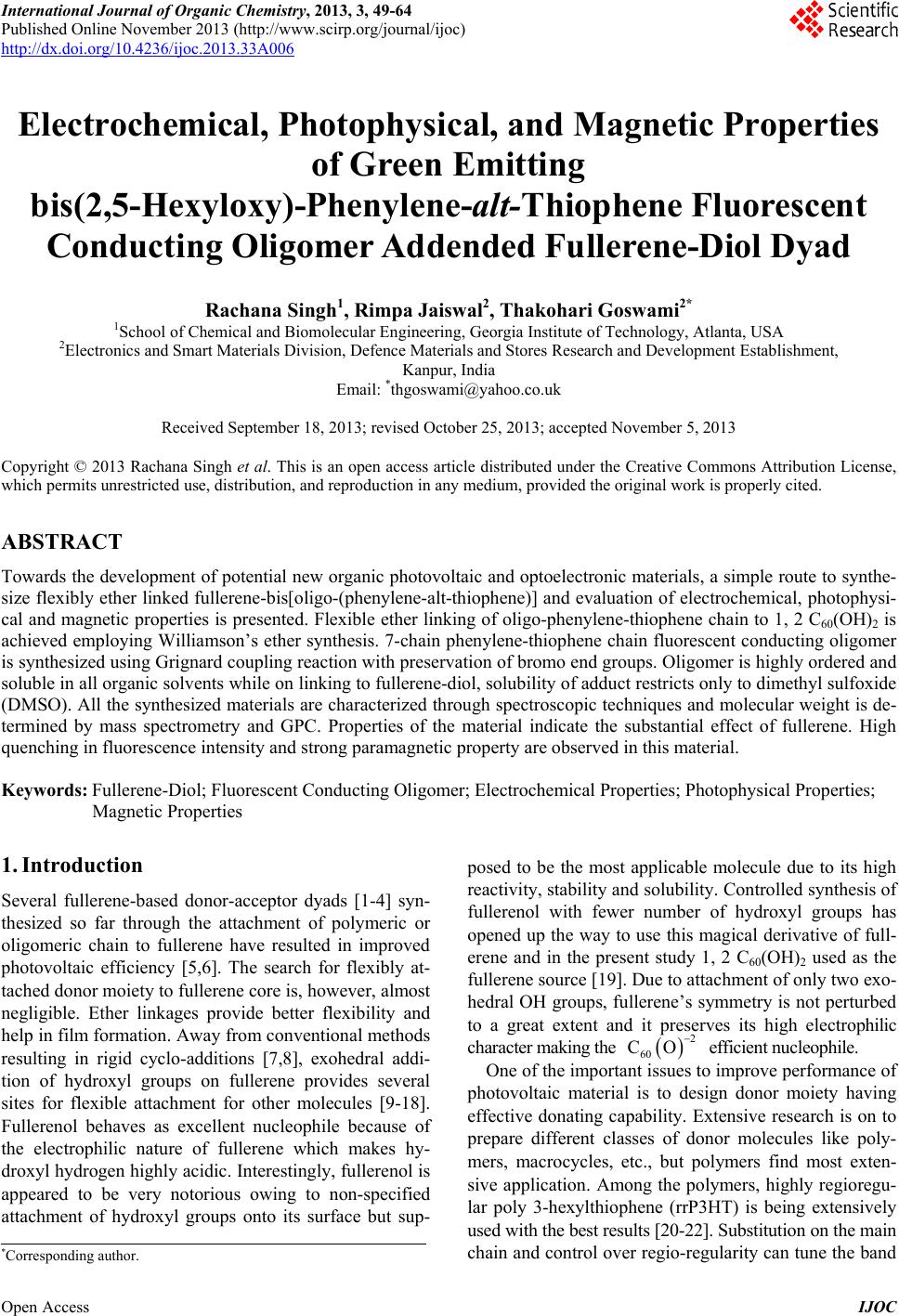 International Journal of Organic Chemistry, 2013, 3, 49-64 Published Online November 2013 (http://www.scirp.org/journal/ijoc) http://dx.doi.org/10.4236/ijoc.2013.33A006 Open Access IJOC Electrochemical, Photophysical, and Magnetic Properties of Green Emitting bis(2,5-Hexyloxy)-Phenylene-alt-Thiophene Fluorescent Conducting Oligomer Addended Fullerene-Diol Dyad Rachana Singh1, Rimpa Jaiswal2, Thakohari Goswami2* 1School of Chemical and Biomolecular Engineering, Georgia Institute of Technology, Atlanta, USA 2Electronics and Smart Materials Division, Defence Materials and Stores Research and Development Establishment, Kanpur, India Email: *thgoswami@yahoo.co.uk Received September 18, 2013; revised October 25, 2013; accepted November 5, 2013 Copyright © 2013 Rachana Singh et al. This is an open access article distributed under the Creative Commons Attribution License, which permits unrestricted use, distribution, and reproduction in any medium, provided the original work is properly cited. ABSTRACT Towards the development of potential new organic photovoltaic and optoelectronic materials, a simple route to synthe- size flexibly ether linked fullerene-bis[oligo-(phenylene-alt-thiophene)] and evaluation of electrochemical, photophysi- cal and magnetic properties is presented. Flexible ether linking of oligo-phenylene-thiophene chain to 1, 2 C60(OH)2 is achieved employing Williamson’s ether synthesis. 7-chain phenylene-thiophene chain fluorescent conducting oligomer is synthesized using Grignard coupling reaction with preservation of bromo end groups. Oligomer is highly ordered and soluble in all organic solvents while on linking to fullerene-diol, solubility of adduct restricts only to dimethyl sulfoxide (DMSO). All the synthesized materials are characterized through spectroscopic techniques and molecular weight is de- termined by mass spectrometry and GPC. Properties of the material indicate the substantial effect of fullerene. High quenching in fluorescence intensity and strong paramagnetic property are observed in this material. Keywords: Fullerene-Diol; Fluorescent Conducting Oligomer; Electrochemical Properties; Photophysical Properties; Magnetic Properties 1. Introduction Several fullerene-based donor-acceptor dyads [1-4] syn- thesized so far through the attachment of polymeric or oligomeric chain to fullerene have resulted in improved photovoltaic efficiency [5,6]. The search for flexibly at- tached donor moiety to fullerene core is, however, almost negligible. Ether linkages provide better flexibility and help in film formation. Away from conventional methods resulting in rigid cyclo-additions [7,8], exohedral addi- tion of hydroxyl groups on fullerene provides several sites for flexible attachment for other molecules [9-18]. Fullerenol behaves as excellent nucleophile because of the electrophilic nature of fullerene which makes hy- droxyl hydrogen highly acidic. Interestingly, fullerenol is appeared to be very notorious owing to non-specified attachment of hydroxyl groups onto its surface but sup- posed to be the most applicable molecule due to its high reactivity, stability and solubility. Controlled synthesis of fullerenol with fewer number of hydroxyl groups has opened up the way to use this magical derivative of full- erene and in the present study 1, 2 C60(OH)2 used as the fullerene source [19]. Due to attachment of only two exo- hedral OH groups, fullerene’s symmetry is not perturbed to a great extent and it preserves its high electrophilic character making the efficient nucleophile. 2 60 CO One of the important issues to improve performance of photovoltaic material is to design donor moiety having effective donating capability. Extensive research is on to prepare different classes of donor molecules like poly- mers, macrocycles, etc., but polymers find most exten- sive application. Among the polymers, highly regioregu- lar poly 3-hexylthiophene (rrP3HT) is being extensively used with the best results [20-22]. Substitution on the main chain and control over regio-regularity can tune the band *Corresponding author.
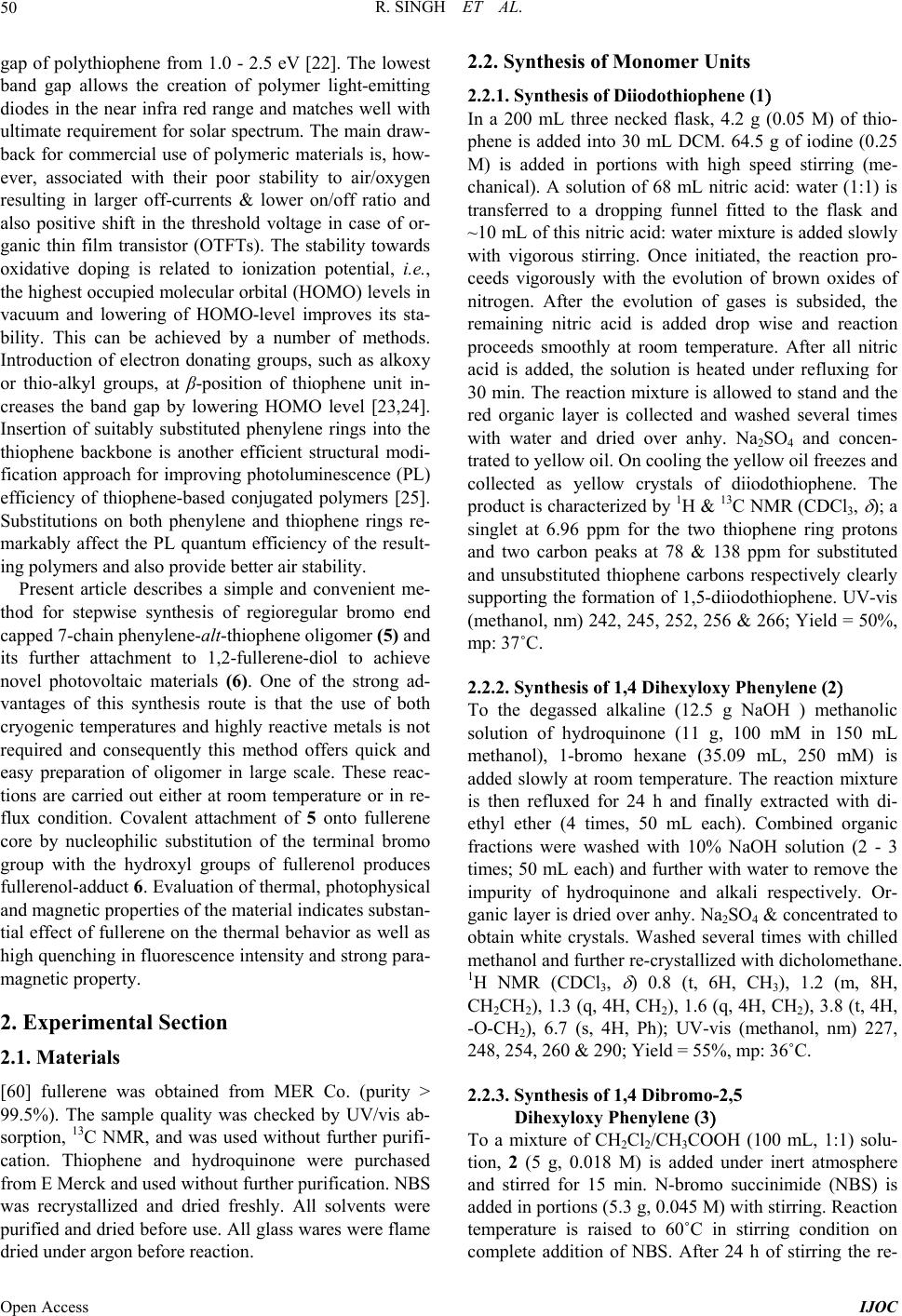 R. SINGH ET AL. 50 gap of polythiophene from 1.0 - 2.5 eV [22]. The lowest band gap allows the creation of polymer light-emitting diodes in the near infra red range and matches well with ultimate requirement for solar spectrum. The main draw- back for commercial use of polymeric materials is, how- ever, associated with their poor stability to air/oxygen resulting in larger off-currents & lower on/off ratio and also positive shift in the threshold voltage in case of or- ganic thin film transistor (OTFTs). The stability towards oxidative doping is related to ionization potential, i.e., the highest occupied molecular orbital (HOMO) levels in vacuum and lowering of HOMO-level improves its sta- bility. This can be achieved by a number of methods. Introduction of electron donating groups, such as alkoxy or thio-alkyl groups, at β-position of thiophene unit in- creases the band gap by lowering HOMO level [23,24]. Insertion of suitably substituted phenylene rings into the thiophene backbone is another efficient structural modi- fication approach for improving photoluminescence (PL) efficiency of thiophene-based conjugated polymers [25]. Substitutions on both phenylene and thiophene rings re- markably affect the PL quantum efficiency of the result- ing polymers and also provide better air stability. Present article describes a simple and convenient me- thod for stepwise synthesis of regioregular bromo end capped 7-chain phenylene-alt-thiophene oligomer (5) and its further attachment to 1,2-fullerene-diol to achieve novel photovoltaic materials (6) . One of the strong ad- vantages of this synthesis route is that the use of both cryogenic temperatures and highly reactive metals is not required and consequently this method offers quick and easy preparation of oligomer in large scale. These reac- tions are carried out either at room temperature or in re- flux condition. Covalent attachment of 5 onto fullerene core by nucleophilic substitution of the terminal bromo group with the hydroxyl groups of fullerenol produces fullerenol-adduct 6. Evaluation of thermal, photophysical and magnetic properties of the material indicates substan- tial effect of fullerene on the thermal behavior as well as high quenching in fluorescence intensity and strong para- magnetic property. 2. Experimental Section 2.1. Materials [60] fullerene was obtained from MER Co. (purity > 99.5%). The sample quality was checked by UV/vis ab- sorption, 13C NMR, and was used without further purifi- cation. Thiophene and hydroquinone were purchased from E Merck and used without further purification. NBS was recrystallized and dried freshly. All solvents were purified and dried before use. All glass wares were flame dried under argon before reaction. 2.2. Synthesis of Monomer Units 2.2.1. Synthesis of Diiodothiophene (1 In a 200 mL three necked flask, 4.2 g (0.05 M of thio- phene is added into 30 mL DCM. 64.5 g of iodine (0.25 M is added in portions with high speed stirring (me- chanical. A solution of 68 mL nitric acid: water (1:1 is transferred to a dropping funnel fitted to the flask and ~10 mL of this nitric acid: water mixture is added slowly with vigorous stirring. Once initiated, the reaction pro- ceeds vigorously with the evolution of brown oxides of nitrogen. After the evolution of gases is subsided, the remaining nitric acid is added drop wise and reaction proceeds smoothly at room temperature. After all nitric acid is added, the solution is heated under refluxing for 30 min. The reaction mixture is allowed to stand and the red organic layer is collected and washed several times with water and dried over anhy. Na2SO4 and concen- trated to yellow oil. On cooling the yellow oil freezes and collected as yellow crystals of diiodothiophene. The product is characterized by 1H & 13C NMR (CDCl3, ); a singlet at 6.96 ppm for the two thiophene ring protons and two carbon peaks at 78 & 138 ppm for substituted and unsubstituted thiophene carbons respectively clearly supporting the formation of 1,5-diiodothiophene. UV-vis (methanol, nm 242, 245, 252, 256 & 266; Yield = 50%, mp: 37˚C. 2.2.2. Synthesis of 1,4 Dihexyloxy Phenylene (2 To the degassed alkaline (12.5 g NaOH methanolic solution of hydroquinone (11 g, 100 mM in 150 mL methanol, 1-bromo hexane (35.09 mL, 250 mM is added slowly at room temperature. The reaction mixture is then refluxed for 24 h and finally extracted with di- ethyl ether (4 times, 50 mL each. Combined organic fractions were washed with 10% NaOH solution (2 - 3 times; 50 mL each and further with water to remove the impurity of hydroquinone and alkali respectively. Or- ganic layer is dried over anhy. Na2SO 4 & concentrated to obtain white crystals. Washed several times with chilled methanol and further re-crystallized with dicholomethane. 1H NMR (CDCl3, ) 0.8 (t, 6H, CH3, 1.2 (m, 8H, CH2CH2, 1.3 (q, 4H, CH2, 1.6 (q, 4H, CH2, 3.8 (t, 4H, -O-CH2, 6.7 (s, 4H, Ph; UV-vis (methanol, nm 227, 248, 254, 260 & 290; Yield = 55%, mp: 36˚C. 2.2.3. Synthesis of 1,4 Dibromo-2,5 Dihexyloxy Phenylene (3 To a mixture of CH2Cl2/CH3COOH (100 mL, 1:1 solu- tion, 2 (5 g, 0.018 M is added under inert atmosphere and stirred for 15 min. N-bromo succinimide (NBS) is added in portions (5.3 g, 0.045 M with stirring. Reaction temperature is raised to 60˚C in stirring condition on complete addition of NBS. After 24 h of stirring the re- Open Access IJOC
 R. SINGH ET AL. 51 action mixture is poured onto 200 mL water. Organic layer is separated and washed 3 - 4 times with 100 mL saturated sodium sulphate solution & further washed with water. Organic layer is dried over anhy. Na2SO4 & concentrated. Pinkish-white colored crystals are obtained and recrystallized with dichloromethane. 1H NMR (CDCl3, : 0.8 (t, 6H, CH3, 1.2 (m, 18H, CH2CH2, 1.3 (q, 4H, CH2, 1.6 (q, 4H, CH2, 3.8 (t, 4H, -O-CH2, 7.06 (s, 2H, Ph; UV-vis (methanol, nm 227, 248, 254, 260 & 29; Yield = 67%, mp: 50˚C. 2.2.4. Synthesis of 4 1) Preparation of Grignard Reagent of Diiodothio- phene: Few drops of mixture of diiodothiophene (1, 1.0078 g, 3 mM and dry dichloro-ethane (1.437 mL, 18 mM in 30 mL dry THF is added to the dry Mg (0.18023, 7.5 mM also in dry THF (20 mL) under inert atmos- phere at refluxing temperature (65˚C - 70˚C. 1 mL of dry dichloro-ethane is further added to initiate the reac- tion. After initiation, the rest of the diiodothiophene/di- chloro-ethane solution is added drop wise over 1 h. Re- action mixture is refluxed for 5 h & cooled to room tem- perature. 2) Grignard coupling reaction: The Grignard of 1 thus prepared, is slowly transferred to the ice-cooled dry THF solution of 3 (3.48 g, 7.5 mM) and Ni (dppe Cl2 (30 mg was added under inert atmosphere with stirring at room temperature. After 24 h, 50 mL of saturated ammonium chloride solution is added and stirred for 30 min. Organic layer is washed several times with water and dried over anhy. Na2SO4 and concentrated to 2 mL. The concentrate is kept in refrigerator for crystallization. The obtained crystals are further recrystallized with di- chloromethane. The FTIR (KBr, cm−1 3050 ( , =C-H, 2919, 2848 ( , C-H, 1676 ( , C=C Ph, 1495 ( , C=C Th, 1462, 1393, 1358 ( , CH3, 1269, 1210 ( , CH2, 1124 ( , C-Br, 1062 ( , =C-O, 1020 ( , C-O ether, 805 & 726 ( , out of plane =C-H. NMR (1H & 13C, CDCl3, 0.8 - 0.9, 1.34 - 1.36, 1.45 - 1.52, 1.75 - 1.85, 3.93 - 3.97, 6.8, 6.9, 7.09; 13.9, 22.5, 25.5, 28.9, 31.4, 70.19, 111.0, 114.2, 118.3, 119.3, 149.9; UV-vis (methanol, nm 249, 255 & 300; Yield = 2 g (83%; mp = 50˚C. 2.2.5. Synthesis of 7-Chain Oligomer (5 Grignard of 1 (0.3359 g, 1 mM, prepared as described above, is added to the ice-cooled dry THF solution of 4 (2 g, 2.5 mM and Ni (ddpe Cl2 (30 mg) under inert at- mosphere in stirring condition. Temperature is raised to r. t (25˚C) and stirring continued for 24 h. Similar work-up steps to that of product 4ii are employed and is recrystal- lized with dichloromethane. FTIR (KBr, cm−1 3000 ( , =C-H, 2932, 2848 ( , C-H, 1677 ( , C=C Ph, 1495 ( , C=C Th, 1462, 1394, 1359 ( , CH3, 1269, 1210 ( , CH2, 1124 ( , C-Br, 1062 ( , =C-O, 1020 ( , C-O ether, 805 & 726 ( , out of plane =C-H. 1H & 13C NMR (CDCl3, 0.8 - 0.9, 1.22, 1.6, 1.7, 3.8, 6.7 - 6.8, 6.8, 7.01; 13.9, 22.3, 25.3, 29.1, 31.2, 70.2, 111, 112, 114, 118, 119, 138, 150, 153. UV-vis (methanol, nm 249, 255 & 300; Yield = 2 g (66%; mp = 55˚C. 2.2.6. Synthesis of Fullerene-Oligomer Adduct (6) To the benzene solution of fullerenol (0.020 g, 0.027 mM), K2CO 3 (anhy, 0.0398 g, 2.88 mM) and 18-crown- 6-ether (catalytic amount) are added at room temperature (25˚C). The benzene solution of oligomer 5 (0.2177 g, 1.44 mM in 10 mL) is added drop wise with stirring at room temperature. The temperature of the reaction mix- ture is now raised to reflux and after 24 h of refluxing; the solid mass is collected by centrifugation and washed several times with methanol: water (80:20) mixture to remove the impurity of K2CO3 and crown ether. Solid dried and collected. Yield = 10 mg (50%; FTIR (KBr, cm−1 3187 ( , =C-H, 2932, 2849 ( , C-H, 1656 ( , C=C Ph, 1495 ( , C=C Th, 1451, 1376 ( , CH3, 1104 ( , C-Br, 1020 ( , C-O ether, 805 & 726 ( , out of plane =C-H; 1H & 13C NMR (DMSO, 0.8 - 1.0, 1.15 - 1.23, 1.6, 2.3, 3.4 - 3.5, 7.16 - 7.18, 7.23 - 7.25; 21, 29, 30, 33, 34, 69.8, 125.6, 128.5, 129.2, 130.08, 148.3, 150.9, 156.2. 3. Results and Discussion 3.1. Synthesis and Identity of Products A simple approach towards the synthesis of highly or- dered oligomeric chain has been adopted starting with 2,5 diiodo thiophene (1) prepared by reported method (Scheme 1). Grignard coupling of (1’) and 1,4 dibromo 2,5-bis hexyloxy benzene (3) results in 3-chained 2,5-bis (4-bromo-2,5(hexyloxy) phenyl) thiophene (4). Repeti- tion of the above reaction using (4) and Grignard of (1) converts (4) into oligomer (5) (Scheme 2) retaining ter- minal bromo groups. The conversion efficiency of the Grignard coupling is excellent (83%). All materials have been purified by flash chromatography. Selective at- tachment of the regioregular oligomer to fullerene core in heterogeneous medium is carried out using benzene solu- tion of fullerene-diol and oligomer (5) with K2CO3 in presence of 18-crown-6-ether. Refluxing for 24 h yields dyad (6) in good yield (Scheme 3). 1,2 C60(OH)2 has been synthesized and purified according to reported method [19]. Oligomer (5) is a pale yellow crystalline solid soluble in most of the organic solvents, while the dyad (6) is black powder with solubility only in DMSO. Product structures are established by spectroscopic characterization. The critical 13C peaks assignment of the oligomer (5 ) suggest three non equivalent carbons (C1, C2, C3) for inner phenylene ring and six for terminal Open Access IJOC
 R. SINGH ET AL. Open Access IJOC 52 SSII i HO OH C6H13OOC6H13 C6H13OOC6H13 Br Br iii Thiophene 1,4- di br omo-2, 5- bi s (hexyloxy)b e nze ne (3) 2,5-diiodothiophene (1) 1,4-bis(hexyloxy)benzene (2) Hydroquinone ii Scheme 1. Synthesis of precursor materials 1 & 3. (i) Iodine (0.25 M), Nitric acid : water (1:1, 68 mL), refluxed for 30 min; (ii) NaOH (12.5 g), 1-bromo hexane (0.25 M), refluxed for 24 h; (iii) CH2Cl2/CH3COOH (100 mL, 1:1), 2 (0.018 M), NBS (0.045 M), 24 h stirring at 60˚C. SII 1SMgIIMg i S C6H13O OC6H13 Br Br 4 3 1 65 2 2 3 4 5 OC6H13 C6H13O 1 65 4 32 ii 2,5-bis(4-bromo-2,5-bis(hexyloxy) phenyl)thiophene (4) S C6H13O OC6H13 Br 1 6 4 3 5 2 3 4 5 OC6H13 C6H13O S C6H13O OC6H13 Br 4 5 1 23 6 2 3 4 5 OC6H13 C6H13O S 6 3 1 4 5 2 2 4 3 2 1 6 5 5 72 43 2,5-bis(4-(5-(4-bromo-2,5-bis(hexyloxy)phenyl) thiophen-2-yl)-2,5-bis(hexyloxy)phenyl)thiophene (5) iii, iv 1' Scheme 2. Synthesis of precursor materials 4 & oligomer 5. (i) Dichloro ethane (18 mL), Mg (7.5 mM), refluxed at 65˚C - 70˚C for 5 h; (ii) THF solution of 3 (7.5 mM), Ni (dppe) Cl2 (30 mg), stirred for 24 h, (iii) 1’; (iv) Ni (dppe Cl2/24 h stirring/r. t. (6) i, ii 1,2 C 60 (OH) 2 phenyl ring, of which C1 and C2 is common for both. Thiophene has two non-equivalent carbons (C2, C3). Overall one should expect eight 13C peaks for (5). On the other hand, 1H NMR assigns four types of non-equivalent protons; inner phenyl ring proton at C3, two different protons for the terminal ring at C3 and C6 and single pro- ton for thiophene at C3. The FTIR spectrum of (5) also nicely accords with the proposed structure. The inter-ring C-C & C-Br streching peak is appearing in product (5). The molecular weight of the oligomer estimated by GPC using polystyrene as standard confirms the 7-ringed oli- gomeric phenylene-thiophene structure with poly-dis- persity ~1.1. The molecular ion peak in ESI-MS (metha- nol, M+-1 at m/z 1521) also adheres with the GPC result. Attachment of oligomer to fullerene core is evident from the disappearance of all the typical peaks of fullerenol and appearance of aromatic & alkyl stretching and bending peaks in FTIR spectrum of (6). The spec- trum also shows peak for C-Br ( at 1104 cm−1 suggest- ing single terminal bromo group involvement in the reac- tion. Fol-O-C ether linkage is ascertained from the ap- Scheme 3. Outline of reaction scheme for the synthesis of phenylene-alt-thiophene addended fullerenediol dyad (6). (i K2CO3 (2.88 mM, 18-Crown-6-Ether; (ii Benzene solution of 5 (1.44 mM, 24 h refluxing.
 R. SINGH ET AL. 53 pearance of broad ( -band at 1020 cm−1. All eight non-equivalent carbon peaks, similar to that of (5), still exist in 13C NMR of the dyad (6). The broad peaks in 1H NMR are shifted down-field due to the attachment of electronegative fullerene into oligomer. Sp3 hybridized fullerene (at the point of attachment) as well as hexyloxy carbon peaks are present. High symmetry of the dyad is as well be determined by NMR spectroscopy. Broad triplet for methyl group of hexyloxy chain, followed by methylene proton peaks between 2.2 - 2.6 ppm are ob- served in 1H NMR of (6). Appearance of single triplet for methylenoxy protons envisaged the equivalent nature of the addend oligomeric chain. Aromatic proton peaks ap- pear between 7.1 - 7.3 ppm. Due to paramagnetic nature of the dyad material, NMR signals are not very sharp. 3.2. Molecular Modeling Effect of exohedral addition of OH-groups in fullerenol and oligomers in dyad is compared with that of fullerene by performing molecular modeling at semiemperical AM1 level using DS visualizer (Figure 1) and with SPARTAN for the lowest energy molecule (1597.3744 Kcal/mol) (Figure 2). C1-C2 bond length of fullerene moiety in derivatives has increased due to addition. Mo- lecular modeling calculation measures C1-C2 bond length of 1.55 - 1.609 Å in diol to compared to 1.38 Å in pris- tine fullerene suggesting conversion of sp2 carbon into sp3. A further nominal increment by ca. 0.03 Å. of C1-C2 bond length has been observed on addition of oligomeric chain. Slight change in bond angle is also observed on oligomer addition. 3.3. Thermal Properties Oligomer 5 is a crystalline solid material possessing good OH HO 1.5515 1.554 1.551 1.580 1.552 1.562 (a) (b) HO OH 104.8o 114.7o 115.7o 112.7o 106.6o 115.4o 113.2o 108.6o 113.8o 110.5o 109.4o 113.2o (c) (d) Figure 1. Semiemperical AM1 molecular modeling using DS visualizer for comparison of bond length ((a) & (b), Å) and bond angles ((c) & (d)) between fullerene-diol and dyad (6). (a) (b) (c) (d) (e) (f) Figure 2. Molecular modeling with SPARTAN for the low- est energy molecule (1597.3744 Kcal/mol) for comparison of bond length (Å) and bond angles (degree) between fullerene- diol and dyad (6). thermal stability. Melting point recorded in DSC is re- ported to be 64.2˚C and the enthalpy of melting (m ) is 121.6 J/g (Figure 3). The oligomer (heating rate of 10˚C/min in nitrogen atmosphere) shows thermal stabil- ity up to 150˚C followed by a sharp degradation. First derivative TGA trace indicate single degradation step (crest temp 318.4˚C) owing to high symmetric structure. It is attributed to the formation of more rigid planar inter- digited π-stack 3D structure and conformational symme- try due to thermal ordering of hexyloxy groups attached in regular fashion at a particular distance. Flexible ether linkages of hexyloxy groups might have assisted in at- tending such geometry. Symmetric structure of 5 is perturbed on chemical at- tachment to fullerene and is clearly evident from the TGA and first derivative TGA thermogram of 6. Adduct 6 still maintain initial thermal stability up to 150˚C and the small weight loss (6.7%) within this temperature range is due to removal of low boiling solvents physi- cally absorbed on the surface. Stepwise removal of ad- dended oligomers is also recorded in first derivative be- fore the structural degradation of fullerene. First step is associated with long temperature zone 150˚C - 410˚C (crest temp 330.78˚C) followed by two sharp steps in the temperature range 425˚C - 520˚C (crest temperature 480.16˚C) and 520˚C - 560˚C (crest temperature 550˚C) respectively (Figure 4). Weight loss associated with each step corresponds to sequential release of terminal bromo Open Access IJOC
 R. SINGH ET AL. Open Access IJOC 54 (a) (b) Figure 3. (a TGA and (b D heating rate of 10˚C/min. SC of oligomer 5 recorded under inert atmosphere and at (a) (b) Figure 4. (a TGA and (b2 atm. ontaining phenylene and attached thiophene ring fol- 3.4. Electrochemical Properties with a computer DSC of adduct 6 recorded at a heating rate of 10˚C /min under N C lowed by middle phenylene and thiophene rings respec- tively. The required amount of absorbed energy associ- ated during sequential release of addended aromatic units is calculated to be 224.5 J/g (DSC of 6 in N2 atm., heat- ing rate 10˚C/min). Cyclic voltammograms are recorded controlled Autolab model 302 Potentiostat at a constant scan rate of 25 mV/s using 0.1 M tetrabutyl ammonium perchlorate (n-Bu4NH4ClO4 in acetonitrile as supporting electrolyte. A three-electrode configuration undivided cell is used: platinum disc working electrode, platinum wire counter electrode and Ag/AgCl (3 M KCl and satu- rated Ag/Cl separated with a diaphragm as reference electrode. Typical cyclic voltammogram of 5 (scans window between –1.2 to 2.0 V) is displayed in Figure 5 and the electrochemical data are presented in Table 1. Formal reduction potential redpapc 2EEE is –0.77 V and the onset reducti re- corded to be –0.66 V. Values of formal oxida poten- tial (Eox and onset oxidation potential (0 ox E are simi- larly measured to be 1.42 V and 1.47 V restively. The measured redox behavior is transposed to estimate the p a an empirical relationship proposed by Bredas et al. [26] on potential ( is ionization potential (I and electron affinity (E using 0 red E tion ecp 0 ox 4.4 eV p IE and 0 red 4.4eV a EE 0 where 0 ox E tion and reduction and are the onset potentials for oxida- relative to Ag/AgCl reference elec- om le and oligo-phenylene analogues su red E trode fr vacuumvel. [27] Electron affinity (LUMO level and ionization potential (HOMO level is calcu- lated as 3.74 eV and 5.87 eV and the difference in energy between Ip and Ea yields the band gap of the material. For 5, the value is 2.13 eV. The measured low band gap value in 5 compared to pristine oligo-thiophene ggest a more regular structure in solution and incorpo- ration of alkoxy substituted phenylene ring alternate to each thiophene can be a better design for obtaining these -conjugated systems. Reports suggest that for thio- phene-phenylene oligomers, the standard formal oxida- tion potential (Eox increases with the introduction of p-phenylene rings into the oligomer [28]. This increase in oxidation potential is ca 0.15 V for one p-phenylene ring and subsequent introduction of p-phenylene rings shift its oxidation potential by ca. 0.10 V. It means that the thio-
 R. SINGH ET AL. 55 -1.0-0.50.0 0.5 1.0 1.5 2.0 -2.0x10-5 0.0 2.0x10-5 4.0x10-5 6.0x10-5 8.0x10-5 current [i] voltage [V] Figure 5. Cyclic voltammogram of oligomer 5 (3 mM in acetonitrile) recorded at 25˚C using sweep rate of 25 mV·s−1 ility than the corresponding oligo-thiophenes. Electro- physical Properties phenylene-thiophene conjugation chain and high (i.e., phenylene band gap materials. Experi- orm at room tem- perature (25˚C) shows maxima at 302 nm which is ap- nergy compared to their phene-phenylene oligomer (5 should have better air sta- b chemical properties of fol-adduct (6) could not be meas- ured due to solubility problem of the material. Operating window of DMSO falls in the range –1 to +1 V and the adduct (6) does not show any characteristic redox peak in this range. 3.5. Photo Electrical and optical properties of copolymers mainly depend on mean length and -electron delocalization [29,30]. The nature of the substituted group generally affects the electronic and optical properties of the conjugated polymers in two ways [31]; the electronic feature of the substituted group and steric hindrance arising from the substituted group. The side chains do not take part directly in the -bonds delocalization, but their steric hindrance could induce a considerable inter-ring twisting, giving rise to a substan- tial reduction of conjugation length. It has been demon- strated both experimentally and theoretically that alkyl substitution in polythiophene gives rise to stronger steric hindrance and increases the torsional angles between aromatic rings (effectively reduces conjugation length) resulting spectral blue shift in absorption [32]. On the other hand, introduction of alkoxy chains at 2- and 5- positions of the phenylene rings induces spectral red shift of the same polymers [33-35]. Strong electron-donating property and less steric hindrance of alkoxy groups compared to alkyl substituents are considered responsible for the spectral red shift and by far, hexyloxy substituents at 2,5-position of phenylene rings have the highest effect on spectral red shift [33]. Theoretical works [36] on the copolymers suggest that the resultant band gap is the weight average band gap value of the individual unit in polymer composed of alternative low (i.e., thiophene mental observation records 20 - 40 nm blue shifts of the absorption maxima (higher energy) in dilute chloroform solution on inserting a single phenylene unit in 5-ring polymer of thiophene analogue [28]. 3.5.1. Absorption Properties Absorption spectrum of (4 in chlorof preciably red-shifted (lower e individual building blocks (1) and (3) because of easy -* transition on increasing the conjugated chain length. A blue-shift (higher energy) in absorption maxima by about 50 nm compared to 3-ring thiophene homologue (Table 2) is indeed observed. The optical-gap calculated from absorption edge (absorption onset; onset 326 nm3.83 eV is substantially higher than its thiophene homologue. The result suggests that the pres- ence of p-phenylene groups does not break OMO-LUMO optical band gap. The absorption maxima of 7-chain oligomer (5 remains the same ( max = 302 nm although optical-gap is slightly reduced (Abs delocalization but do influence their H onset 340 nm3.66 eV on increasing the chain length from 3 to 7 (Figure 6). This result indi- cates that the absorption maxima remains uneffected on inserting 1,4-heylene group be- tween two thiophene units, although optical gap is slightly decreased by ca. 0.17 eV. The optical gap is however significantly higher compared to 7-ring thio- phene homo-polymer (~2.8 eV and the difference is about 0.8 eV and the optical band gap is more compara- ble to 7-ring oligo p-phenylene homo-polymer. The inductive effect due to electron-donating character of an alkoxy group present in 2,5-positions of phenylene ring would expect to contribute red shifting of abs xyloxy substituted phen orption an ol d emission maxima. Indeed, this effect is substantial in fluorescence but in absorption it contributes for blue- shifting. It is attributed that although these alkoxy groups are positioned head-to-tail in adjacent rings but still yields a slightly greater preference for non-coplanar ge- ometry in ground state. Thus, no shifting of absorption maxima on increasing the conjugation chain length can be rationalized by combining the total effect of alkoxy substituted phenylene with thiophene and chain exten- sion. The absorption spectrum in methanol also shows similar spectral features. This indicates that polarity of the solvent does not influence the absorption property. Insolubility of the adduct (6) in common solvent (soluble only in DMSO) prohibits comparison of absorption property. UV-vis spectrum of (6) recorded in DMSO shows high absorption in UV-region and extended tailing in the entire visible region, typically matched with the characteristic of the fullerene derivatives (Figure 6). 3.5.2. Emission Properties Comparative emission spectra of oligomer 5, fulleren Open Access IJOC
 R. SINGH ET AL. Open Access IJOC 56 Table 1. Electrochemica p-Doping (V n-y Levels (eV l data of oligomer 5. Doping (V Energ Eonset Epc Eonset Epc HOMOO Band Gap(eV Epa Epa LUM 1.47 1.55 1.42 −0.66 − 0.77 - −5.87 −3.74 2.13 Table 2. Optical data of 4, 5 and 6. Abs. max (nm) Onset abs. onset(nm)/band gap (eV)Emission em (nm) Stokes’ shift(nm) 3T 342/3.62 433/2.86 91 4 300/4.13 326/3.83 502/2.48 340.66 41686 202 7T 409/3.03 490/2.53 81 5 302/4.10 /3512/2.43 212 6 entire UV 461/2.7 /3.00 436/2. Figure 6. UV-vis spectra of (A) 5 and (B) 6 recoded in nd oligomer-fol adduct (6 measured in solid state using te both the cases (Figure 7). Small perturbations of - 76 nm compared to 5. Con- ju es oped state is EPR inactive, radical(s)/ion(s) and elec- r chloroform and DMSO respectively at room temperature. a excitation wavelength 350 nm ( ex = 350 nm are pre- sented in Figure 7 and photophysical data are complied in Table 2. Fluorescence spectra of 5 show strong single emission peak in green region (512 nm/2.43 eV). A small red-shifting (10 nm) of emission peak in oligomer 5 compared to 4 is observed. It is attributed that this small red-shifting is net balance of two opposing factors oper- ating simultaneously; the presence of phenyl ring in the backbone and contribution of thiophene ring and chain extension. However, both oligomer 5 and monomer 4 show a large Stokes’ shift between absorption and emis- sion peaks. The Stokes’ shift is more than 200 nm and can be ascribed to the formation of more rigid planar inter-digited π-stack 3D structure and conformational symmetry occurring after photo-excitation [37-39] al- lowing a possible exciton migration to long conjugation segments. The large Stokes’ shift of these materials thus promises a possible use as active medium in laser diodes. Fullerene-diol and adduct 6 shows similar spectral pat- rn and the emission peaks appear at 416 and 436 nm in symmetry of fullerene in both fullerenol and adduct (6) result similar spectral patterns. Fullerene core governs the emission peaks positions and are independent of the nature of addends. Fluorescence intensity of the adduct (6) is however highly quenched compared to both fullerenol and oligomer (5) and is also blue shifted com- pared to oligomer 5. This indicates an efficient intra- and inter-molecular electron transfer from oligo-phenylene- alt-thiophene (donor) to the fullerene (acceptor) produc- ing a stable charge-transfer exciplex in photo-excited (Figure 8). Electron acceptor property of fullerene is well known and the functionalization with oligophenylene- alt-thiophene molecules undergoes fast charge-separation (CS) and slow charge-recombination. [40] The stronger quenching in fluorescence intensity in C60-oligothio- phene-C60 triads compared to oligo-thiophene-C60 dyads is observed earlier [41-45]. In addition, adduct (6) also show high blue-shift in photoluminescence spectra ( gation defect and conformational disorder arises due to steric repulsion and distortion of torsional angle between phenylene-thiophene rings on chemical attachment of C60 results blue-shifting [33]. 3.6. Magnetic Properti Oligomer 5 in its pristine un-d indicating an absence of free tronically defect free symmetric structure in solid state. The oligomer behaves like a p-donor in presence of strong acceptor and creates an electronic imbalance within the adduct on chemical attachment to fullerene (acceptor) making it EPR active (Figure 9) [46]. Higher g-value (g = 2.005) compared to both free electron (g = 2.0023) and fullerenol (g = 1.99) owing to strong elec- tronic interaction between oligomer donor chain and
 R. SINGH ET AL. 57 fullerene acceptor producing resultant dipole moment within the dyad molecule. Deviation of g-factor from the free-spin value is a useful indication of the extent of spin-orbit coupling in the paramagnetic species and pro- vides information about the molecular environment of the unpaired electrons. Absorption at higher magnetic field (3515 G) compared to free electron (3300 G) indi- cate electron-electron interaction within the molecule in ground state needing higher energy for electronic transi- tion. Peak to peak width is inversely proportional to the spin-spin relaxation time (8 21.31 10 p TgH ) which depends on the electron – electron interactions. For adduct 6, T2 values are ofs (0.48 × 10−9 s) and peak to peak width ( the order of nanosecond p ) is slightly broadened (13.8G pp H ). The higher peak width compared to pure fullerenol is due to the shorter lifetime of excited stalecule readily relax back to ground state 4. Conclusio tes and the mo n d bis-(2,5-hexyloxy) substitutes ene oligomer and its attachment to Bromo-end cappe phenylene-alt-thioph fullerene-diol are explored for their thermal, electro- chemical, photophysical and magnetic properties. Syn- thesis of oligomer and formation of its adduct with fullerenol are successfully carried out. Thermal disorder of oligomer on chemical attachment to fullerenol is evi- dent from the multi-step degradation of the adduct (6) compared to single step degradation in oligomer (5). Also, the chemical attachment induced paramagnetic character to otherwise EPR inactive material. Low band- gap of the oligomer in solution indicates symmetric pla- nar structure and good electron donating contribution of alkoxy substituents towards easy migration of -elec- trons. Attachment to fullerene moiety results partial dis- tortion of co-planarity and is easily reflected from the Figure 7. Comparative PL spectra of (A oligomer 5, (B C60(OH2 and (C adduct 6 using 350 nm excitation wave- length in solid state at room temperature. S OC6H13 C6H13O O C6H13O OC6H13 S OC6H13 C6H13O Br OC6H13 C6H13O S S OC6H13 C6H13O O C6H13O OC6H13 S OC6H13 C6H13O Br OC6H13 C6H13O S Intramolecular CS Intermolecular CS Figure 8. Schematic diagram of proposed intra- and intermolecular CS processes in 6. Open Access IJOC
 R. SINGH ET AL. 58 [G] 3000 3100 3200 3300 34003500 3600 3700 3800 3900 4000 -2.5 -2.0 -1.5 -1.0 -0.5 0.0 0.5 1.0 1.5 2.0 [*10^ 3] Figure 9. EPR spectra of adduct 6 recorded in solid at room temperature. blue shifting of emission peak. There is considerable charge-transfer interaction between p-type oligomeric chain and n-type fullerene core resulting in the high quenching of fluorescence intensity. Optical properties of the polymer can be tuned by suitable manipulation of the back-bone chain and also by changing the substituents on both phenylene and thiophene rings. Long chain alkoxy pendant groups substantially improve the solubility yet ontribute to retai These types of fullerene-addended materi- als may find potential in solar cells and photo-sensing devices. REFERENCES [1] J. Modin, H. Johansson and H. Grennberg, “New Pyra- zolino- and Pyrrolidino[60]fullerenes with Transition-Me- tal Chelating Pyridine Substitutents: Synthesis and Com- plexation to Ru(II),” Organic Letters, Vol. 7, No. 18, 2005, pp. 3977-3979. http://dx.doi.org/10.1021/ol051468f c excited state. n rigid planar configuration in photo- [2] S. Campidelli, R. Deschenaux, A. Swartz, G. M. A. Rah- man, D. M. Guldi, D. Milic, E. V’azquez and M. Prato “A Dendritic Fullerene-Porphyrin Dyad,” Photochemica & Photobiological Sciences, Vol. 5, No. 12, 2006, pp , l . 1137-1141. http://dx.doi.org/10.1039/b610881d [3] P. Vivo, M. Ojala, V. Chukharev, A. Efimov and H. Lem- metyinen, “Role of a Phthalocyanine-Fullerene Dyad in Multilayered Organic Solar Cells,” Journal of Photo- chemistry and Photobiology A: Chemistry, Vol. 203, No. 2-3, 2009, pp. 125-130. http://dx.doi.org/10.1016/j.jphotochem.2008.12.030 [4] J. Baffreau, L. Ordronneau, L. S. Leroy and P. Hudhomme, “Synthesis of Perylene-3,4-Mono(dicarboximide)-Full- erene C60 Dyads as New Light-Harvesting Systems,” Journal of Organic Chemistry, Vol. 73, No. 16, 2008, pp. 6142. http://dx.doi.org/10.1021/jo800804z [5] W. B. Zhang, Y. Tu, R. Ranjan, R. M. V. Horn, S. Leng, J. Wang, M. J. Polce, C. Wesdemiotis, R. P. Quirk, G. R. Newkome and S. Z. D. Cheng, “Clicking Fullerene with Polymers: Synthesis of [60]Fullerene End-Capped Poly- styrene,” Macromolecules, Vol. 41, No. 3, 2008, pp. 515- 517. http://dx.doi.org/10.1021/ma702345r [6] C. Wang, Z. X. Guo, S. Fu, W. Wu and D. Zhu, “Poly- mers Containing Fullerene or Carbon Nanotube Struc- tures,” Progress in Polymer Science, Vol. 29, No. 11, 2004, p. 1079-1141. http://dx.doi.org/10.1016/j.progpolymsci.2004.08.001 [7] A. L. Ortiz, D. M. Rivera, A. J. Athans and L. Echegoyen, “Regioselective Addition of N-(4-Thiocyanatophenyl)pyr- rolidine Addends to Fullerenes,” European Journal of Organic Chemistry, 2009, p. 3396. [8] Z. Zhou, G. H. Sarova, S. Zhang, Z. Ou, F. T. Tat, K. M. Kadish, L. Echegoyen, D. M. Guldi, D. I. Schuster and S. R. Wilson, “Fullerene Polypyridine Ligands: Synthesis, Ruthenium Complexes, and Electrochemical and Photo- physical Properties,” Chemistry-A European Journal, Vol. 12, No. 16, 2006, pp. 4241-4248. http://dx.doi.org/10.1002/chem.200600021 [9] R. Singh and T. H. Goswami, “Photophysical and Optical Limiting Properties of Multifunctional Hemi-Ortho Ester Derivatives of Fullerenol: Effects of TBAH Doping, Fullerenol Concentration and Solvent Polarity,” Synthetic Metals, Vol. 161, No. 9-10, 2011, pp. 670-679. http://dx.doi.org/10.1016/j.synthmet.2011.01.012 [10] R. Singh and T. H. Goswami, “Effect of Nature of Ad- dends and Ionic Dopant on Magnetic Properties of Mul- tifunctional Star-Like Hemi-Ortho Ester Derivatives of 1, No. 19-20, 2011, 070-2077. j.synthmet.2011.07.015 Fullerenol,” Synthetic Metals, Vol. 16 pp. 2 http://dx.doi.org/10.1016/ [11] R. Singh and T Luminescent Mul- tifunctional Hves of Fullerenol,” . H. Goswami, “Highly emi-Ortho Ester Derivati Synthetic Metals, Vol. 157, No. 22-23, 2007, pp. 951-955. http://dx.doi.org/10.1016/j.synthmet.2007.09.006 [12] R. Singh and T. H. Goswami, “Understanding of Thermo- Gravimetric Analysis to Calculate Number of Addends in Multifunctional Hemi-Ortho Ester Derivatives of Fullere- nol,” Thermochim Acta, Vol. 513, No. 1-2, 2011, pp. 60- 67. http://dx.doi.org/10.1016/j.tca.2010.11.012 [13] R. Singh and T. H. Goswami, “Synthesis and Evaluation of Thermal, Photophysical and Magnetic Properties of Novel Starlike Fullerene-Organosilane Macromolecules,” Journal of Organometallic Chemistry, Vol. 693, No. 11, 2008, pp. 2021-2032. http://dx.doi.org/10.1016/j.jorganchem.2008.03.006 [14] R. Singh and T. H. Goswami, “Acid Catalyzed 1,2 Mi- chael Addition Reaction: A signing Fullerene Core Starlike Macromolecule,” Jou Viable Synthetic Route in De- rnal of Physical Organic Chemistry, Vol. 21, No. 3, 2008, pp. 225-236. http://dx.doi.org/10.1002/poc.1304 [15] T. H. Goswami and R. Singh, “Recent Development of Fullerenol Reseach,” In: C. N. Kramer, Ed., Fullerene Re- search Advances, NOVA Science Publishers, New York, 2007, pp. 55-96. [16] R. Singh and T. H. Goswami, “Thermal Analysis: A Uni- que Method to Estimate the Number of Substituents in Fullerene Derivatives,” Thermochim Acta, Vol. 419, No. 1-2, 2004, pp. 97-104. http://dx.doi.org/10.1016/j.tca.2004.02.001 Open Access IJOC
 R. SINGH ET AL. 59 [17] T. H. Goswami, B. Nandan, S. Alam and G. N. Mathur, “A Selective Reaction of Polyhydroxy Fullerene with Cycloaliphatic Epoxy Resin in Designing Ether Con- nected Epoxy Star U Core,” Polymer, Vol. 44, No. 11, 2003, pp. 3209-3214. tilizing Fullerene as a Molecular http://dx.doi.org/10.1016/S0032-3861(03)00241-6 [18] T. H. Goswami, R. Singh, S. Alam and G. N. Mathur, “One-Pot Synthesis of a Novel Water-Soluble Fullerene- Core Starlike Macromolecule via Successive Michael and Nucleophilic Addition Reaction,” Chemical Materials, Vol. 16, No. 12, 2004, pp. 2442-2448. http://dx.doi.org/10.1021/cm0350232 [19] M. S. Meier and J. Kiegiel, “Preparation and Characteri- zation of the Fullerene Diols 1,2-C60(OH)2, 1,2-C70(OH)2, and 5,6-C70(OH)2,” Organic Letters pp. 1717-1719. , Vol. 3, No. 11, 2001 159120 , http://dx.doi.org/10.1021/ol0 [20] R. H. Lohwasser, J. Bandara and M. Thelakkat, “Tailor- Made Synthesis of Poly(3-hexylthiophene) with Carbox- ylic Endgroups and Its Application as a Polymer Sensi- tizer in Solid-State Dye-Sensitized Solar Cells,” Journal of Materials Chemistry, Vol. 19, 2009, pp. 4126-4130. http://dx.doi.org/10.1039/b900921c [21] J. U. Lee, A. Cirpan, T. Emrick, T. P. Russell and W. H. Jo, “Synthesis and Photophysical Property of Well-De- fined Donor-Acceptordiblock Copolymer Based on Re- gioregular Poly(3-hexylthiophene) and Fullerene,” Jour- nal of Materials Chemistry, Vol. 19, 2009, pp. 1483-1489. http://dx.doi.org/10.1039/b813368a [22] C. P. Chen, S. H. Chan, T. C. Chao, C. Ting and B. T. Ko, “Low-Bandgap Poly(Thiophene-Phenylene-Thiophene) De- rivatives with Broaden Absorption Spectra for Use in High-Performance Bulk-Heterojunction Polymer Solar Cells,” Journal of the American Chemical Society, Vol. 130, No. 38, 2008, pp. 12828-12833. http://dx.doi.org/10.1021/ja801877k [23] M. L. Blohm, J. E. Pikett and P. C. VanDort, “Synthesis, Characterization, and Stability of Poly(3,4-Dibutoxy- thiophenevinylene) Copolymers,” Macromolecules, Vol. 26, No. 11, 1993, pp. 2704-2710. http://dx.doi.org/10.1021/ma00063a011 [24] S. A. Chen and C. C. Tsai, “Structure/Properties of Con- jugated Conductive Poly thiophenes and Poly(4-Methylthiophenes),” Macrom mers. 2. 3-Ether-Substituted Poly- ole- cules, Vol. 26, No. 9, 1993, pp. 2234-2239. http://dx.doi.org/10.1021/ma00061a015 [25] G. Gigli, G. Barbarella, L. Favaretto, F. Cacialli and R. Cingolani, “High-Efficiency Oligothiopene-Based Light- Emitting Diodes,” Applied Physics Letters, Vol. 75, 1999, p. 439. http://dx.doi.org/10.1063/1.124403 [26] J. L. Bredas, R. Silbey, D. S. Boudreaux and R. R. Chance, “Chain-Length Dependence of Electronic and Electrochemical Properties of Conjugated Systems: Poly- /ja00360a004 acetylene, Polyphenylene, Polythiophene, and Polypyr- role,” Journal of the American Chemical Society, Vol. 105, No. 22, 1983, pp. 6555-6559. http://dx.doi.org/10.1021 wn and R. E. [27] D. M. de Leeuw, M. M. Simenon, A. R. Bro F. Einerhand, “Stability of N-Type Doped Conducting Polymers and Consequences for Polymeric Microelec- tronic Devices,” Synthetic Metals, Vol. 87, No. 1, 1997, pp.53-59. http://dx.doi.org/10.1016/S0379-6779(97)80097-5 [28] S. A. Ponomarenko, S. Kirchmeyer, A. Elschner, N. M. Alpatova, M. Halik, H. Klauk, U. Zschieschang and G. Schmid, “Decyl-End-Capped Thiophene-Phenylene Oli- gomers as Organic Semiconducting Materials with Im- proved Oxidation Stability,” Chemical Materials, Vol. 18, No. 2, 2006, pp. 579-586. http://dx.doi.org/10.1021/cm052210m [29] S. Mohapatra, B. T. Holmes, C. R. Newman, C. F. Pren- dergast, C. D. Frisbie and M. D. Ward, “Organic Thin- Film Transistors Based on Tolyl-Substituted Oligothio- phenes,” Advanced Functional Materials, Vol. 14, No. 6, 2004, pp. 605-609. http://dx.doi.org/10.1002/adfm.200400034 [30] Q. Zhang, J. Feng, K. Liu, D. Zhu, M. Yang, H. Ye and X. Liu, “Synthesis and Characterization of Novel Low Band Gap Polymers: Poly(heteroarylene methines),” Syntheti Metals, Vol. 156, No. 11-13, 2006, p c p. 804-808. http://dx.doi.org/10.1016/j.synthmet.2006.04.014 [31] G. Louarn, J. Y. Mevellec, J. P. Buisson and S. Lefrant, “Experimental Ant Theoretical Study of Vibrational Pro- perties of Polythiophène, Polyméthylthiophène and Po- lyoctylthiophène,” Journal de Chimie Physique, Vol. 89, 1992, p. 987. [32] J. F. Pan, S. J. Chua and W. Huang, “Conformational Analysis (ab Initio HF/3-21G*) and Optical Properties of Poly(thiophene-phenylene-thiophene) (PTPT),” Chemical Physics Letters, Vol. 363, No. 1-2, 2002, pp. 18-24. http://dx.doi.org/10.1016/S0009-2614(02)00934-X [33] J. Pei, W. L. Yu, W. Huang and A. J Series of Efficient Thiophene-Based . Heeger, “A Novel Light-Emitting Con- jugated Polymers and Application in Polymer Light- Emitting Diodes,” Macromolecules, Vol. 33, No. 7, 2000, pp. 2462-2471. http://dx.doi.org/10.1021/ma9914220 [34] F. Naso, F. Babudri, D. Colangiuli, G. M. Farinola, F. Quaranta, R. Rella, R. Tafuro and L. Valli, “Thin Film Construction and Characterization and Gas-Sensing Per- formances of a Tailored Phenylene-Thienylene Copoly- mer,” Journal of the American Chemical Society, Vol. 125, No. 30, 2003, pp. 9055-9061. http://dx.doi.org/10.1021/ja035490k [35] S. J. Toal and T. C. Williams, “Polymer Sensors for Ni- troaromatic Explosives Detection,” Journal of Materials Chemistry, Vol. 16, 2006, pp. 2871-2883. http://dx.doi.org/10.1039/b517953j [36] F. Meyer, A. J. Heeger and J. L. Bredas, “Fine Tuning of the Band Gap in Conjugated Polymers via Control of Block Copolymer Sequences,” The Journal of Chemical Physics, Vol. 97, No. 4, 1992, p. 2750. http://dx.doi.org/10.1063/1.463065 [37] M. A. Loi, E. J. W List, C. Gaderm Leising, G. Bongiovanni, A. Mura, J. aier, W. Graupner, G. J. Pireaux and K. Kaeriyama, “Optical Characterisation of Poly-2,5-diheptyl- 1,4-phenylene-alt-2,5-Thienylene,” Synthetic Metals, Vol. 111-112, 2000, pp. 519-522. http://dx.doi.org/10.1016/S0379-6779(99)00410-5 [38] R. A. J Janssen, L. Smilowitz, N. S. Sariciftci and D. Open Access IJOC
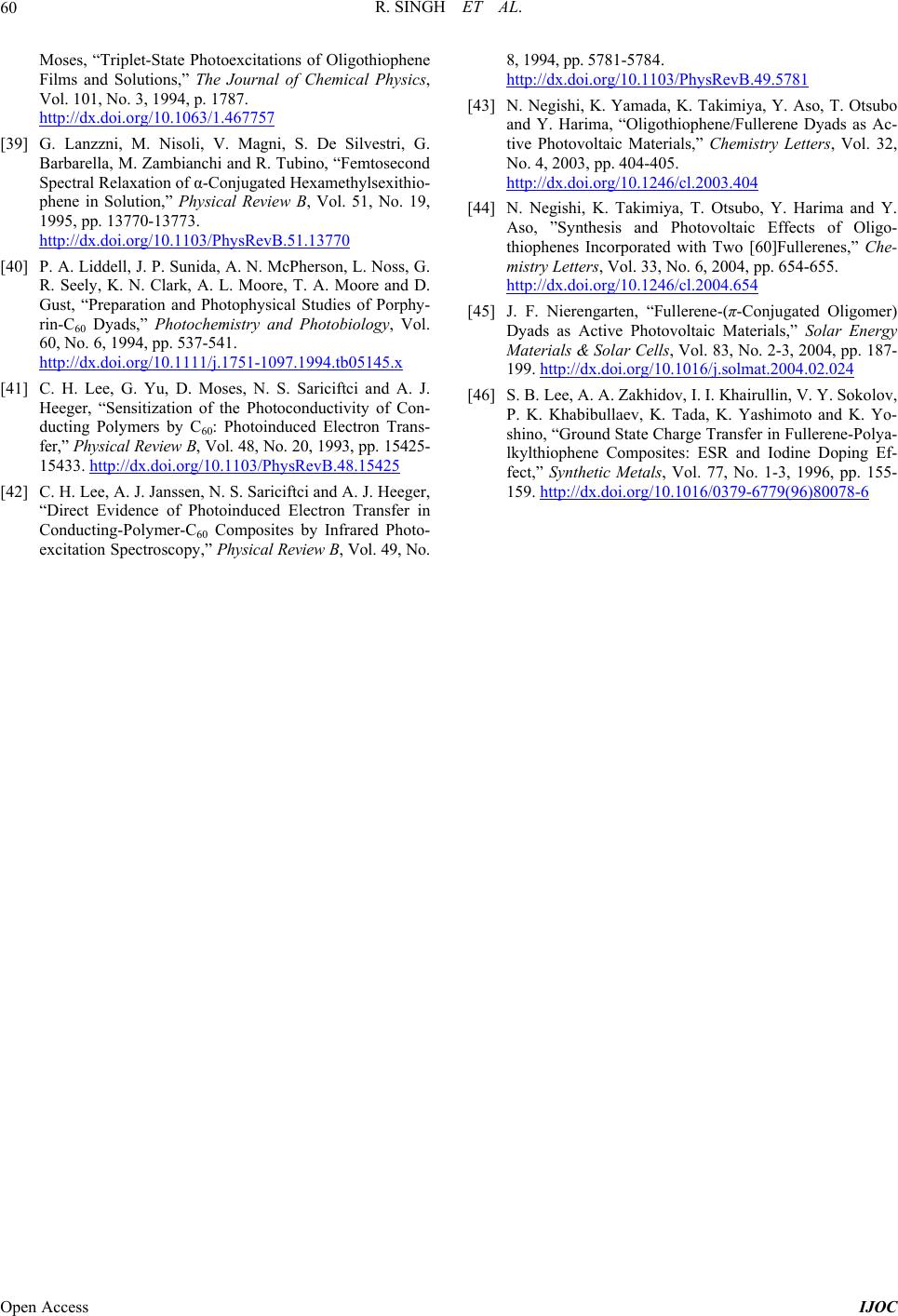 R. SINGH ET AL. Open Access IJOC 60 pheneMoses, “Triplet-State Photoexcitations of Oligothio Films and Solutions,” The Journal of Chemical Physics, Vol. 101, No. 3, 1994, p. 1787. http://dx.doi.org/10.1063/1.467757 [39] G. Lanzzni, M. Nisoli, V. Magni, S. De Silvestri, G. Barbarella, M. Zambianchi and R. Tubino, “Femtosecond Spectral Relaxation of α-Con phene in Solution,” Physical Review B jugated Hexamethylsexithio- , Vol. 51, No. 19, 1995, pp. 13770-13773. http://dx.doi.org/10.1103/PhysRevB.51.13770 [40] P. A. Liddell, J. P. Sunida, A. N. McPherson, L. Noss, G. R. Seely, K. N. Clark, A. L. Moore, T. A. Moore and D. Gust, “Preparation an rin-C60 Dyads,” Photochemistry and Photobiolo d Photophysical Studies of Porphy- gy, Vol. 60, No. 6, 1994, pp. 537-541. http://dx.doi.org/10.1111/j.1751-1097.1994.tb05145.x [41] C. H. Lee, G. Yu, D. Moses, N. S. Sariciftci and A. J. Heeger, “Sensitization of the Photoconductivity of Con- ducting Polymers by C60: Photoinduced Electron Trans- fer,” Physical Review B, Vol. 48, No. 20, 1993, pp. 15425- 15433. http://dx.doi.org/10.1103/PhysRevB.48.15425 [42] C. H. Lee, A. J. Janssen, N. S. Sariciftci and A. J. Heeger, “Direct Evidence of Photoinduced Electron Transfer in Conducting-Polymer-C60 Composites by Infrared Photo- excitation Spectroscopy,” Physical Review B, Vol. 49, No. 8, 1994, pp. 5781-5784. http://dx.doi.org/10.1103/PhysRevB.49.5781 tsubo .404 [43] N. Negishi, K. Yamada, K. Takimiya, Y. Aso, T. O and Y. Harima, “Oligothiophene/Fullerene Dyads as Ac- tive Photovoltaic Materials,” Chemistry Letters, Vol. 32, No. 4, 2003, pp. 404-405. http://dx.doi.org/10.1246/cl.2003 o, Y. Harima and Y. [44] N. Negishi, K. Takimiya, T. Otsub Aso, ”Synthesis and Photovoltaic Effects of Oligo- thiophenes Incorporated with Two [60]Fullerenes,” Che- mistry Letters, Vol. 33, No. 6, 2004, pp. 654-655. http://dx.doi.org/10.1246/cl.2004.654 [45] J. F. Nierengarten, “Fullerene-(π-Conjugated O Dyads as Active Photovoltaic Materials,” Sol ligomer) ar Energy Materials & Solar Cells, Vol. 83, No. 2-3, 2004, pp. 187- 199. http://dx.doi.org/10.1016/j.solmat.2004.02.024 [46] S. B. Lee, A. A. Zakhidov, I. I. Khairullin, V. Y. Sokolov, P. K. Khabibullaev, K. Tada, K. Yashimoto and K. Yo- shino, “Ground State Charge Transfer in Fullerene-Poly lkylthiophene Composites: ESR and Iodine Doping E a- f- fect,” Synthetic Metals, Vol. 77, No. 1-3, 1996, pp. 155- 159. http://dx.doi.org/10.1016/0379-6779(96)80078-6
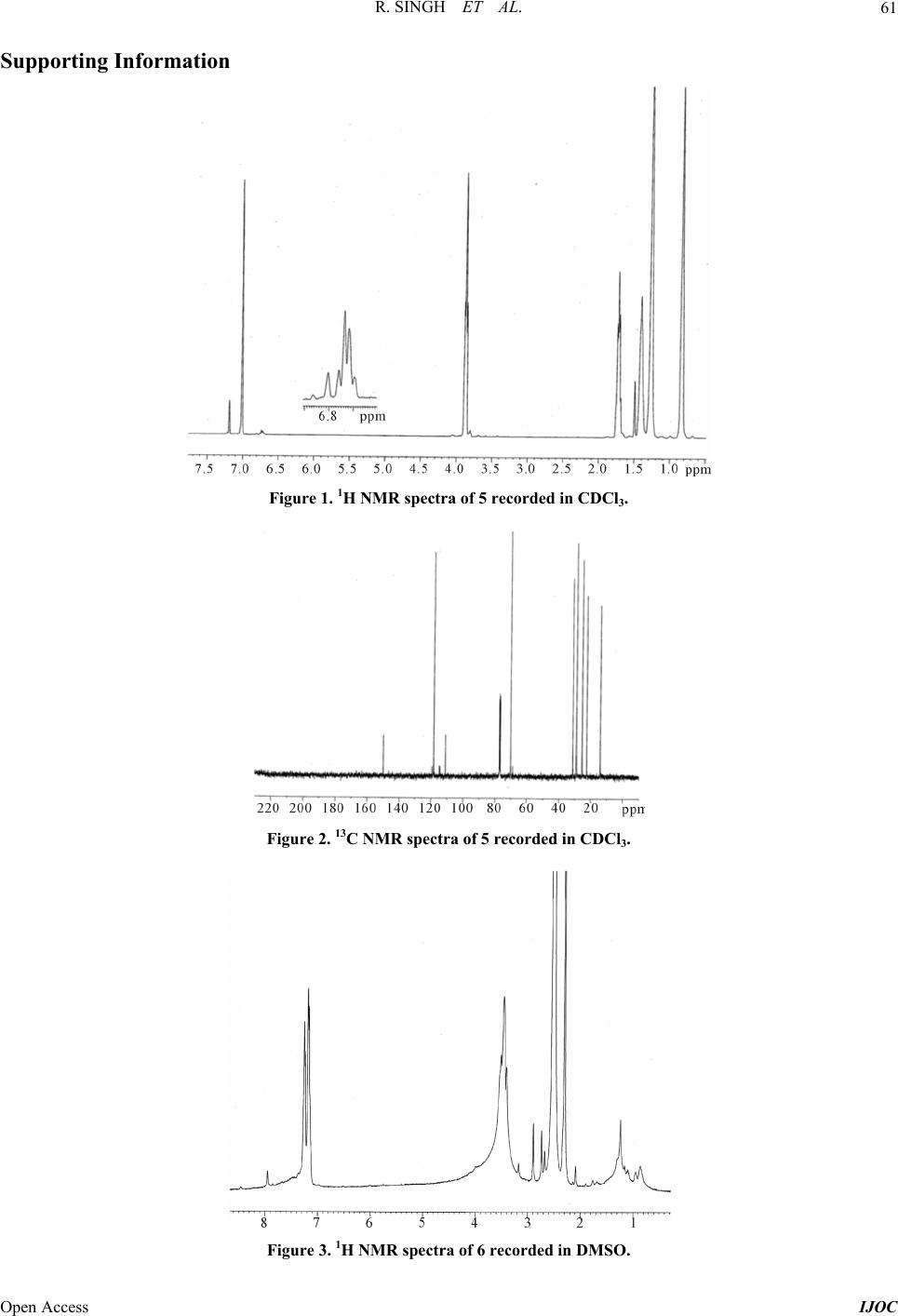 R. SINGH ET AL. 61 Supporting Information Figure 1. 1H NMR spectra of 5 recorded in CDCl3. Figure 2. 13C NMR spectra of 5 recorded in CDCl3. Figure 3. 1H NMR spectra of 6 recorded in DMSO. Open Access IJOC
 R. SINGH ET AL. 62 Figure 4. 13C NMR spectra of 6 recorded in DMSO. Figure 5. FTIR spectra of 5. Figure 6. FTIR spectra of 6. Open Access IJOC
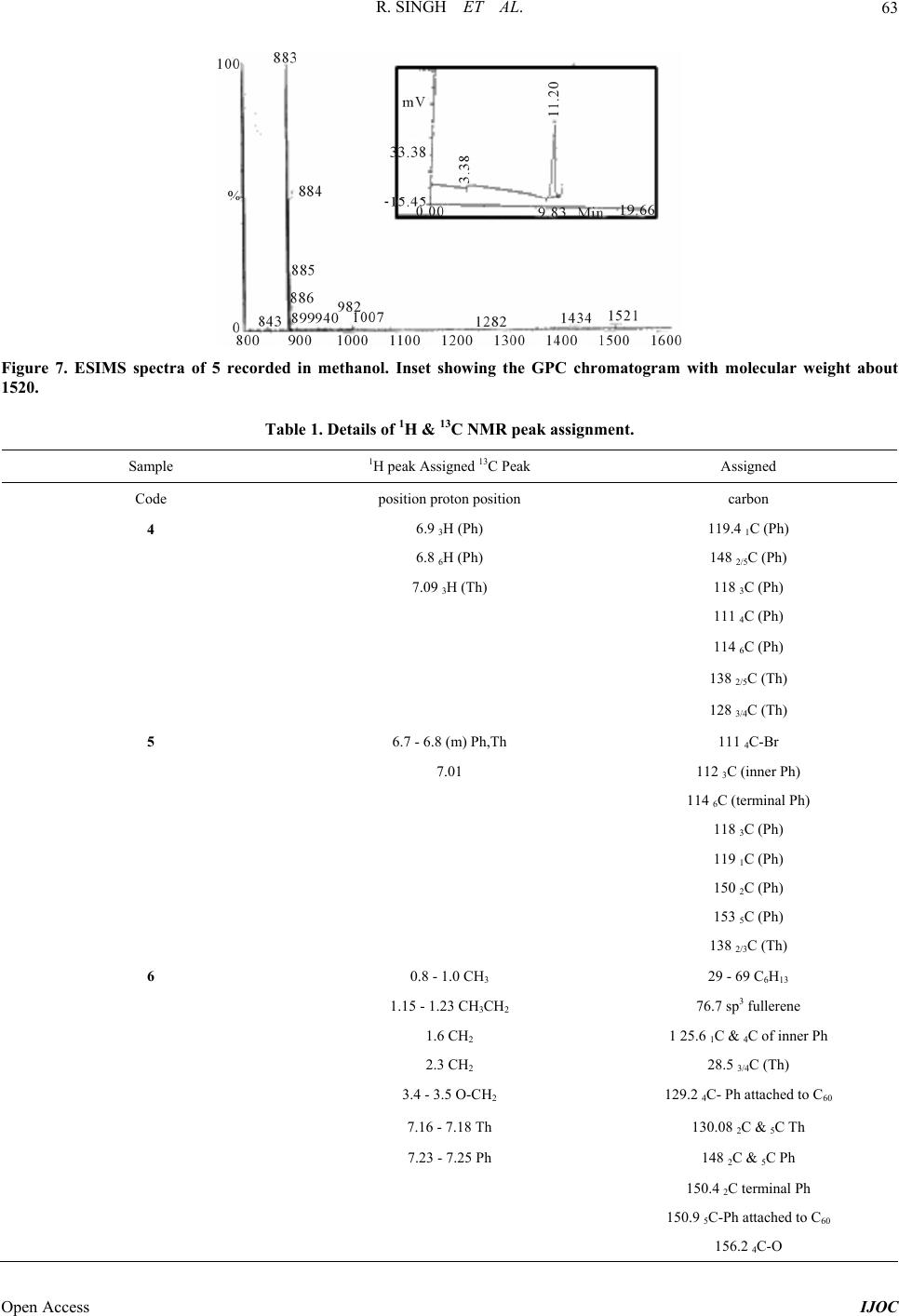 R. SINGH ET AL. 63 Figure 7. ESIMS spectra of 5 recorded in methanol. Inset showing the GPC chromatogram with molecular weight about 1520. Table 1. Details of 1H & 13C NMR peak assignment. Sample 1H peak Assigned 13C Peak Assigned Code position proton position carbon 4 6.9 3H (Ph) 119.4 1C (Ph) 6.8 6H (Ph) 148 2/5C (Ph) 7.09 3H (Th) 118 3C (Ph) 111 4C (Ph) 114 6C (Ph) 138 2/5C (Th 128 3/4C (Th 5 6.7 - 6.8 (m) Ph,Th 111 4C-Br 7.01 112 3C (inner Ph) 114 6C (terminal Ph) 118 3C (Ph) 119 1C (Ph) 150 2C (Ph) 153 5C (Ph) 138 2/3C (Th) 6 0.8 - 1.0 CH3 29 - 69 C6H13 1.15 - 1.23 CH3CH2 76.7 sp3 fullerene 1.6 CH2 1 25.6 1C & 4C of inner Ph 2.3 CH2 28.5 3/4C (Th 3.4 - 3.5 O-CH2 129.2 4C- Ph attached to C60 7.16 - 7.18 Th 130.08 2C & 5C Th 7.23 - 7.25 Ph 148 2C & 5C Ph 150.4 2C terminal Ph 150.9 5C-Ph attached to C60 156.2 4C-O Open Access IJOC
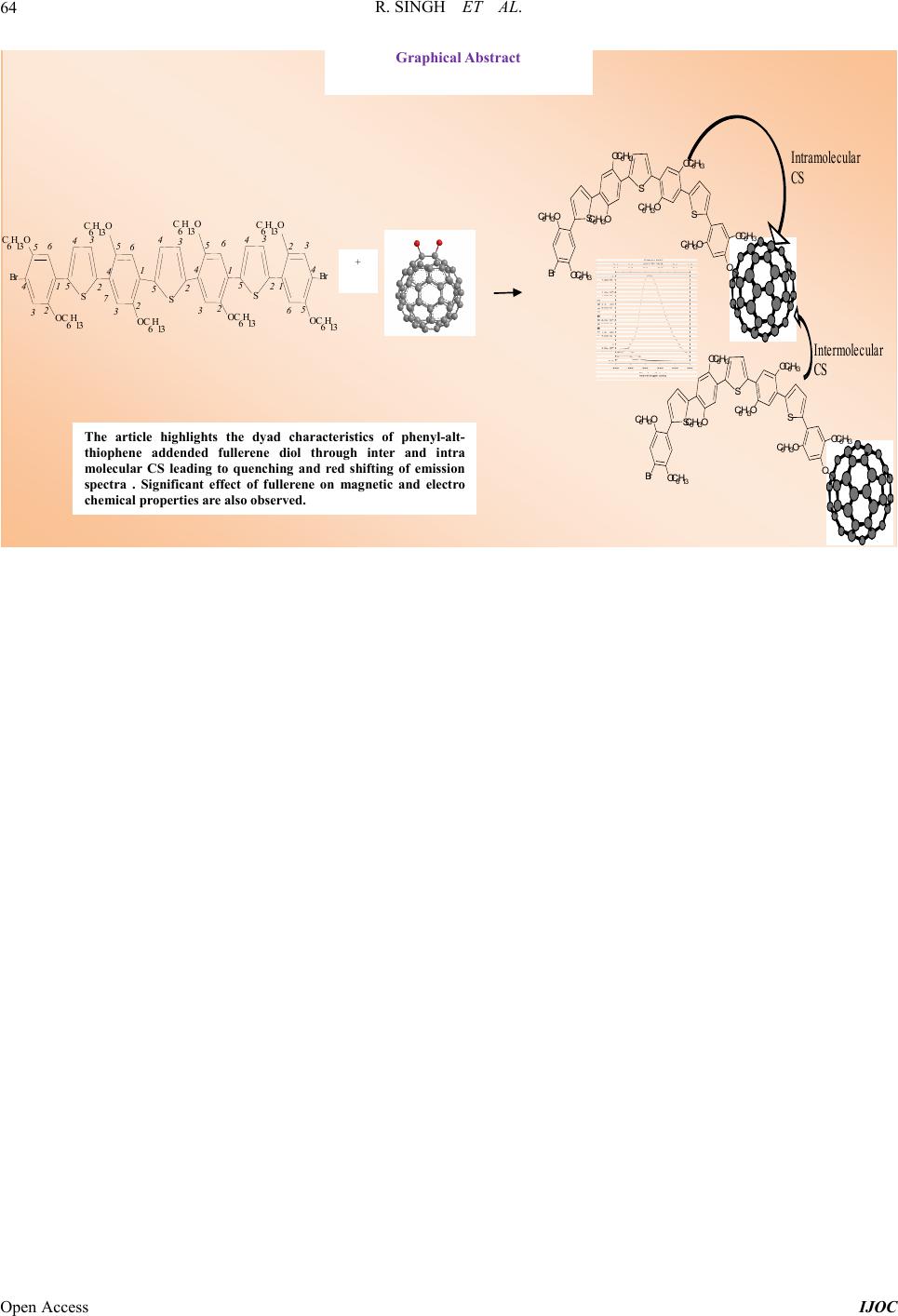 R. SINGH ET AL. Open Access IJOC 64 Graphical Abstract S C 6H 1 3O O C 6H 13 B r 1 4 3 5 2 3 4 5 O C 6H 13 C 6H 13 O S C 6 H 1 3O OC 6H 13 B r 4 5 1 23 2 3 4 5 O C 6H 13 C 6 H 13O S 3 1 4 5 2 2 4 32 1 5 5 2 43 + S OC 6 H 13 C 6 H 13O O C 6 H 13O OC 6 H 13 S OC 6 H 13 C 6 H 13O Br OC 6 H 13 C 6 H 13O S S OC 6 H 1 C 6 H 13O O C 6 H 13O OC 6 H 13 S OC 6 H 13 C 6 H 13O Br OC 6 H 13 C 6 H 13O S Intramolecular CS Intermolecular CS The article highlights the dyad characteristics of phenyl-alt- thiophene addended fullerene diol through inter and intra molecular CS leading to quenching and red shifting of emission spectra . Significant effect of fullerene on magnetic and electro chemical properties are also observed.
|