatechine-3-Gallate, and Melatonin,” Open Access NM
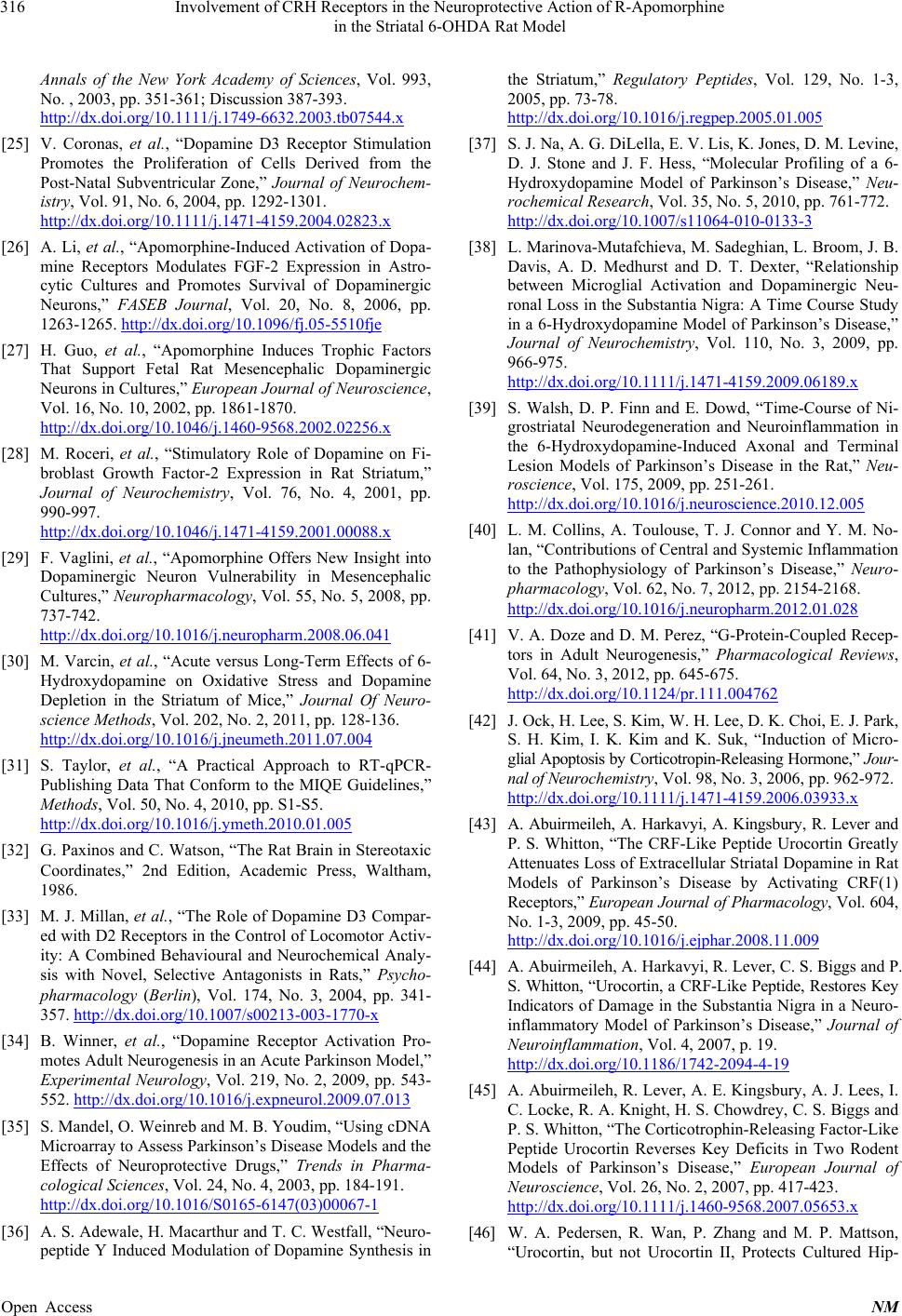 Involvement of CRH Receptors in the Neuroprotective Action of R-Apomorphine in the Striatal 6-OHDA Rat Model 316 Annals of the New York Academy of Sciences, Vol. 993, No. , 2003, pp. 351-361; Discussion 387-393. http://dx.doi.org/10.1111/j.1749-6632.2003.tb07544.x [25] V. Coronas, et al., “Dopamine D3 Receptor Stimulation Promotes the Proliferation of Cells Derived from the Post-Natal Subventricular Zone,” Journal of Neurochem- istry, Vol. 91, No. 6, 2004, pp. 1292-1301. http://dx.doi.org/10.1111/j.1471-4159.2004.02823.x [26] A. Li, et al., “Apomorphine-Induced Activation of Dopa- mine Receptors Modulates FGF-2 Expression in Astro- cytic Cultures and Promotes Survival of Dopaminergic Neurons,” FASEB Journal, Vol. 20, No. 8, 2006, pp. 1263-1265. http://dx.doi.org/10.1096/fj.05-5510fje [27] H. Guo, et al., “Apomorphine Induces Trophic Factors That Support Fetal Rat Mesencephalic Dopaminergic Neurons in Cultures,” European Journal of Neuroscience, Vol. 16, No. 10, 2002, pp. 1861-1870. http://dx.doi.org/10.1046/j.1460-9568.2002.02256.x [28] M. Roceri, et al., “Stimulatory Role of Dopamine on Fi- broblast Growth Factor-2 Expression in Rat Striatum,” Journal of Neurochemistry, Vol. 76, No. 4, 2001, pp. 990-997. http://dx.doi.org/10.1046/j.1471-4159.2001.00088.x [29] F. Vaglini, et al., “Apomorphine Offers New Insight into Dopaminergic Neuron Vulnerability in Mesencephalic Cultures,” Neuropharmacology, Vol. 55, No. 5, 2008, pp. 737-742. http://dx.doi.org/10.1016/j.neuropharm.2008.06.041 [30] M. Varcin, et al., “Acute versus Long-Term Effects of 6- Hydroxydopamine on Oxidative Stress and Dopamine Depletion in the Striatum of Mice,” Journal Of Neuro- science Methods, Vol. 202, No. 2, 2011, pp. 128-136. http://dx.doi.org/10.1016/j.jneumeth.2011.07.004 [31] S. Taylor, et al., “A Practical Approach to RT-qPCR- Publishing Data That Conform to the MIQE Guidelines,” Methods, Vol. 50, No. 4, 2010, pp. S1-S5. http://dx.doi.org/10.1016/j.ymeth.2010.01.005 [32] G. Paxinos and C. Watson, “The Rat Brain in Stereotaxic Coordinates,” 2nd Edition, Academic Press, Waltham, 1986. [33] M. J. Millan, et al., “The Role of Dopamine D3 Compar- ed with D2 Receptors in the Control of Locomotor Activ- ity: A Combined Behavioural and Neurochemical Analy- sis with Novel, Selective Antagonists in Rats,” Psycho- pharmacology (Berlin), Vol. 174, No. 3, 2004, pp. 341- 357. http://dx.doi.org/10.1007/s00213-003-1770-x [34] B. Winner, et al., “Dopamine Receptor Activation Pro- motes Adult Neurogenesis in an Acute Parkinson Model,” Experimental Neurology, Vol. 219, No. 2, 2009, pp. 543- 552. http://dx.doi.org/10.1016/j.expneurol.2009.07.013 [35] S. Mandel, O. Weinreb and M. B. Youdim, “Using cDNA Microarray to Assess Parkinson’s Disease Models and the Effects of Neuroprotective Drugs,” Trends in Pharma- cological Sciences, Vol. 24, No. 4, 2003, pp. 184-191. http://dx.doi.org/10.1016/S0165-6147(03)00067-1 [36] A. S. Adewale, H. Macarthur and T. C. Westfall, “Neuro- peptide Y Induced Modulation of Dopamine Synthesis in the Striatum,” Regulatory Peptides, Vol. 129, No. 1-3, 2005, pp. 73-78. http://dx.doi.org/10.1016/j.regpep.2005.01.005 [37] S. J. Na, A. G. DiLella, E. V. Lis, K. Jones, D. M. Levine, D. J. Stone and J. F. Hess, “Molecular Profiling of a 6- Hydroxydopamine Model of Parkinson’s Disease,” Neu- rochemical Research, Vol. 35, No. 5, 2010, pp. 761-772. http://dx.doi.org/10.1007/s11064-010-0133-3 [38] L. Marinova-Mutafchieva, M. Sadeghian, L. Broom, J. B. Davis, A. D. Medhurst and D. T. Dexter, “Relationship between Microglial Activation and Dopaminergic Neu- ronal Loss in the Substantia Nigra: A Time Course Study in a 6-Hydroxydopamine Model of Parkinson’s Disease,” Journal of Neurochemistry, Vol. 110, No. 3, 2009, pp. 966-975. http://dx.doi.org/10.1111/j.1471-4159.2009.06189.x [39] S. Walsh, D. P. Finn and E. Dowd, “Time-Course of Ni- grostriatal Neurodegeneration and Neuroinflammation in the 6-Hydroxydopamine-Induced Axonal and Terminal Lesion Models of Parkinson’s Disease in the Rat,” Neu- roscience, Vol. 175, 2009, pp. 251-261. http://dx.doi.org/10.1016/j.neuroscience.2010.12.005 [40] L. M. Collins, A. Toulouse, T. J. Connor and Y. M. No- lan, “Contributions of Central and Systemic Inflammation to the Pathophysiology of Parkinson’s Disease,” Neuro- pharmacology, Vol. 62, No. 7, 2012, pp. 2154-2168. http://dx.doi.org/10.1016/j.neuropharm.2012.01.028 [41] V. A. Doze and D. M. Perez, “G-Protein-Coupled Recep- tors in Adult Neurogenesis,” Pharmacological Reviews, Vol. 64, No. 3, 2012, pp. 645-675. http://dx.doi.org/10.1124/pr.111.004762 [42] J. Ock, H. Lee, S. Kim, W. H. Lee, D. K. Choi, E. J. Park, S. H. Kim, I. K. Kim and K. Suk, “Induction of Micro- glial Apoptosis by Corticotropin-Releasing Hormone,” Jour- nal of Neurochemistry, Vol. 98, No. 3, 2006, pp. 962-972. http://dx.doi.org/10.1111/j.1471-4159.2006.03933.x [43] A. Abuirmeileh, A. Harkavyi, A. Kingsbury, R. Lever and P. S. Whitton, “The CRF-Like Peptide Urocortin Greatly Attenuates Loss of Extracellular Striatal Dopamine in Rat Models of Parkinson’s Disease by Activating CRF(1) Receptors,” European Journal of Pharmacology, Vol. 604, No. 1-3, 2009, pp. 45-50. http://dx.doi.org/10.1016/j.ejphar.2008.11.009 [44] A. Abuirmeileh, A. Harkavyi, R. Lever, C. S. Biggs and P. S. Whitton, “Urocortin, a CRF-Like Peptide, Restores Key Indicators of Damage in the Substantia Nigra in a Neuro- inflammatory Model of Parkinson’s Disease,” Journal of Neuroinflammation, Vol. 4, 2007, p. 19. http://dx.doi.org/10.1186/1742-2094-4-19 [45] A. Abuirmeileh, R. Lever, A. E. Kingsbury, A. J. Lees, I. C. Locke, R. A. Knight, H. S. Chowdrey, C. S. Biggs and P. S. Whitton, “The Corticotrophin-Releasing Factor-Like Peptide Urocortin Reverses Key Deficits in Two Rodent Models of Parkinson’s Disease,” European Journal of Neuroscience, Vol. 26, No. 2, 2007, pp. 417-423. http://dx.doi.org/10.1111/j.1460-9568.2007.05653.x [46] W. A. Pedersen, R. Wan, P. Zhang and M. P. Mattson, “Urocortin, but not Urocortin II, Protects Cultured Hip- Open Access NM
 Involvement of CRH Receptors in the Neuroprotective Action of R-Apomorphine in the Striatal 6-OHDA Rat Model 317 pocampal Neurons from Oxidative and Excitotoxic Cell Death via Corticotropin-Releasing Hormone Receptor Type I,” Journal of Neuroscience, Vol. 22, No. 2, 2002, pp. 404- 412. [47] E. Gonzalez-Rey, A. Fernandez-Martin, A. Chorny and M. Delgado, “Therapeutic Effect of Urocortin and Adreno- medullin in a Murine Model of Crohn’s Disease,” Gut, Vol. 55, No. 6, 2006, pp. 824-832. http://dx.doi.org/10.1136/gut.2005.084525 [48] H. Y. Huang, S. Z. Lin, W. F. Chen, K. W. Li, J. S. Kuo and M. J. Wang, “Urocortin Modulates Dopaminergic Neu- ronal Survival via Inhibition of Glycogen Synthase Kinase- 3β and Histone Deacetylase,” Neurobiology of Aging, Vol. 32, No. 9, 2011, pp. 1662-1677. http://dx.doi.org/10.1016/j.neurobiolaging.2009.09.010 [49] Y. Kim, M. K. Park and S. Chung, “Protective Effect of Urocortin on 1-Methyl-4-Phenylpyridinium-Induced Dopa- minergic Neuronal Death,” Molecules and Cells, Vol. 30, No. 5, 2010, pp. 427-433. http://dx.doi.org/10.1007/s10059-010-0132-x [50] A. C. Moss, P. Anton, T. Savidge, et al., “Urocortin II Me- diates Pro-Inflammatory Effects in Human Colonocytes via Corticotropin-Releasing Hormone Receptor 2α,” Gut, Vol. 56, No. 9, 2007, pp. 1210-1217. http://dx.doi.org/10.1136/gut.2006.110668 [51] E. Kokkotou, D. Torres, A. C. Moss, M. O'Brien, D. E. Grigoriadis, K. Karalis and C. Pothoulakis, “Corticotropin- Releasing Hormone Receptor 2-Deficient Mice Have Re- duced Intestinal Inflammatory Responses,” Journal of Im- munology, Vol. 177, No. 5, 2006, pp. 3355-3361. [52] M. Decressac, L. Prestoz, J. Veran, A. Cantereau, M. Ja- ber and A. Gaillard, “Neuropeptide Y Stimulates Prolif- eration, Migration and Differentiation of Neural Precur- sors from the Subventricular Zone in Adult Mice,” Neu- robiology of Disease, Vol. 34, No. 3, 2009, pp. 441-449. http://dx.doi.org/10.1016/j.nbd.2009.02.017 [53] M. Dimitrijevic, S. Stanojevića, K. Mitića, N. Kuštrimo- vića, V. Vujićb, T. Miletića and V. Kovačević-Jovanovića, “The Anti-Inflammatory Effect of Neuropeptide Y (NPY) in Rats Is Dependent on Dipeptidyl Peptidase 4 (DP4) Activity and Age,” Peptides, Vol. 29, No. 12, 2008, pp. 2179-2187. http://dx.doi.org/10.1016/j.peptides.2008.08.017 [54] M. Decressac, B. Wright, B. David, P. Tyers, M. Jaber, R. A. Barker and A. Gaillard, “Exogenous Neuropeptide Y Promotes in Vivo Hippocampal Neurogenesis,” Hippo- campus, Vol. 21, No. 3, 2011, pp. 233-238. http://dx.doi.org/10.1002/hipo.20765 [55] N. Thiriet, X. L. Deng, M. Solinas, B. Ladenheim, W. Curtis, S. R. Goldberg, R. D. Palmiter and J. L. Cadet, “Neuropeptide Y Protects against Methamphetamine-In- duced Neuronal Apoptosis in the Mouse Striatum,” Jour- nal of Neuroscience, Vol. 25, No. 22 , 2005, pp. 5273- 5279. http://dx.doi.org/10.1523/JNEUROSCI.4893-04.2005 [56] A. S. Adewale, H. Macarthur and T. C. Westfall, “Neu- ropeptide Y-Induced Enhancement of the Evoked Release of Newly Synthesized Dopamine in Rat Striatum: Media- tion by Y2 Receptors,” Neuropharmacology, Vol. 52, No. 6, 2007, pp. 1396-1402. http://dx.doi.org/10.1016/j.neuropharm.2007.01.018 [57] D. Quarta, C. P. Leslie, R. Carletti, E. Valerio and L. Ca- berlotto, “Central Administration of NPY or an NPY-Y5 Selective Agonist Increase in Vivo Extracellular Mono- amine Levels in Mesocorticolimbic Projecting Areas,” Neuropharmacology, Vol. 60, No. 2-3, 2011, pp. 328-335. http://dx.doi.org/10.1016/j.neuropharm.2010.09.016 [58] E. Zambello, L. Zanetti, G. F. Hédou, O. Angelici, R. Ar- ban, R. O. Tasan, G. Sperk and L. Caberlotto, “Neuro- peptide Y-Y2 Receptor knockout Mice: Influence of Ge- netic Background on Anxiety-Related Behaviors,” Neu- roscience, Vol. 176, No. , 2010, pp. 420-430. http://dx.doi.org/10.1016/j.neuroscience.2010.10.075 [59] M. Smialowska, H. Domin, B. Zieba, E. Koźniewska, R. Michalik, P. Piotrowski and M. Kajta, “Neuroprotective Effects of Neuropeptide Y-Y2 and Y5 Receptor Agonists in Vitro and in Vivo,” Neuropeptides, Vol. 43, No. 3, 2009, pp. 235-249. http://dx.doi.org/10.1016/j.npep.2009.02.002 [60] B. Mertens, A. Massie, Y. Michotte and S. Sarre, “Effect of Nigrostriatal Damage Induced by 6-Hydroxydopamine on the Expression of Glial Cell Line-Derived Neurotro- phic Factor in the Striatum of the Rat,” Neuroscience, Vol. 162, No. 1, 2009, pp. 148-154. http://dx.doi.org/10.1016/j.neuroscience.2009.04.036 [61] S. Mandel, E. Grünblatt, P. Riederer and M. B. Youdim, “Genes and Oxidative Stress in Parkinsonism: cDNA Mi- croarray Studies,” Advances in Neurology, Vol. 91, 2003, pp. 123-132. [62] M. Ohta, I. Mizuta, K. Ohta, M. Nishimura, E. Mizuta, K. Hayashid and S. Kuno, “Apomorphine Up-Regulates NGF and GDNF Synthesis in Cultured Mouse Astrocytes,” Biochemical and Biophysical Research Communications, Vol. 272, No. 1, 2000, pp. 18-22. http://dx.doi.org/10.1006/bbrc.2000.2732 [63] I. Branchi, I. D’Andreaa, M. Armida, et al., “Striatal 6- OHDA Lesion in Mice: Investigating Early Neurochemical Changes Underlying Parkinson’s Disease,” Behavioural Brain Research, Vol. 208, No. 1, 2010, pp. 137-143. http://dx.doi.org/10.1016/j.bbr.2009.11.020 [64] V. Francardo, A. Recchia, N. Popovic, D. Andersson, H. Nissbrandt and M. A. Cenci, “Impact of the Lesion Pro- cedure on the Profiles of Motor Impairment and Molecu- lar Responsiveness to L-DOPA in the 6-Hydroxydopa- mine Mouse Model of Parkinson’s Disease,” Neurobiol- ogy of Disease, Vol. 42, No. 3, 2011, pp. 327-340. http://dx.doi.org/10.1016/j.nbd.2011.01.024 [65] S. J. Haas, A. Ahrens, S. Petrov, O. Schmitt and A. Wree, “Quinolinic Acid Lesions of the Caudate Putamen in the Rat Lead to a Local Increase of Ciliary Neurotrophic Fac- tor,” Journal of Anatomy, Vol. 204, No. 4, 2004, pp. 271- 281. http://dx.doi.org/10.1111/j.0021-8782.2004.00279.x [66] R. W. Rodrigues, V. C. Gomide and G. Chadi, “Astro- glial and Microglial Activation in the Wistar Rat Ventral Tegmental Area after a Single Striatal Injection of 6-Hy- droxydopamine,” International Journal of Neuroscience, Vol. 114, No. 2, 2004, pp. 197-216. Open Access NM
 Involvement of CRH Receptors in the Neuroprotective Action of R-Apomorphine in the Striatal 6-OHDA Rat Model Open Access NM 318 http://dx.doi.org/10.1080/00207450490249338 [67] V. C. Gomide, G. A. Silveira and G. Chadi, “Transient and Widespread Astroglial Activation in the Brain after a Striatal 6-OHDA-Induced Partial Lesion of the Nigrostri- atal System,” International Journal of Neuroscience, Vol. 115, No. 1, 2005, pp. 99-117. http://dx.doi.org/10.1080/00207450490512696 [68] J. Henning, U. Strauss, A. Wree, J. Gimsa, A. Rolfs, R. Benecke and U. Gimsa, “Differential Astroglial Activation in 6-Hydroxydopamine Models of Parkinson’s Disease,” Neuroscience Research, Vol. 62, No. 4, 2008, pp. 246- 253. http://dx.doi.org/10.1016/j.neures.2008.09.001 [69] M. E. Emborg, “Evaluation of Animal Models of Parkin- son’s Disease for Neuroprotective Strategies,” Journal of Neuroscience Methods, Vol. 139, No. 2, 2004, pp. 121- 143. http://dx.doi.org/10.1016/j.jneumeth.2004.08.004 [70] F. Blandini, M. T. Armentero and E. Martignoni, “The 6-Hydroxydopamine Model: News from the Past,” Park- insonism & Related Disorders, Vol. 14, Suppl. 2, 2008, pp. S124-S129. http://dx.doi.org/10.1016/j.parkreldis.2008.04.015 [71] S. Duty and P. Jenner, “Animal Models of Parkinson’s Disease: A Source of Novel Treatments and Clues to the Cause of the Disease,” British Journal of Pharmacology, Vol. 164, No. 4, 2011, pp. 1357-1391. http://dx.doi.org/10.1111/j.1476-5381.2011.01426.x
|