 Vol.4, No.11A, 1-11 (2013) Agricultural Sciences http://dx.doi.org/10.4236/as.2013.411A001 Histological analysis and transcription profiles on somatic embryogenesis in interspecific hybrids of Elaeis guineensis × E. oleifera# Paula Cristina da Silva Angelo1*, Douglas André Steinmacher2,3, Ricardo Lopes1, Raimundo Nonato Vieira da Cunha1, Miguel Pedro Guerra2,3 1Embrapa Western Amazon, Manaus, Brazil; *Corresponding Author: paula.angelo@embrapa.br 2UFSC, Federal University of Santa Catarina—CCA, Graduate Program in Plant Genetic Resources, Physiology of Development and Plant Genetics Laboratory, Florianópolis, Brazil 3Brazilian Council for Technological Development—CNPq, Brazil Received 23 July 2013; revised 29 August 2013; accepted 19 September 2013 Copyright © 2013 Paula Cristina da Silva Angelo et al. This is an open access article distributed under the Creative Commons Attri- bution License, which permits unrestricted use, distribution, and reproduction in any medium, provided the original work is properly cited. ABSTRACT Elaeis guineensis (African oil palm) and E. oleife- ra (American oil palm) are bred to attain high oil yields, disease resistances, and decelerated shoot elongation. We cultivated immature zy- gotic embryos from backcrossed and F1 inter- specific progenies on media cont aining 110, 150, or 200 mg·l−1 2,4-diclorophenoxyacetic acid (2,4- D) to obtain embryogenic cultures. These were set to multiply on medium containing 8 mg·l−1 2,4-D or lower concentrations of auxins and fi- nally we induced plantlets regeneration, from each zygotic embryo, independently, in order to have the clones organized according to their respective origins. Reductions in auxins in- duced cultures to revert from highly embryo- genic into competent for embryogenesis and finally to organogenic degenerated callus lines. Histology and the expression of SOMATIC EM- BRYOGENESIS RECEPTOR KINASE, DEHYDRIN, DEFENSIN, TRANSPOSASE, and LIPID TRANS- FER PROTEIN were analy zed on four callus lines representative of morphological aspects con- sistently observed. The highest number of em- bryogenic cultures was obtained on 150 mg·l−1 2,4-D. Maturation and multiplication of somatic embryos through secondary embryogenesis occurred simultaneously on 8 mg·l−1 2,4-D. LIPID TRANSFER PROTEIN expression was detected in one of the embryogenic cultures and corre- lated with protoderm onset. Three six-week cy- cles on induction medium yielded 1.5 shoots above 6 cm per poly-embryogenic complex, which performed better than embryoids indi- vidualized mechanically. Rooting was observed for 77% and 82% of shoots from these two types of explants, respectively. Rooted plantlets ready for acclimatization were obtained nine months after shoot induct ion had started. Keywords: Amazon; Arecaceae; Dend ê; Caiaué; Gene Expression 1. INTRODUCTION Elaeis guineensis (Jacq.) tp. tenera cultivars are con- sidered the best producers of oil, which is used in food, biofuels and cosmetics [1-3]. In Latin America, tenera plantations are harmed by the lethal yellowing anomaly. However, it does not eliminate F1 hybrids between E. guineensis and the Amazon native species, E. oleifera (Kunth) Cortés, growing in the same impacted areas. In addition, E. oleifera has a lower rate of annual shoot growth and a higher content of unsaturated fatty-acids [4]. To maintain high yields, guineens is × oleifera hy- brids are backcrossed with E. guineensis [4], and the progenies resulting from this complex breeding strategy present variability. Cloning can facilitate comparison among plants grown in different experimental areas. A few thousand clones for each progeny—tens of clones #Part of the Pos-Doctoral activities of the first author. Cite this paper: Silva Angelo, P., Steinmacher, D., Lopes, R., Vieira da Cunha, R. and Guerra, M. (2013) Histological analysis and transcrip- tion profiles on somatic embryogenesis in interspecific hybrids of Ela- eis guineensis × E. oleifera. Agricultural Sciences, 4, 1-11. http://dx.doi.org/10.4236/as.2013.411A001 Copyright © 2013 SciRes. OPEN A CCESS
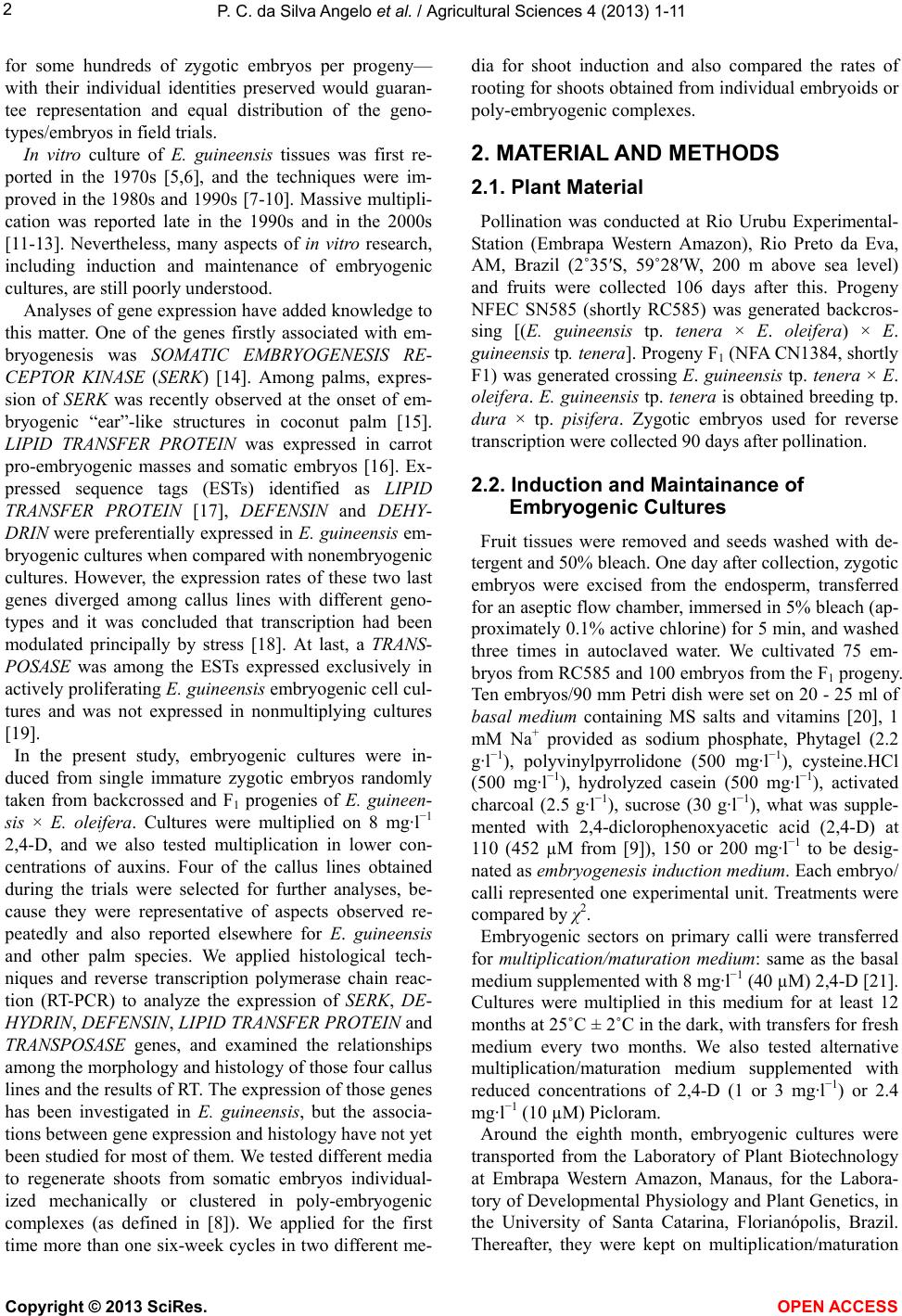 P. C. da Silva Angelo et al. / Agricultural Sciences 4 (2013) 1-11 2 for some hundreds of zygotic embryos per progeny— with their individual identities preserved would guaran- tee representation and equal distribution of the geno- types/embryos in field trials. In vitro culture of E. guineensis tissues was first re- ported in the 1970s [5,6], and the techniques were im- proved in the 1980s and 1990s [7-10]. Massive multipli- cation was reported late in the 1990s and in the 2000s [11-13]. Nevertheless, many aspects of in vitro research, including induction and maintenance of embryogenic cultures, are still poorly understood. Analyses of gene expression have added knowledge to this matter. One of the genes firstly associated with em- bryogenesis was SOMATIC EMBRYOGENESIS RE- CEPTOR KINASE (SERK) [14]. Among palms, expres- sion of SERK was recently observed at the onset of em- bryogenic “ear”-like structures in coconut palm [15]. LIPID TRANSFER PROTEIN was expressed in carrot pro-embryogenic masses and somatic embryos [16]. Ex- pressed sequence tags (ESTs) identified as LIPID TRANSFER PROTEIN [17], DEFENSIN and DEHY- DRIN were preferentially expressed in E. guineensis em- bryogenic cultures when compared with nonembryogenic cultures. However, the expression rates of these two last genes diverged among callus lines with different geno- types and it was concluded that transcription had been modulated principally by stress [18]. At last, a TRANS- POSASE was among the ESTs expressed exclusively in actively proliferating E. guineensis embryogenic cell cul- tures and was not expressed in nonmultiplying cultures [19]. In the present study, embryogenic cultures were in- duced from single immature zygotic embryos randomly taken from backcrossed and F1 progenies of E. guineen- sis × E. oleifera. Cultures were multiplied on 8 mg·l−1 2,4-D, and we also tested multiplication in lower con- centrations of auxins. Four of the callus lines obtained during the trials were selected for further analyses, be- cause they were representative of aspects observed re- peatedly and also reported elsewhere for E. guineensis and other palm species. We applied histological tech- niques and reverse transcription polymerase chain reac- tion (RT-PCR) to analyze the expression of SERK, DE- HYDRIN, DEFENSIN, LIPID TRANSFER PROTEIN and TRANSPOSASE genes, and examined the relationships among the morphology and histology of those four callus lines and the results of RT. The expression of those genes has been investigated in E. guineensis, but the associa- tions between gene expression and histology have not yet been studied for most of them. We tested different media to regenerate shoots from somatic embryos individual- ized mechanically or clustered in poly-embryogenic complexes (as defined in [8]). We applied for the first time more than one six-week cycles in two different me- dia for shoot induction and also compared the rates of rooting for shoots obtained from individual embryoids or poly-embryogenic complexes. 2. MATERIAL AND METHODS 2.1. Plant Material Pollination was conducted at Rio Urubu Experimental- Station (Embrapa Western Amazon), Rio Preto da Eva, AM, Brazil (2˚35ʹS, 59˚28ʹW, 200 m above sea level) and fruits were collected 106 days after this. Progeny NFEC SN585 (shortly RC585) was generated backcros- sing [(E. guineensis tp. tenera × E. oleifera) × E. guineensis tp. tenera]. Progeny F1 (NFA CN1384, shortly F1) was generated crossing E. guineensis tp. tenera × E. oleifera. E. guineensis tp. tenera is obtained breeding tp. dura × tp. pisifera. Zygotic embryos used for reverse transcription were collected 90 days after pollination. 2.2. Induction and Maintainance of Embryogenic Cultures Fruit tissues were removed and seeds washed with de- tergent and 50% bleach. One day after collection, zygotic embryos were excised from the endosperm, transferred for an aseptic flow chamber, immersed in 5% bleach (ap- proximately 0.1% active chlorine) for 5 min, and washed three times in autoclaved water. We cultivated 75 em- bryos from RC585 and 100 embryos from the F1 progeny. Ten embryos/90 mm Petri dish were set on 20 - 25 ml of basal medium containing MS salts and vitamins [20], 1 mM Na+ provided as sodium phosphate, Phytagel (2.2 g·l−1), polyvinylpyrrolidone (500 mg·l−1), cysteine.HCl (500 mg·l−1), hydrolyzed casein (500 mg·l−1), activated charcoal (2.5 g·l−1), sucrose (30 g·l−1), what was supple- mented with 2,4-diclorophenoxyacetic acid (2,4-D) at 110 (452 µM from [9]), 150 or 200 mg·l−1 to be desig- nated as embryogenesis induction medium. Each embryo/ calli represented one experimental unit. Treatments were compared by χ2. Embryogenic sectors on primary calli were transferred for multiplication/maturation medium: same as the basal medium supplemented with 8 mg·l−1 (40 µM) 2,4-D [21]. Cultures were multiplied in this medium for at least 12 months at 25˚C ± 2˚C in the dark, with transfers for fresh medium every two months. We also tested alternative multiplication/maturation medium supplemented with reduced concentrations of 2,4-D (1 or 3 mg·l−1) or 2.4 mg· l −1 (10 µM) Picloram. Around the eighth month, embryogenic cultures were transported from the Laboratory of Plant Biotechnology at Embrapa Western Amazon, Manaus, for the Labora- tory of Developmental Physiology and Plant Genetics, in the University of Santa Catarina, Florianópolis, Brazil. Thereafter, they were kept on multiplication/maturation Copyright © 2013 SciRes. OPEN A CCESS
 P. C. da Silva Angelo et al. / Agricultural Sciences 4 (2013) 1-11 3 medium modified to contain vitamins described in [22] instead of those from [20] plus glutamine (1 g·l-1) and with no cysteine. 2.3. Origin and Morphology of Representative Callus Lines The callus lines designated as 509, and 511A and 511B were raised from single zygotic embryos designated as 509 and as 511, respectively, from progeny RC585. The callus line designated as 002 was raised from zygotic embryo 002 from progeny F1. Callus line 511A was a derivative of the highly em- bryogenic culture 511. Following 14 months on mul- tiplication/maturation medium containing 8 mg·l−1 2,4-D, part of that culture was transferred for 1 mg·l−1 2,4-D in basal medium free of charcoal. Two months after this, we observed the reversion for calli bear- ing “ear”-like embryogenic structures (as defined in [15]), which were transferred to 3 mg·l−1 2,4-D for additional two months. Callus lines 509 and 002 were highly embryogenic cultures collected from multiplication/maturation me- dium supplemented with 8 mg·l−1 2,4-D 18 months after the introduction of zygotic embryos in vitro. Callus line 511B was obtained by transferring “ear”- like embryogenic structures excised from 511A for 2.4 mg·l−1 Picloram in basal medium free of charcoal [23] for two months followed by additional three months on basal medium free of growth regulators and charcoal. Immediately after registration of the morphological aspects through high definition digital images, biological material (calli and/or more friable cultures) was organ- ized as individual 100 mg samples, immediately frozen in liquid nitrogen and preserved at −80˚C. 2.4. Histological Analysis Samples from callus lines 511A, 509, 002, and 511B were defrosted overnight at 8˚C inside 3% paraformal- dehyde in 0.1 M phosphate buffer (pH 6.8). The material went through a dehydration series (30 to 96.5˚ ethanol) and was embedded in historesin (Leica) according to the instructions of the manufacturer. Tissues were sliced in 8 - 10 µm sections and stained with 0.05% toluidine blue in 0.1 M phosphate buffer (pH 6.8) for analyses using an Olympus light microscope/documentation system. 2.5. Transcription Profiles Total RNA was extracted from individual samples of 100 mg following disruption in a tissue lyser (Precellys) using the RNeasy Plant Mini kit (QIAGEN). The number of samples extracted from each callus line (511A, 509, 002 and 511B) was defined by the amount of RNA ob- tained from each one of them. The sum of the aliquots should reach approximately 13 µg to allow replication. RNA was treated with DNase I, purified in RNeasy Mini Spin columns (QIAGEN), quantified by spectrophotome- try (Nanodrop) and evaluated through denaturing agarose gel electrophoresis. Extraction was repeated from 90 days old immature zygotic embryos as well. RNA aliquots of 4 µg (biological replicas) from callus lines or 2 µg from immature zygotic embryos were used for 20 µl standard reactions of first strand polymerization with the Long Range 2-Step RT-PCR kit and oligo-dT primers (QIAGEN). From those, 3 µl were used for sec- ond-step PCR amplification reactions (technical repli- cates) using specific primers for SERK (5’-GGCGTCGT CGTAAGCCGCAAG-3’ and 5’-TGGCCAAGCCAAAG TCTCCAACA-3’), designed from conserved regions in the coconut SERK mRNA (GI90891655). Primers for DEFENSIN (5’-TGGGGACGAAGGTGGCGGA-3’ and 5’-AAAGCATCGGCGCCGGACT-3’), DEHYDRIN (5’- GACGAGTACGGCAACCCGATC-3’ and 5’-CTTTTCC ATCATCCCCTTCTT-3’) and TRANSLATION ELON- GATION FACTOR 1, the internal reference gene (5’-GGTGTGAAGCAGATGATTTGC-3’ and 5’-CCTG GATCATGTCAAGAGCC-3’) were reported in [24]. Primers for the LIPID TRANSFER PROTEIN (5’-TG- GAGAAAGGTGCACCGAAGCCGT-3’ and 5’-CCGG- CCAGTCTTACAGCCTGCACA-3’) and the TRANS- POSASE (5’-GCCTTGCTAGATCTAGGAGCTA GT-3’ and 5’-CCGTAGCTAAGAATGGTCTTCCT-3’) were designed for conserved regions in corresponding E. guineensis ESTs (GI193220058/DW248433 and GI221144290, respectively). All the cDNA amplicons were produced simultaneously using a step-down proce- dure [25] of −0.8˚C·s−1 from 78˚C to 54˚C for annealing in the first 13 PCR cycles. Anneling temperature was fixed in 54˚C for the next 32 cycles. Synthesis was fixed in 68˚C for 1 minute and denaturation was fixed in 93˚C for 10 seconds. Amplicons were stained with GelRed (Biotium), resolved in 1.5% agarose gels and exposed to UV illumination. The images (TIF) were used to calcu- late the calibrated quantity of fluorescence using linear regression equations developed with Quantity One 4.6 (BioRad) taking the ELONGATION FACTOR 1 as cali- brator. Reverse transcription (with three biological repli- cas), cDNA amplification, electrophoresis and quantifi- cation (in three technical replicas) were performed for each callus line. The identity of the amplicons was con- firmed by the proper size in electrophoresis, sequencing (MegaBace) and, for the LIPID TRANSFER PROTEIN, by amplification using nested (5’-TGCTGCAGTGACC TGACACGGGCT-3’ and 5’-TCGCAGGGGCTAAGAG CGTCGGA-3’) primers. The mean values for the cali- brated quantity of fluorescence for the five amplicons in Copyright © 2013 SciRes. OPEN A CCESS
 P. C. da Silva Angelo et al. / Agricultural Sciences 4 (2013) 1-11 4 the four callus lines were compared using ANOVA and Tukey tests. 2.6. Plantlet Regeneration For shoot induction, we used nondisrupted poly-em- bryogenic complexes (156 explants) or mechanically in- dividualized pear-shaped somatic embryos (180 explants) taken from callus lines 509 and 002. Cultivation was conducted on basal medium free of casein, glutamine, and charcoal, supplemented with 0.56 mg·l−1 (3 µM) naphthaleneacetic acid (NAA) and 2.25 mg·l−1 (10 µM) 6-benzyladenine (BA) [6] that was designated as NB, or with 0.08 mg·l−1 (0.44 µM) NAA and 5.00 mg·l−1 (24.6 µM) 2-isopentenyladenine (2-iP) [26], designated as NI. Explants were exposed to 16 h of photoperiod under 40 - 50 μmol·m−2·s−1 irradiance. Poly-embryogenic com- plexes were submitted to three cycles of shoot induction, six weeks long each. Comparison between percentages of leaf-like structures obtained from each type of explant was performed only at the end of the first cycle because individualized embryos were not submitted to more than one cycle of shoot induction. Shoots were allowed to reach 6 cm on in half-strength basal medium supple- mented with 30 g·l−1 sucrose before mechanical indi- vidualization for rooting. Rooting was tested on 76 shoots from poly-embryo- genic complexes and on 38 shoots from mechanically individualized somatic embryos, in basal medium free of casein or glutamine, containing half the concentration of iron, supplemented with 30 or 60 g·l−1 sucrose [6] and NAA (11.1 mg·l−1 or 16.7 mg·l−1). Explants were culti- vated on 50 ml medium/500 ml flask or 15 ml medium/ 22.5 × 2.5 cm culture tubes. Treatments were under. All the statistical analyses (χ2 tests, Z tests, ANOVA and Tukey tests) were performed using SigmaPlot, ver- sion 11.2, P ≤ 0.05. 3. RESULTS 3.1. Induction of Embryogenesis E. guineensis × E. oleifera immature zygotic embryos were maintained for more than 12 months on induction medium, because embryogenesis in palms often depends on long-term cultivation [27,28]. By the sixth month, 20% and 39% of the cultures from F1 progenies and RC585, respectively, were active and responsive to changes in the cultivation conditions. The explants were primary compact calli or calli that had given raise to em- bryogenic cultures, which were first observed in the second month (Table 1). Up to this point, discards were caused by oxidation, hardening, rooting, or contamina- tion (around 5%), in this order. The use of 150 mg·l−1 2,4-D was valuable in inducing embryogenesis for both Table 1. Classification of calli induced on immature zygotic embryos from two progenies of Elaeis guineensis × E. oleifera, six months after inoculation on induction media supplemented with different concentrations of 2,4-D. Calli (%) Progeny n 2,4-D (mg·l−1) Primary Embryogenic 110 25.0 5.0 150 45.0 15.0 F1 100 200 5.0 5.0 110 31.0 3.4 150 17.3 24.1 RC58575 200 20.7 3.5 progenies but it was significantly superior for RC585 (χ2 = 7.16; P = 0.0278). 3.2. Morphology and Histology of Representative Callus Lines Callus line 511A derived from 511 following a reduc- tion in 2,4-D concentration. Two months were enough for the reversion of the cultures from friable and highly embryogenic back to compact calli displaying superficial “ear”-like embryogenic structures (Figure 1A). This structures had already been observed during the transi- tion from primary to embryogenic calli, in the first months of in vitro cultivation, more than a year before. The highly embryogenic features of 511 was not main- tained on 1 mg·l−1 2,4-D or recovered on 3 mg·l−1 2,4-D, but we could still observe displacement of internal mer- istematic cores for the surface of the embryogenic struc- tures, where segmentation and spatial reorientation of the external cell layers took place (Figure 1B). Simultane- ously, there was the extrusion of tiny pro-embryos with a small number of cells (Figure 1C), which would possi- bly maturate and emerge through or detach from the sur- face of the calli (Figure 1D). Lines 509 (Figure 1E) and 002 (Figure 1I) diverged in important aspects by the time of collection despite both were highly embryogenic. Somatic embryos in cal- lus line 509 erupted in clusters from a compact basal callus. Some of them were intermediary, translucent and elongating (Figure 1E, arrow), with protoderm ongoing differentiation (Figure 1F, arrows). This phase of de- velopment was observed solely in this callus line. Other sectors in the same calli and culture produced pear- shaped somatic embryos with procambium and periderm, which entered secondary embryogenesis, characterized by the arising of multiple clumps of meristematic cells at the junctions to the basal callus (Figure 1H). In contrast, line 002 from F1 hybrids had friable, pearly colored sectors (Figure 1I, arrow) that were finely Copyright © 2013 SciRes. OPEN A CCESS
 P. C. da Silva Angelo et al. / Agricultural Sciences 4 (2013) 1-11 5 fragmented (Figure 1J), and multiplied fast. This char- acteristic was exclusive of this callus line. In comparison to 509, by the time they were collected for histological and reverse transcription analyses, 002 displayed fewer sectors of fully developed/clustered somatic embryos on compact basal calli. Callus line 511B was raised from “ear”-like embryo- genic structures excised from 511A, cultivated in re- duced auxin concentration (2.4 mg·l−1 Picloram) and in absence of growth regulators. Concomitant with reduce- tions in auxin concentration inflicted to 511 (from 110 for 8 mg·l−1), again to 511A (from 8 to 3 mg·l−1) and fi- nally to 511B (as stated above) we observed the con- tinuous degeneration of a highly embryogenic culture until reach the sectored, hairy, calli displaying root-like structures and embryogenic sectors, producing a few peripheral yellowish pear-shaped somatic embryos (Fig- ure 1M). These would be associated with residual mer- istem segmentation (Figure 1N). Xylem elements pre- senting secondary wall deposition (Figure 1P) were pre- sent but no organized vascular bundle was observed. As stated for 511A, lines 509 and 002 also produced very small isolated pro-embryos, several of them ob- served as four-celled structures in the histological sec- tions, surrounded by extra-cellular matrix and thickened outer walls (Figures 1D, G and K). These barriers would promote the isolation of pro-embryos in coconut and date palm [28,29] and contribute to maturation. A thickened surrounding matrix can be observed surrounding larger portions of cells which are presumably in course of or- ganization to differentiate in somatic embryos in callus line 002 as well (Figures 1J and L). This process was not observed in callus line 509. It is part of the difference between 509 and 002 with possible repercussions to the origin of the embryos coming from each one of these callus lines: from big compact basal callus in 509 and from more friable meristematic cores in 002. 3.3. Transcription Profiles We detected the expression of the targeted genes through the presence of cDNA amplicons of expected sizes. Except for the LIPID TRANSFER PROTEIN, which was observed only in callus line 509 (Figure 2A, LT), amplicons coming from the other genes were pre- sent in all the callus lines and also in immature zygotic embryos (Figure 2B). There was no statistically signifi- cant difference among callus lines (Tukey tests, P > 0.05) regarding the mean values of calibrated quantity of fluo- rescence recorded for SERK, DEHYDRIN, DEFENSIN or TRANSPOSASE transcripts. The highest quantity of fluorescence was recorded for DEHYDRIN, followed by DEFENSIN, TRANSPOSASE, SERK and finally LIPID TRANSFER PROTEIN in callus line 509, which was ap- proximately half that of SERK (Figure 2C). We obtained good quality sequence reads covering 432 of the 579 base pairs (bp) expected for the SERK amplicon, including the catalytic domain of the corre- spondent enzyme. When that read was used to screen the GenBank, the closest match [E−value = 5e−143 and 91% (368/408) identity] was to a Cocos nucifera SERK mRNA (AY791293.2/GI 90891655), as expected since at that moment there were no entries designated as SERK from Elaeis in the nonredundant nucleotide collection. The similarity among sequences from different palm species had already been reported [24]. Accordingly, the next best hit was a SERK from Areca catechu (GU584945.1), another Arecaceae. The third and fourth closest matches were, respectively, to a not yet named mRNA and to an mRNA for a SERK-FAMILY RECEPTOR-LIKE KINASE (AB188247.1), both from Oryza sativa, which is a monocot. SERK primers also prompted the amplification of a 550 bp band (Figures 2A and B), which we could not isolate and was consid- ered an artifact of the PCR. The sequenced portion of the cDNA amplicon corre- sponding to the LIPID TRANSFER PROTEIN was 80% identical to the E. guineensis DW248433 (GI 193220058) mentioned in [17]. The reads covered a domain (cd- 00010-AAI/LTSS) conserved in proteins involved in plant defense against insects and pathogens, lipid trans- port between intracellular membranes and, nutrient stor- age. This same domain is present in the polypeptide de- duced from DW248433 when translated in frame +1 from the first ATG codon. The amplicon produced by nested primers had around 156 bp (result not shown). The nucleotide sequences obtained for DEHYDRIN, DEFENSIN and TRANSPOSASE were around 97% simi- lar to those published for E. guineensis. 3.4. Plantlet Regeneration Haustorium-like structures were not included in Table 2, despite the presence of chlorophyll. The two types of explants—poly-embryogenic complexes and somatic em- bryos individualized mechanically from the complexes Table 2. Percentage of Elaeis guineensis × E. oleifera indi- vidualized somatic embryoids (ISE) and poly-embryogenic complexes (PEC) producing shoots only, roots only or display- ing no reaction after a period of six weeks on induction media supplemented with NAA and BA (NB) or NAA and 2-iP (NI) and submitted to a 16 h photoperiod. Explant nIM Shoots Roots No reaction 90NB 11.5 21.5 0 ISE 90NI 16.5 34.0 1.5 78NB 15.5 5.5 7.5 PEC 78NI 33.0 2.5 14.0 Copyright © 2013 SciRes. OPEN A CCESS
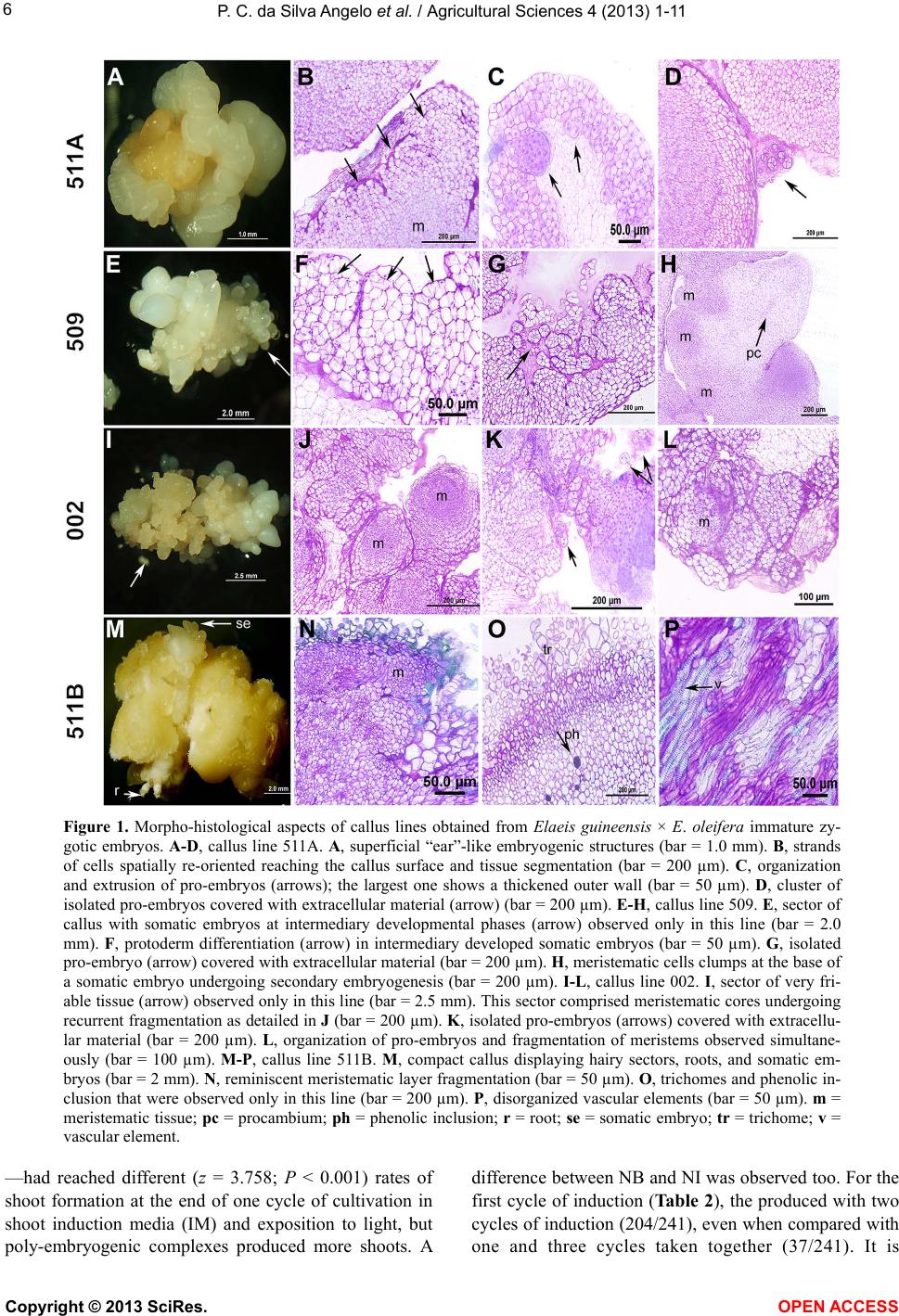 P. C. da Silva Angelo et al. / Agricultural Sciences 4 (2013) 1-11 Copyright © 2013 SciRes. 6 Figure 1. Morpho-histological aspects of callus lines obtained from Elaeis guineensis × E. oleifera immature zy- gotic embryos. A-D, callus line 511A. A, superficial “ear”-like embryogenic structures (bar = 1.0 mm). B, strands of cells spatially re-oriented reaching the callus surface and tissue segmentation (bar = 200 µm). C, organization and extrusion of pro-embryos (arrows); the largest one shows a thickened outer wall (bar = 50 µm). D, cluster of isolated pro-embryos covered with extracellular material (arrow) (bar = 200 µm). E-H, callus line 509. E, sector of callus with somatic embryos at intermediary developmental phases (arrow) observed only in this line (bar = 2.0 mm). F, protoderm differentiation (arrow) in intermediary developed somatic embryos (bar = 50 µm). G, isolated pro-embryo (arrow) covered with extracellular material (bar = 200 µm). H, meristematic cells clumps at the base of a somatic embryo undergoing secondary embryogenesis (bar = 200 µm). I-L, callus line 002. I, sector of very fri- able tissue (arrow) observed only in this line (bar = 2.5 mm). This sector comprised meristematic cores undergoing recurrent fragmentation as detailed in J (bar = 200 µm). K, isolated pro-embryos (arrows) covered with extracellu- lar material (bar = 200 µm). L, organization of pro-embryos and fragmentation of meristems observed simultane- ously (bar = 100 µm). M-P, callus line 511B. M, compact callus displaying hairy sectors, roots, and somatic em- bryos (bar = 2 mm). N, reminiscent meristematic layer fragmentation (bar = 50 µm). O, trichomes and phenolic in- clusion that were observed only in this line (bar = 200 µm). P, disorganized vascular elements (bar = 50 µm). m = meristematic tissue; pc = procambium; ph = phenolic inclusion; r = root; se = somatic embryo; tr = trichome; v = vascular element. —had reached different (z = 3.758; P < 0.001) rates of shoot formation at the end of one cycle of cultivation in shoot induction media (IM) and exposition to light, but poly-embryogenic complexes produced more shoots. A difference between NB and NI was observed too. For the first cycle of induction (Table 2), the produced with two cycles of induction (204/241), even when compared with ne and three cycles taken together (37/241). It is o OPEN ACCESS
 P. C. da Silva Angelo et al. / Agricultural Sciences 4 (2013) 1-11 7 Figure 2. Profile of gene expression in Elaeis guineensis × E. olei fera hybrids. A, amplicons for callus lines 511A, 509, 002 and 511B. B, amplicons for immature zygotic embryos, col- lected 90 days after pollination. C, calibrated quantity of fluorescence to the amplicons for each callus line. Amplicons were produced by RT-PCR and fluorescence was calibrated by that of the internal reference gene. Columns represent mean values for three replicates and bars represent the standard deviations of the means. SK = SERK (somatic embryogenesis re- ceptor kinase); DH = DEHYDRIN ; DF = DEFENSIN; LT = LIPID TRANSFER PROTEIN; TR = TRANSPOSASE; EF = ELONGATION FACTOR 1, the internal reference gene. M = 1 kb Plus Ladder (Invitrogen). interesting to note that individualized pearshaped em- bryos often produced multiple shoots too (Figures 3C and D), probably as consequence of secondary embryo- genesis (Figure 1H), and this feature contributed to the final scores. We obtained 47 shoots above 6 cm from individualized somatic embryos (26% in 180, Table 2) and 241 from poly-embryogenic complexes (1.54 shoots/ explant in average; 1.46 for callus line 509 and 1.56 for callus line 002). Some shoots were used for rooting experiments (Fig- ure 3E) following separation from the complexes. De- spite media supplemented with 60 g·l−1 of sucrose and 16.7 mg·l−1 NAA promoted rooting in higher frequencies, the concentrations of sugars and NAA tested did not dif- fer statistically. Percentages of rooting were 77 and 82 for shoots coming from poly-embryogenic complexes or individualized embryoids, respectively. 4. DISCUSSION In this work, the goal was to clone E. guineensis × E. oleifera zygotic embryos through somatic embryogene- Figure 3. Overview of in vitro regenerated plantlets of Elaeis guineensis × E. oleifera hybrids. A-B, shoots emerging from poly-embryogenic complexes. C-D, shoots produced from in- dividualized somatic embryos. E, shoots transferred for rooting media. sis. Histological and reverse transcription techniques were used to complement morphology as support for Copyright © 2013 SciRes. OPEN A CCESS
 P. C. da Silva Angelo et al. / Agricultural Sciences 4 (2013) 1-11 8 decisions, going deeper on the comprehension of em- bryogenesis and multiplication/maturation of embryo- genic cultures, and to institute molecular markers to as- sist selection of the best callus lines, maybe among thousands if numerous progenies are going to be cloned. An example would be the CYCLIN DEPENDENT KINASE from coconut palm, which increased expression, marked the emergence of “ear”-like embryogenic struc- tures [30]. “Ear”-like structures were reported decades ago for E. guineensis [8] and observed in coconut [15] and Bactris [23]. In the present study, they became visible during the initial months of cultivation and again following the re- duction in auxin concentration, which induced culture 511 to revert throughout the route for embryogenesis to produce callus line 511A. For this last callus line, histo- logical analysis revealed the migration of meristematic activity from the center of the calli to the periphery in the “ear”-like embryogenic structures, “where cells became spatially organized in strands, and the tissues entered segmentation. Rearrangements like these could be the prognostication of the development of “clusters of pro- embryos, which would remain fused to form poly-em- bryogenic complexes” [8]. In addition, rare clusters of isolated globular pro-embryos, with a reduced number of cells were also observed. These results all together indi- cated the occurrence of more than one route for embryo- genesis in callus line 511A. Somatic embryos would ori- ginate from one or a few cells [28], or from the segmen- tation of sectors of meristematic “cells re-oriented to divide anticlinally, rather than periclinally”, what was suggested to occur in barley too [8, 31]. Nevertheless, all the somatic embryos promoted to the experimental phase of shoot regeneration in this work came from poly-em- bryogenic complexes and not from spontaneously iso- lated somatic embryo. Multiple meristematic cores were observed in 511A and the other callus lines, certainly resulting from the fragmentation of an initial meristem in each zygotic em- bryo. The importance of cellular injuries inflicted to the external meristematic layers and/or the central and pe- ripheral zones of the shoot apical meristem (SAM) to induce its segmentation and the organization of multiple meristem cores has been demonstrated [32]. These pro- cesses possibly occurred in association with the appear- ing of “ear”-like embryogenic structures. Periclinal cellu- lar divisions would trigger radial expansion in detriment of somatic embryos multiplication/maturation. Radial ex- pansion could impel meristematic activity from the inner cores of the calli towards its surface, finally causing the mechanical rupture of the external cell layers, inducing fragmentation, followed by spatial reorganization and tissue segmentations. It is plausible to suppose that under ideal conditions the equilibrium between multiplication, fragmentation and reorganization of peripheral meris- tematic cells is achieved and the proper number of cellu- lar layers and rows is settled in individual neighbor api- cal meristems. Thereafter, photomorphogenesis and dif- ferentiation of multiple shoots from poly-embryogenic complexes become possible. The entrance in equilibrium would be interesting to mark in order to avoid unneces- sary exposition of the cultures to high concentrations of auxins. Differing from Bactris [26], isolated “ear”-like struc- tures could not be induced to display secondary embryo- genesis by cultivation in medium supplemented with 2.4 mg· l −1 Picloram. Isolation and sequential reductions in the concentration of auxins resulted in a degenerated callus line with organogenic characteristics. This demons- trated that at least 8 mg·l−1 of 2,4-D were essential to maintain the highly embryogenic features observed in callus lines 511 (not shown) and 509, at least, which were multiplied for more than a year. Once transferred back for media containing 8 mg·l−1, calli from 511A re- sumed the characteristics observed in 511, produced so- matic embryos and plantlets (results not show). Cultivation in medium free of growth regulators dur- ing the stage of multiplication of the embryogenic cul- tures would be essential to obtain normal regenerated plantlets, when this stage is to be maintained for very long periods, as 20 years [13]. In the present work the best condition for multiplication-maturation of somatic embryos was 8 mg·l−1 2,4-D applied for a little more than a year [21]. Characteristics resembling the description of fast growing cultures, that would be associated with the mantled anomaly were only observed in callus line 002. In this reason, we considered that if plants affected are to be expected they will be found among regenerants from that callus line. Expression of SERK genes has been related to the ac- quisition of cellular totipotency, which is essential for embryogenesis [33] and can be followed by pluripotency and finally organogenesis. Indeed, highly embryogenic features, competence for embryogenesis, or embryogene- sis observed simultaneously to organogenesis could ex- plain SERK transcription in the callus lines we studied. These conditions were observed in callus lines 509 and 002, in callus line 511A, which was very similar to the coconut calli expressing SERK in peripheral embryo- genic structures [15] and finally in 511B, respectively. On the other hand, in Rosa hybrida cv. Linda two out of four SERK genes analyzed were good markers for so- matic embryogenesis but would not be exclusively re- lated to embryogenesis, despite no conclusion about al- ternative functions for those gene products had been proposed [34]. The ubiquitous expression of DEHYDRIN and DE- FENSIN was reputed to the stresses imposed equally to Copyright © 2013 SciRes. OPEN A CCESS
 P. C. da Silva Angelo et al. / Agricultural Sciences 4 (2013) 1-11 9 all the cultures as stated previously [18]. Dehydrins are late embryogenesis abundant (LEA) proteins related to the acquisition of desiccation tolerance but, in addition, they have been clearly related to stress responses, as well as defensins [24,35-38]. The differences among the ex- pression profiles of those two genes and that of the LIPID TRANSFER PROTEIN led us to conclude that transcription of this last was not related to stress in our experiments. We propose that the differences in those patterns of multiplication/maturation were the reasons for the exclu- sive expression of the LIPID TRANSFER PROTEIN in line 509. Callus line 509 presented mid-term pro-em- bryos, going through protoderm organization, fixed to a compact basal callus. Callus line 002 presented finely fragmented meristematic sectors going through cyclic multiplication. We considered the LIPID TRANSFER PROTEIN transcription as a transient indicator of route definition towards embryo maturation, probably going on more intensely in the poly-embryogenic complexes of callus line 509, in contrast with the prevalence of cyclic multiplication and fragmentation of meristematic cores in callus line 002. Reverse transcription was performed using the maximum amount of total RNA (4 μg) recom- mended for 20 μl standard reactions and a great number of PCR cycles were used for cDNA amplification in each biological replicate. These procedures assured that the results captured the differential transcription of the LIPID TRANSFER PROTEIN. Indeed, transcription in callus lines 002, 511A and 511B, if there was any, was so minimal that it could not be detected. In carrot, all the cell masses with diameters between 30 and 50 µm that produced somatic embryos were found to express the LIPID TRANSFER PROTEIN, what was not observed in the earliest stages, and expression was proposed to occur in concert with protoderm formation [39]. We detected transcription of the LIPID TRANSFER PROTEIN in 90 days old zygotic embryos, and this pointed out for the possibility of an extended period of expression in Elaeis. However, it was demonstrated in rice that expression of LIPID TRANSFER PROTEIN changed spatially during maturation from the protoderm, for the leaf primordium, and finally for the vascular bundles [40]. The association of a lipid transfer protein with the cytoplasm, cell walls, and spaces between protodermal and subprotodermal cells in embryogenic but not in meristematic cells of the SAM was recently reported for a lipid transfer protein in Arabidopsis thaliana. It was considered that some rela- tion between the accumulation of the protein and the differentiation/dedifferentiation—named together as chan- ge of developmental direction—could exist [41]. The absence in the SAM of a protein expressed when tissues become committed to differentiation would be coherent since it is expected to last nondifferentiated for long pe- riods [33]. Finally, a correlation between the lipid trans- fer protein and the accumulation of reserves in the em- bryos cannot be completely discarded, neither some in- fluence of the differences among the genotypes of the callus lines on the intensity of gene expression, in reason of the complex origin of the embryos. Transposon activation via expression of TRANSPO- SASE has been correlated with the chromatin demethyla- tion and remodeling in presence of 2,4-D [42], which was considered ultimately a stress factor that can influ- ence chromatin conformation and induce embryogenesis [43]. The presence of ESTs homologous to TRANSPO- SASE was exclusively associated with the occurrence of embryogenesis in E. guineensis [19] but transposons were detected in tissues of tenera collected in and ex vitro with no remarkable differences in distribution/lo- calization [44]. For regeneration of plantlets, those poly-embryogenic complexes that produced green, leaf-like structures were transferred to half-strength basal medium free of growth regulators for shoot elongation up to 6 cm, and those which did not present such structures were submitted to further cycles of shoot induction. The induction of shoots on poly-embryogenic complexes was proven successful [12,13] as proposed earlier for the interspecific hybrids [45]. Cultivating poly-embryogenic complexes in me- dium supplemented with abscisic acid [9] before shoot induction might contribute to synchronization [46]. The procedures described above reduced the manipulation required to individualize embryos mechanically, contri- buting to decrease contamination rates and to obtain rooted plantlets ready for acclimatization in a year and five months. 5. ACKNOWLEDGEMENTS We are grateful to Embrapa (grant #02.09.03.005.00) for financial support, to Aline Mabel Rosa for sectioning the tissues for histological analyses, Alison Gonçalves Nazareno for assistance with the automatic sequencer, Nelson Lourenço de Paula, Raimundo Oliveira do Nasci- mento, and Raimundo César Pereira de Moraes for excising the zygotic embryos and Raimundo Rocha for collects at Rio Urubu Experimental Station. REFERENCES [1] Homma, O., Furlan Júnior J., Carvalho R.A. and Ferreira, C.A.P. (2000) Bases para uma política de desenvol- vimento da cultura do dendezeiro na Amazônia. In: Viégas, I.J.M. and Müller, A.A., Eds., A Cultura do Den- dezeiro na Amazônia Brasileira. Embrapa Amazônia Ori- ental-Embrapa Amazônia Ocidental, Brasil, 11-30. [2] Fiorese, C. (2008) Notícias da Amazônia. http://www.noticiasdaamazonia.com.br/2521-com-fabrica -no-para-natura-aposta-na-industria-sustentavel/ Copyright © 2013 SciRes. OPEN A CCESS
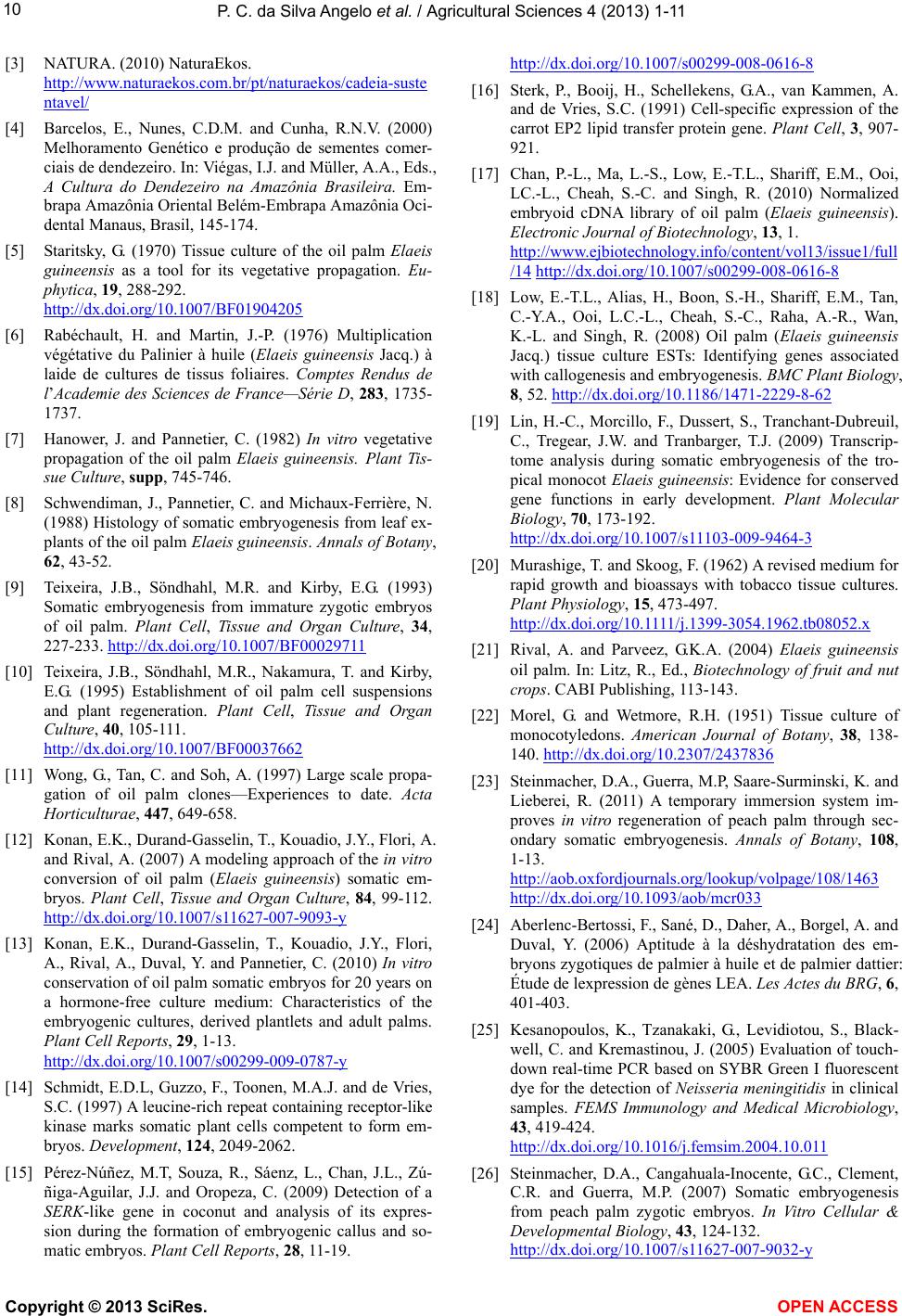 P. C. da Silva Angelo et al. / Agricultural Sciences 4 (2013) 1-11 10 [3] NATURA. (2010) NaturaEkos. http://www.naturaekos.com.br/pt/naturaekos/cadeia-suste ntavel/ [4] Barcelos, E., Nunes, C.D.M. and Cunha, R.N.V. (2000) Melhoramento Genético e produção de sementes comer- ciais de dendezeiro. In: Viégas, I.J. and Müller, A.A., Eds., A Cultura do Dendezeiro na Amazônia Brasileira. Em- brapa Amazônia Oriental Belém-Embrapa Amazônia Oci- dental Manaus, Brasil, 145-174. [5] Staritsky, G. (1970) Tissue culture of the oil palm Elaeis guineensis as a tool for its vegetative propagation. Eu- phytica, 19, 288-292. http://dx.doi.org/10.1007/BF01904205 [6] Rabéchault, H. and Martin, J.-P. (1976) Multiplication végétative du Palinier à huile (Elaeis guineensis Jacq.) à laide de cultures de tissus foliaires. Comptes Rendus de l’Academie des Sciences de France—Série D, 283, 1735- 1737. [7] Hanower, J. and Pannetier, C. (1982) In vitro vegetative propagation of the oil palm Elaeis guineensis. Plant Tis- sue Culture, supp, 745-746. [8] Schwendiman, J., Pannetier, C. and Michaux-Ferrière, N. (1988) Histology of somatic embryogenesis from leaf ex- plants of the oil palm Elaeis guineensis. Annals of Botany, 62, 43-52. [9] Teixeira, J.B., Söndhahl, M.R. and Kirby, E.G. (1993) Somatic embryogenesis from immature zygotic embryos of oil palm. Plant Cell, Tissue and Organ Culture, 34, 227-233. http://dx.doi.org/10.1007/BF00029711 [10] Teixeira, J.B., Söndhahl, M.R., Nakamura, T. and Kirby, E.G. (1995) Establishment of oil palm cell suspensions and plant regeneration. Plant Cell, Tissue and Organ Culture, 40, 105-111. http://dx.doi.org/10.1007/BF00037662 [11] Wong, G., Tan, C. and Soh, A. (1997) Large scale propa- gation of oil palm clones—Experiences to date. Acta Horticulturae, 447, 649-658. [12] Konan, E.K., Durand-Gasselin, T., Kouadio, J.Y., Flori, A. and Rival, A. (2007) A modeling approach of the in vitro conversion of oil palm (Elaeis guineensis) somatic em- bryos. Plant Cell, Tissue and Organ Culture, 84, 99-112. http://dx.doi.org/10.1007/s11627-007-9093-y [13] Konan, E.K., Durand-Gasselin, T., Kouadio, J.Y., Flori, A., Rival, A., Duval, Y. and Pannetier, C. (2010) In vitro conservation of oil palm somatic embryos for 20 years on a hormone-free culture medium: Characteristics of the embryogenic cultures, derived plantlets and adult palms. Plant Cell Reports, 29, 1-13. http://dx.doi.org/10.1007/s00299-009-0787-y [14] Schmidt, E.D.L, Guzzo, F., Toonen, M.A.J. and de Vries, S.C. (1997) A leucine-rich repeat containing receptor-like kinase marks somatic plant cells competent to form em- bryos. Development, 124, 2049-2062. [15] Pérez-Núñez, M.T, Souza, R., Sáenz, L., Chan, J.L., Zú- ñiga-Aguilar, J.J. and Oropeza, C. (2009) Detection of a SERK-like gene in coconut and analysis of its expres- sion during the formation of embryogenic callus and so- matic embryos. Plant Cell Reports, 28, 11-19. http://dx.doi.org/10.1007/s00299-008-0616-8 [16] Sterk, P., Booij, H., Schellekens, G.A., van Kammen, A. and de Vries, S.C. (1991) Cell-specific expression of the carrot EP2 lipid transfer protein gene. Plant Cell, 3, 907- 921. [17] Chan, P.-L., Ma, L.-S., Low, E.-T.L., Shariff, E.M., Ooi, LC.-L., Cheah, S.-C. and Singh, R. (2010) Normalized embryoid cDNA library of oil palm (Elaeis guineensis). Electronic Journal of Biotechnology, 13, 1. http://www.ejbiotechnology.info/content/vol13/issue1/full /14 http://dx.doi.org/10.1007/s00299-008-0616-8 [18] Low, E.-T.L., Alias, H., Boon, S.-H., Shariff, E.M., Tan, C.-Y.A., Ooi, L.C.-L., Cheah, S.-C., Raha, A.-R., Wan, K.-L. and Singh, R. (2008) Oil palm (Elaeis guineensis Jacq.) tissue culture ESTs: Identifying genes associated with callogenesis and embryogenesis. BMC Plant Biology, 8, 52. http://dx.doi.org/10.1186/1471-2229-8-62 [19] Lin, H.-C., Morcillo, F., Dussert, S., Tranchant-Dubreuil, C., Tregear, J.W. and Tranbarger, T.J. (2009) Transcrip- tome analysis during somatic embryogenesis of the tro- pical monocot Elaeis guineensis: Evidence for conserved gene functions in early development. Plant Molecular Biology, 70, 173-192. http://dx.doi.org/10.1007/s11103-009-9464-3 [20] Murashige, T. and Skoog, F. (1962) A revised medium for rapid growth and bioassays with tobacco tissue cultures. Plant Physiology, 15, 473-497. http:// dx.doi.org/1 0.1111/j.1399-3054.1962.tb08052.x [21] Rival, A. and Parveez, G.K.A. (2004) Elaeis guineensis oil palm. In: Litz, R., Ed., Biotechnology of fruit and nut crops. CABI Publishing, 113-143. [22] Morel, G. and Wetmore, R.H. (1951) Tissue culture of monocotyledons. American Journal of Botany, 38, 138- 140. http://dx.doi.org/10.2307/2437836 [23] Steinmacher, D.A., Guerra, M.P, Saare-Surminski, K. and Lieberei, R. (2011) A temporary immersion system im- proves in vitro regeneration of peach palm through sec- ondary somatic embryogenesis. Annals of Botany, 108, 1-13. http://aob.oxfordjournals.org/lookup/volpage/108/1463 http://dx.doi.org/10.1093/aob/mcr033 [24] Aberlenc-Bertossi, F., Sané, D., Daher, A., Borgel, A. and Duval, Y. (2006) Aptitude à la déshydratation des em- bryons zygotiques de palmier à huile et de palmier dattier: Étude de lexpression de gènes LEA. Les Actes du BRG, 6, 401-403. [25] Kesanopoulos, K., Tzanakaki, G., Levidiotou, S., Black- well, C. and Kremastinou, J. (2005) Evaluation of touch- down real-time PCR based on SYBR Green I fluorescent dye for the detection of Neisseria meningitidis in clinical samples. FEMS Immunology and Medical Microbiology, 43, 419-424. http://dx.doi.org/10.1016/j.femsim.2004.10.011 [26] Steinmacher, D.A., Cangahuala-Inocente, G.C., Clement, C.R. and Guerra, M.P. (2007) Somatic embryogenesis from peach palm zygotic embryos. In Vitro Cellular & Developmental Biology, 43, 124-132. http://dx.doi.org/10.1007/s11627-007-9032-y Copyright © 2013 SciRes. OPEN A CCESS
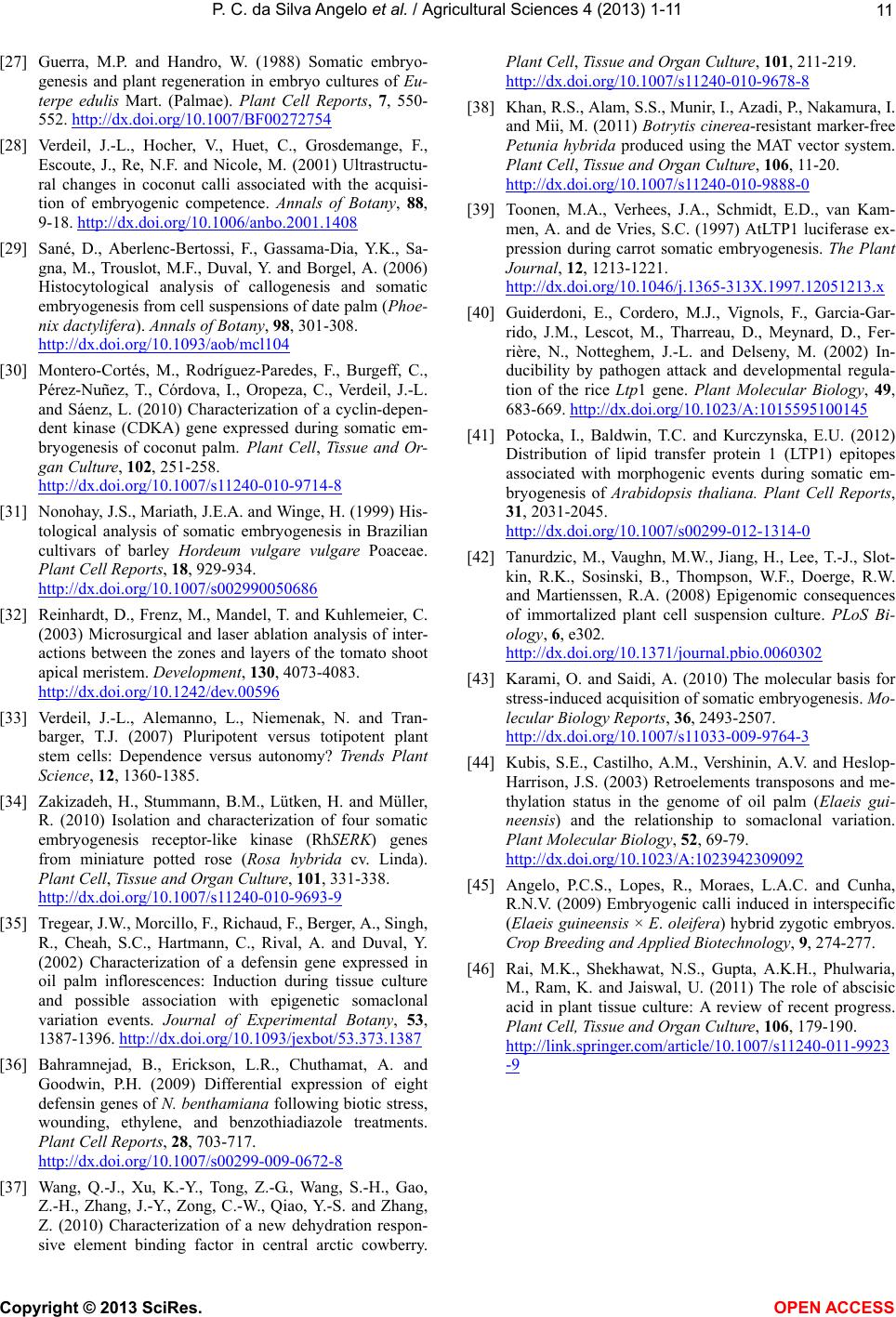 P. C. da Silva Angelo et al. / Agricultural Sciences 4 (2013) 1-11 Copyright © 2013 SciRes. OPEN A CCESS 11 [27] Guerra, M.P. and Handro, W. (1988) Somatic embryo- genesis and plant regeneration in embryo cultures of Eu- terpe edulis Mart. (Palmae). Plant Cell Reports, 7, 550- 552. http://dx.doi.org/10.1007/BF00272754 [28] Verdeil, J.-L., Hocher, V., Huet, C., Grosdemange, F., Escoute, J., Re, N.F. and Nicole, M. (2001) Ultrastructu- ral changes in coconut calli associated with the acquisi- tion of embryogenic competence. Annals of Botany, 88, 9-18. http://dx.doi.org/10.1006/anbo.2001.1408 [29] Sané, D., Aberlenc-Bertossi, F., Gassama-Dia, Y.K., Sa- gna, M., Trouslot, M.F., Duval, Y. and Borgel, A. (2006) Histocytological analysis of callogenesis and somatic embryogenesis from cell suspensions of date palm (Phoe- nix dactylifera). Annals of Botany, 98, 301-308. http://dx.doi.org/10.1093/aob/mcl104 [30] Montero-Cortés, M., Rodríguez-Paredes, F., Burgeff, C., Pérez-Nuñez, T., Córdova, I., Oropeza, C., Verdeil, J.-L. and Sáenz, L. (2010) Characterization of a cyclin-depen- dent kinase (CDKA) gene expressed during somatic em- bryogenesis of coconut palm. Plant Cell, Tissue and Or- gan Culture, 102, 251-258. http://dx.doi.org/10.1007/s11240-010-9714-8 [31] Nonohay, J.S., Mariath, J.E.A. and Winge, H. (1999) His- tological analysis of somatic embryogenesis in Brazilian cultivars of barley Hordeum vulgare vulgare Poaceae. Plant Cell Reports, 18, 929-934. http://dx.doi.org/10.1007/s002990050686 [32] Reinhardt, D., Frenz, M., Mandel, T. and Kuhlemeier, C. (2003) Microsurgical and laser ablation analysis of inter- actions between the zones and layers of the tomato shoot apical meristem. Development, 130, 4073-4083. http://dx.doi.org/10.1242/dev.00596 [33] Verdeil, J.-L., Alemanno, L., Niemenak, N. and Tran- barger, T.J. (2007) Pluripotent versus totipotent plant stem cells: Dependence versus autonomy? Trends Plant Science, 12, 1360-1385. [34] Zakizadeh, H., Stummann, B.M., Lütken, H. and Müller, R. (2010) Isolation and characterization of four somatic embryogenesis receptor-like kinase (RhSERK) genes from miniature potted rose (Rosa hybrida cv. Linda). Plant Cell, Tissue and Organ Culture, 101, 331-338. http://dx.doi.org/10.1007/s11240-010-9693-9 [35] Tregear, J.W., Morcillo, F., Richaud, F., Berger, A., Singh, R., Cheah, S.C., Hartmann, C., Rival, A. and Duval, Y. (2002) Characterization of a defensin gene expressed in oil palm inflorescences: Induction during tissue culture and possible association with epigenetic somaclonal variation events. Journal of Experimental Botany, 53, 1387-1396. http://dx.doi.org/10.1093/jexbot/53.373.1387 [36] Bahramnejad, B., Erickson, L.R., Chuthamat, A. and Goodwin, P.H. (2009) Differential expression of eight defensin genes of N. benthamiana following biotic stress, wounding, ethylene, and benzothiadiazole treatments. Plant Cell Reports, 28, 703-717. http://dx.doi.org/10.1007/s00299-009-0672-8 [37] Wang, Q.-J., Xu, K.-Y., Tong, Z.-G., Wang, S.-H., Gao, Z.-H., Zhang, J.-Y., Zong, C.-W., Qiao, Y.-S. and Zhang, Z. (2010) Characterization of a new dehydration respon- sive element binding factor in central arctic cowberry. Plant Cell, Tissue and Organ Culture, 101, 211-219. http://dx.doi.org/10.1007/s11240-010-9678-8 [38] Khan, R.S., Alam, S.S., Munir, I., Azadi, P., Nakamura, I. and Mii, M. (2011) Botrytis cinerea-resistant marker-free Petunia hybrida produced using the MAT vector system. Plant Cell, Tissue and Organ Culture, 106, 11-20. http://dx.doi.org/10.1007/s11240-010-9888-0 [39] Toonen, M.A., Verhees, J.A., Schmidt, E.D., van Kam- men, A. and de Vries, S.C. (1997) AtLTP1 luciferase ex- pression during carrot somatic embryogenesis. The Plant Journal, 12, 1213-1221. http://dx.doi.org/10.1046/j.1365-313X.1997.12051213.x [40] Guiderdoni, E., Cordero, M.J., Vignols, F., Garcia-Gar- rido, J.M., Lescot, M., Tharreau, D., Meynard, D., Fer- rière, N., Notteghem, J.-L. and Delseny, M. (2002) In- ducibility by pathogen attack and developmental regula- tion of the rice Ltp1 gene. Plant Molecular Biology, 49, 683-669. http://dx.doi.org/10.1023/A:1015595100145 [41] Potocka, I., Baldwin, T.C. and Kurczynska, E.U. (2012) Distribution of lipid transfer protein 1 (LTP1) epitopes associated with morphogenic events during somatic em- bryogenesis of Arabidopsis thaliana. Plant Cell Reports, 31, 2031-2045. http://dx.doi.org/10.1007/s00299-012-1314-0 [42] Tanurdzic, M., Vaughn, M.W., Jiang, H., Lee, T.-J., Slot- kin, R.K., Sosinski, B., Thompson, W.F., Doerge, R.W. and Martienssen, R.A. (2008) Epigenomic consequences of immortalized plant cell suspension culture. PLoS Bi- ology, 6, e302. http://dx.doi.org/10.1371/journal.pbio.0060302 [43] Karami, O. and Saidi, A. (2010) The molecular basis for stress-induced acquisition of somatic embryogenesis. Mo- lecular Biology Reports, 36, 2493-2507. http://dx.doi.org/10.1007/s11033-009-9764-3 [44] Kubis, S.E., Castilho, A.M., Vershinin, A.V. and Heslop- Harrison, J.S. (2003) Retroelements transposons and me- thylation status in the genome of oil palm (Elaeis gui- neensis) and the relationship to somaclonal variation. Plant Molecular Biology, 52, 69-79. http://dx.doi.org/10.1023/A:1023942309092 [45] Angelo, P.C.S., Lopes, R., Moraes, L.A.C. and Cunha, R.N.V. (2009) Embryogenic calli induced in interspecific (Elaeis guineensis × E. oleifera) hybrid zygotic embryos. Crop Breeding and Applied Biotechnology, 9, 274-277. [46] Rai, M.K., Shekhawat, N.S., Gupta, A.K.H., Phulwaria, M., Ram, K. and Jaiswal, U. (2011) The role of abscisic acid in plant tissue culture: A review of recent progress. Plant Cell, Tissue and Organ Culture, 106, 179-190. http://link.springer.com/article/10.1007/s11240-011-9923 -9
|