 Journal of Cosmetics, Dermatological Sciences and Applications, 2013, 3, 55-63 Published Online November 2013 (http://www.scirp.org/journal/jcdsa) http://dx.doi.org/10.4236/jcdsa.2013.33A2013 Open Access JCDSA 55 Impact of Celosia cristata Extract on Adipogenesis of Native Human CD34+/CD31− Cells* Richard Fitoussi1, David Estève2, Anne-Sophie Delassus1, Katell Vié1# 1Centre de Recherche Clarins, Pontoise, France; 2Inserm, UMR1048, Team 1, Institute of Metabolic and Cardiovascular Diseases, Toulouse, France. Email: richard.fitoussi@clarins.net, #katell.vie@clarins.net Received October 11th, 2013; revised November 8th, 2013; accepted November 16th, 2013 Copyright © 2013 Richard Fitoussi et al. This is an open access article distributed under the Creative Commons Attribution License, which permits unrestricted use, distribution, and reproduction in any medium, provided the original work is properly cited. ABSTRACT Background: The adipose tissue mainly consists of adipocytes but also contains non-adipose cells. Among them, pro- genitor cells represent a local pool of immature cells that, in vitro, can undergo various lineage differentiation processes. These cells are thought to contribute to normal homeostasis of the adipose tissue through adipogenesis but also to the growth of the adipose tissue under chronic energy overload. The aim of the present study is to evaluate in vitro the ca- pacity of a Celosia cristata extract to impact the adipogenic potential of native human adipose tissue progenitor cells, i.e. commitment and differentiation towards adipogenic lineage. Methods: Native adipose tissue progenitor cells were isolated by immunoselection/depletion approaches from human subcutaneous adipose tissues. Two distinct cell culture conditions were used to assess the effect of Celosia cristata extract on commitment and differenciation of progenitor cells. Cells were cultured either in differentiation medium for 10 days in the presence/absence of Celosia cristata ex- tracts to study the impact on differentiation or first cultured in a commitment-inducing medium, with or without Celosia cristata extract, for 48 h and then cultured 10 days in differentiation medium to assess the impact on commitment. In both experimental series, the fate of progenitor cells was studied by quantification of lipids and by determining the ex- pression of key genes involved in adipogenesis. Results: Data show that Celosia cristata extract reduces lipid content of progenitor cells undergoing differentiation. This reduction correlates with a reduced expression of C/EBPα. When progenitor cells are placed in commitment-inducing conditions, Celosia cristata extract induces a more potent reduction of lipid content. This reduction correlates with a decrease in the expression levels of master genes involved in adipo- genesis: the genes of transcription factors PPARγ2 and C/EBPα as well as marker genes coding for LPL and GPDH. Conclusions: Celosia cristata extract decreases adipogenesis. The effect of the extract is stronger when studying com- mitment and differentiation than differentiation alone; it suggests that the extract impact the commitment of human adipose tissue progenitor cells. Keywords: Adipose Tissue; Stem/Progenitor Cells; Adipogenic Commitment; Adipogenic Differentiation 1. Background The increasing incidence of obesity and obesity-related morbidity has paid attention on the adipose tissue. Nev- ertheless, adipose tissue is not only a fat store. It also plays a pivotal role in energy homeostasis due to its metabolic and secretory activities. Depending on its ana- tomical location, it exhibits distinct metabolic and secre- tory properties as well as growth capacity [1]. If the adipose tissue is mainly composed of adipocytes, it also contains non-adipose cells grouped under the term stroma-vascular fraction. This fraction includes several cell populations: vascular cells, immune cells as well as cells identified as progenitor cells. In human adult adi- pose tissue, progenitor cells were identified by their sur- face markers, as CD34 positive cells and CD31 negative (CD34+/CD31−) cells [2,3]. They represent a local pool of immature cells that exhibits stem/progenitor cell prop- erties. Depending on the in vitro culture conditions, they show the ability to express specific lineage markers of mesenchymal stromal cells and are able to undergo adi- pogenesis, osteogenesis, chondrogenesis and angio- *Competing interests: Richard Fitoussi, Anne-Sophie Delassus and Katell Vié are employed full time by Clarins Company and this study was financed by Clarins. Clarins is a major company specialised in the design, manufacturing and marketing of cosmetic products. #Corresponding author.
 Impact of Celosia cristata Extract on Adipogenesis of Native Human CD34+/CD31− Cells 56 genesis as well as neurogenesis [4,5]. In addition, the human adipose tissue progenitor cells exhibit prolifera- tion and migratory potentials. In vivo approaches in mur- ine models have clearly shown that human adipose tis- sue progenitor cells have the capacity to promote the repair of damaged or ischemic tissues including heart, muscle and bone [6-8]. Under normal conditions, adipose tissue progenitor cells are thought to contribute to the cellular homeostasis of the adipose tissue by providing new adipocytes through adipogenesis and contributing probably to local angiogenesis. Indeed, it is now well established that ap- proximately, 10% of the adipocytes are renewed per year [9]. Under chronic energy overload, the growth of the adipose tissue is due to an increase in the number of adi- pocytes through differentiation of progenitor cells (hy- perplasia) but also increase in the size of the adipocytes (hypertrophy). Progenitor cells might also be critical in causing regional variation in fat tissue function and de- velopment [10]. The model of adipose conversion was mainly estab- lished in cell lines from embryonic or adult murine cells. It is a sequential process whose first step is the determi- nation of progenitor cells into adipoblasts that are then committed into preadipocytes [11]. In mice, the bone morphogenic proteins (BMPs) and more specific BMP2, BMP4 and BMP7 have been described as promoting commitment towards the adipocyte lineage [12]. There- after, under the stimuli of adipogenic factors including insulin, cortisol and peroxisome proliferator activated receptor (PPAR) gamma agonist, committed preadipo- cyte differentiate into adipocyte. In human adults, very few data are available concerning the state of the pro- genitor cells, (i.e. uncommitted adipoblats, committed preadipocytes and differentiated preadipocytes), the se- quence of the process of adipose conversion and the mechanisms that control the appearance of new adipo- cytes within the adipose tissue. In a search for anti-obesity agents, different substances were shown to modulate adipose conversion [13-15]. In this study, we evaluate the capacity of a Celosia cristata extract to impact adipose conversion of human adipose tissue progenitor cells studying commitment and/or dif- ferentiation towards the adipogenic lineage. 2. Methods 2.1. Celosia cristata Extract An extract of Celosia cristata (Silab, Brive, France) was used. The crude extract is a lyophilised homogenate of flowering tops from China. For experiments, the crude extract was dissolved in water to prepare a stock solution. This stock solution was then diluted in the media to a final concentration of 0.05%, 0.1% and 0.5%. 2.2. Isolation of Progenitor Cells from Human Adipose Tissue Human subcutaneous adipose tissues were obtained from healthy women undergoing elective surgery for aesthetic fat removal (mean age 43.8 ± 1.3 years, mean body mass index 27.7 ± 0.6 kg/m2). All patients gave their informed consent and the protocol of the study was approved by the Institutional Research Board of INSERM and Tou- louse University Hospital. After removal, the adipose tissue was immediately di- gested. Tissues were placed in phosphate-buffered saline (PBS) containing 250 U/ml of collagenase (Worthington Biochemical Corporation, Lakewood, USA) and 2% bo- vine serum albumin, pH 7.4 and incubated 30 min at 37˚C under constant shaking. After centrifugation (300 g, 10 min, RT), the pellet containing the stroma vascular fraction was resuspended in erythrocyte lysis buffer (155 mmol/l NH4Cl, 5.7 mmol/l K2HPO4, 0.1 mmol/l EDTA, pH 7.3) for 10 min. After successive filtrations through 100, 70 and 40 µm sieves, the stroma vascular fraction cells were pelleted and then resuspended in PBS supple- mented with 2% fetal calf serum. Adipose tissue progenitor cells were isolated from the stroma vascular fraction using an immuno-selection/de- pletion protocol as previously described [14]. Briefly, the stroma vascular fraction was incubated 15 min at RT with Stem Cell Technologies CD34 positive selection antibody cocktail (St. Katharinen, Germany). Following the addition of magnetic nanoparticles, cells were recov- ered by successive magnetic sorting steps. Cells were suspended in PBS supplemented with 0.1% BSA. Pro- genitor cells, that are CD34+/CD31−, were depleted from CD31+ cells using Life Technologies® (Carlsbad, Cali- fornie) CD31-coupled magnetic Dynal microbeads (50 μl/ml). After incubation (4˚C, 20 min), the cells were suspended in 10 ml PBS with 0.1% BSA, and exposed to the magnet for 1 min. The magnetic bead-free fraction containing CD34+/CD31− progenitor cells, was collected, centrifuged (250 g, 10 min) and suspended in culture medium. 2.3. Culture Conditions of Progenitor Cells Freshly harvested progenitor cells were plated at a den- sity of 120,000 cells/cm2 and cultured according to one of the two protocols described bellow. To assess the impact of Celosia cristata extracts on adipogenic differentiation only, cells were cultured in ECBM (Endothelial Cell Basal Medium, Promocell, Germany) supplemented with 10% fetal calf serum (FSC) for 48 h. Cells were washed and then cultured in ECBM supplemented with 66 nM Insulin, 10 µg/ml Transferrin, 1 nM Triiodothyronine, 100 nM cortisol and 1 µg/ml and 1 µg/ml Rosiglitazone (ITTCR) (Day 0) with or without Open Access JCDSA
 Impact of Celosia cristata Extract on Adipogenesis of Native Human CD34+/CD31− Cells 57 the Celosia cristata extract (0.05%, 0.1% or 0.5%). Cells were incubated at 37˚C in an atmosphere containing 5% of CO2 and media were changed every four days. At day 10, cells were analysed. In a second experimental series, cells were first cul- tured in a commitment-inducing medium at 37˚C in an atmosphere containing 5% of CO2. To analyse the impact of Celosia cristata extracts, 0.05% or 0.5% or no extract was added to the commitment-inducing medium. After 48 h, cells were washed and culture medium was changed for the differentiation-inducing medium (ITTCR). After 10 days of culture at 37˚C in an atmosphere of 5% CO2 cells were analysed. 2.4. Determination of the Toxicity Associated with the Celosia cristata Extract To evaluate possible cytotoxic effects of Celosia cristata extract, a viability assay was used to determine loss of cell membrane integrity. The ToxiLight™ bioassay kit (Lonza, Switzerland), was used according to manufac- turer’s specifications. Briefly, 20 µl of RT warmed cell culture supernatant was transferred to a 96-well plate and 100 µl of adenylate kinase detection reagent was added. After 5 min at RT, luminescence was recorded. 2.5. Immunofluorescence Microscopy Analysis For immunofluorescence studies, cells were first fixed by 15 minutes incubation in a 4% paraformaldehyde solu- tion at room temperature. After washing, they were stained with Bodipy® 493/503 (10 µg/ml, 15 min, Invi- trogen, Cergy-Pontoise, France) to detect neutral lipids and with Hoechst 33342 (5 µg/ml, 15 min, Invitrogen, Cergy-Pontoise, France) to stain DNA. Excitation and emission wavelengths used to analyse and quantify Bodipy® and Hoechst stainings were respectively 485 nm/538 nm and 385 nm/460 nm. Results were analysed by inversed fluorescent microscope (Nikon Eclipse TE300) and pictures were taken under 20× objective (numeric camera and acquisition software NIS-Elements 2.5 BR, Nikon®). After cells staining with Bodipy® 493/503 and Hoechst fluorescence was quantified using Fluoroscan (fluoro- metric plate reader). Samples were excited at 485 nm and 355 nm and fluorescence emission was measured at 538 and 460 nm for Bodipy and Hoechst respectively. Bodipy® values, measuring lipid accumulation, were normalized by Hoechst values that represent the number of cells. 2.6. Gene Expression of Adipogenesis-Associated Genes Total RNA was extracted from cells using the RNeasy kit (Qiagen, Courtaboeuf, France). After fluorometric quan- tification (Quant-iT™ RiboGreen® RNA Assay Kit, Invi- trogen, Cergy-Pontoise, France), RNA was reverse tran- scribed using Superscript® II kit (Invitrogen, Cergy- Pontoise, France). To ensure the absence of contaminat- ing genomic DNA, reverse transcription controls missing the superscript enzyme were used. Real-time PCR analyses were carried out in 96-well reaction plates with 15 ng of the cDNA samples in a final volume of 20 µl using GeneAmp 7500 detection system (Applied Biosystems, Courtaboeuf, France). Experiments were performed in duplicate with Taqman® assays (Ap- plied Biosystems, Courtaboeuf, France) specific for C/ EBPα (CCAAT/enhancer binding protein-Hs00269972- s1), PPARγ2 (peroxisome proliferator activated receptor -Hs01115510-m1), LPL (lipoprotein lipase-Hs00173425- m1) and GPDH (Glycerol-3-phosphate dehydrogenase - Hs00193386-m1). All reactions were carried out under identical conditions: 50˚C for 2 min, 95˚C for 10 min, followed by 40 cycles of 95˚C for 15 sec and 60˚C for 1 min. Results were analyzed with the GeneAmp 7500 software and all values were normalized to the levels of 18S rRNA (Applied Biosystems, VIC/TAMRA probe). 2.7. Statistical Analysis Values are given as mean ± SE mean for (n) separate experiments. Comparisons between groups were ana- lyzed one-way ANOVA (parametric or non parametric, for repeated measure or not), followed by a Dunn’s mul- tiple comparison test (Prism 4, GraphPad Software, USA). Differences were considered significant when p < 0.05. 3. Results 3.1. Celosia cristata Extract Can Decrease Lipid Content of Progenitor Cells Undergoing Adipogenic Differentiation The effects of Celosia cristata extract on lipid content of progenitor cells undergoing adipogenic differentiation for 10 days was investigated in vitro by cultivating progeni- tor cells in a differentiation medium for 10 days in the absence or presence of 0.05%, 0.1% or 0.5% of the plant extract. As shown in Figure 1(a), control cells show an accumulation of lipids. Cells treated with 0.05% or 0.1% of Celosia cristata extract present no significant differ- ence in the accumulation of lipids. Only cells treated with 0.5% of Celosia cristata extract show a decrease in lipid content as shown by the reduction of Bodipy® stained positive cells. Lipid-associated fluorescence after adipogenic differ- entiation of progenitor cells was quantified. The results (Figure 1(b)) reveal that, compared to control cells (set as 100%), treatment with 0.05% of Celosia cristata ex- tract induces no difference in lipid content. Cells treated Open Access JCDSA
 Impact of Celosia cristata Extract on Adipogenesis of Native Human CD34+/CD31− Cells 58 (a) (b) Figure 1. Lipid content of progenitor cells after 10 days in adipogenic culture condition with or without Celosia cristata extract. (a) Representative microphotographs of progenitor cells cultured for 10 days in adipogenic medium with or without increasing doses of Celosia cristata extract lipids were stained by Bodipy and nuclei by nuclei. Scale bar: 100 µm; (b) Mean fluorescence at 538 nm ± standard error of 2 independent experiments performed on 4 progenitor cell samples from distinct patients and expressed as percentage of the control (*p < 0.05). with 0.1% of Celosia cristata extract show a non-sig- nificant (p > 0.05) decrease in lipid content. Only cells subjected to 0.5% of Celosia cristata extract present a significant (p < 0.05) 44.4% reduction in the accumula- tion of lipids. 3.2. Celosia cristata Extract Does Not Affect Progenitor Cells Viability To determine any possible adverse effects of Celosia cristata extract, a viability assay was performed after 3 days of culture in differentiation medium. As illustrated in Figure 2, control progenitor cells (set as 100%) and progenitor cells cultured in the presence of 0.05%, 0.1% or 0.5% Celosia cristata extract display comparable level of adenylate kinase activity indicating that the perme- ability of cells is not impaired and that their viability is not affected by the treatment with the Celosia cristata extract. 3.3. Celosia cristata Reduces the Expression of Adipogenesis-Associated Genes for Progenitor Cells Undergoing Adipogenic Differentiation Since higher dose (0.5%) of Celosia cristata extract re- duces lipid accumulation in progenitor cells undergoing adipogenic differentiation for 10 days, the effect of the extract on the expression of master genes involved in adipogenesis was investigated after 10 days of culture. We first determined the expression level of an essential adipogenic transcription factor gene: CCAAT/enhancer binding protein alpha (C/EBPα) by real-time PCR analy- ses (n = 3 for each treatment—Figure 3(a)). Compared to untreated control cells the relative expression level of the C/EBPα gene in cells treated with 0.05% and 0.1% Celosia cristata extract show no significant difference (p > 0.05). Only treatment of cells with 0.5% of Celosia cristata extract induces a significant (p < 0.05) decreased expression of C/EBPα gene to a level of 56.0% compared to the control. The effect of Celosia cristata extract on the expression of genes coding for two adipocyte differentiation markers (lipoprotein lipase (LPL) and the Glycerol-3-phosphate dehydrogenase (GPDH)) was also studied by real-time PCR analyses (Figures 3(b) and (c) respectively). For both marker genes, none of the concentration of Celosia cristata extract tested (0.05%, 0.1% or 0.5%) induces significant differences (p < 0.05) in the corresponding gene expression. Nevertheless, it should be noted that a trend in reduction of both gene expression was found that did not reach statistical significance due to high hetero- geneity. 3.4. Celosia cristata Extract Decreases Lipid Content of Progenitor Cells Undergoing Adipogenic Commitment The effect of Celosia cristata on lipid content was also studied on progenitor cells undergoing commitment. Open Access JCDSA
 Impact of Celosia cristata Extract on Adipogenesis of Native Human CD34+/CD31− Cells 59 Figure 2. Cell viability results after 3 days in adipogenic culture condition with or without Celosia cristata extract. Activity of adenylate kinase present in the culture media of progenitor cells, treated or not with Celosia cristata extracts, for three days. Data are mean ± standard error of 2 inde- pendent experiments performed on 3 progenitor cells sam- ples from distinct patients, expressed as percentage of the control. Cells were first cultured for 48 h in a commitment-in- ducing medium in the absence or presence of 0.05% or 0.5% of Celosia cristata extract. They were then cultured in a differentiation medium for 10 days. Analysis of rep- resentative images of lipid-associated fluorescence (Fig- ure 4(a)) show that untreated control cells presents an accumulation of lipids. Cells treated with Celosia cristata extract reveal a reduced green fluorescence, in- dicating a lower lipid accumulation. To quantify these results the fluorescence associated with staining of the lipid content was determined (Figure 4(b)). Compared to control cells (set as 100%), treatment with 0.05% and 0.5% of Celosia cristata extract induces a significant (p < 0.05) reduction of lipid accumulation, respectively 67.2% and 78.7%. 3.5. Celosia cristata Reduces the Expression of Adipogenesis-Associated Genes for Progenitor Cells Undergoing Adipogenic Commitment To gain more insight in the underlying mechanisms leading to the reduction of lipid accumulation, we studied the effect of Celosia cristata extract on expression of two genes coding for transcription factors: C/EBPα and PPARγ2 genes. Real-time PCR analyses was performed on samples from progenitor cells cultured two days in commitment-inducing medium, with or without Celosia cristata extract, followed by 10 days of culture in differ- (a) (b) Figure 3. Expression of adipogenesis-associated genes in progenitor cells after 10 days in adipogenic differentiation condition with or without Celosia cristata extract Gene ex- pression levels, determined by real-time PCR analyses, are mean ± standard error of 2 independent experiments per- formed on 3 progenitor cells samples from distinct patients. Results are expressed as percentage of the control (*p < 0.05). (a) Expression level of C/EBPα transcription factor gene; (b) Expression levels of two adipocyte specific genes: LPL (plain bars) and GDPH (dashed bars). entiation medium. As shown in Figure 5(a), relative ex- pression of the C/EBPα gene was significantly reduced to 82.4% at the lowest dose tested (0.05%). Compared to untreated control cells, the expression level of PPARγ2 gene in the presence of 0.05% and 0.5% of Celosia cristata extract compared to that of control is signifi- cantly (p < 0.05) reduced: 74.8% (±5.9%) and 75.8% (± 7.5%) respectively. No significant differences could be observed between the two doses of extract tested. Open Access JCDSA
 Impact of Celosia cristata Extract on Adipogenesis of Native Human CD34+/CD31− Cells 60 (a) (b) Figure 4. Lipid content of progenitor cells after 10 days in adipogenic culture condition after pre-treatement or not with Celosia cristata extract. (a) Representative micropho- tographs of native progenitor cells treated or not 2 days with indicated doses of Celosia cristata extract lipid accu- mulation was revealed by Bodipy staining and nuclei were stained by Hoechst. Scale bar: 100 µm; (b) Mean fluores- cence at 538 nm ± standard error of 2 independent experi- ments performed on 3 progenitor cells samples from dis- tinct patients and expressed as percentage of the control (*p < 0.05). The effect of Celosia cristata extract on the expression of genes coding for adipocyte differentiation marker, LPL and GPDH, was also studied under the same culture conditions by real-time PCR analysis was performed. Results (Figure 5(b)) show that, compared to control (set as 100%), both doses of Celosia cristata extract induce an almost identical reduction of LPL gene expression: 78.4% at 0.05% and 80.6% at 0.5%. Celosia cristata (a) (b) Figure 5. Expression of adipogenesis-associated genes in progenitor cells after 10 days in adipogenic culture condi- tion after pre-treatement or not with Celosia cristata extract. Gene expression levels, determined by real-time PCR analyses, are mean ± standard error of 2 independent ex- periments performed on 3 progenitor cells samples from distinct patients. Results are expressed as percentage of the control (*p < 0.05). (a) Expression level of the transcription factor gene C/EBPα (plain bars) and PPARγ2 (dashed bars); (b) Expression levels of two adipocyte specific genes: LPL (plain bars) and GDPH (dashed bars). extract also significantly reduces GDPH gene expression, leading to expression levels of 71.2% at 0.05% and 78.0% at 0.5%, these two values being similar according to statistical analysis (p < 0.05). 4. Discussion The adipose tissue has been studied with increasing in- tensity as a result of the emergence of obesity and the realization that it serves as an integrator of various physiological pathways. In particular, it plays a crucial Open Access JCDSA
 Impact of Celosia cristata Extract on Adipogenesis of Native Human CD34+/CD31− Cells 61 role in energy homeostasis thanks to its capacity to store energy. As such, the adipose tissue will expand in period of food superabundance. This expansion implies two processes: the increase in number of adipocytes by adi- pogenesis, i.e. the recruitment of progenitor cells towards the adipose pathway, and the increase in adipocyte size. Since adipogenesis relates to adipocyte differentiation and maturation, inhibiting adipogenesis at various stages, as well as inducing apoptosis, may be promising path- ways to treat obesity and its related morbidity. Several plant extracts and their respective active in- gredients were already shown to affect adipose tissue [14, 15]. The most studied plant is green tea for which several components have been shown to have an effect. In par- ticular the epigallocatechin gallate (EGCG) was shown to reduce triglyceride incorporation during adipogenesis of human subcutaneous progenitor cells [13]. It was also recently implicated in the inhibition of proliferation and differentiation of primary human visceral preadipocytes [16]. Other plant extracts have been show to act on pro- genitor cells. In our case, we investigated the effect of a Celosia cristata flower extract that is used in traditional Chinese and Indian medicine for its astringent and hemo- static properties. We focused on its role on human sub- cutaneous progenitor cells. Since adipose conversion is a sequential process, we used culture conditions that allow discriminating be- tween an action of the Celosia cristata extract on differ- entiation and on commitment. To assess the effect on dif- ferentiation, progenitor cells were cultured in conditions allowing preadipocytes to differentiate in adipocytes. Analysis was performed after 10 days, a period sufficient to analyse fully differentiated fat cells. In contrast, to study the effect of the extract on com- mitment, native progenitor cells were subjected to 2 days of pre-culture in a commitment-inducing medium with or without Celosia cristata extract. During this period, un- committed preadipocytes could engage in the adipose differentiation pathway. This pre-culture was followed by 10 additional days of culture in differentiation medium to allow for development in adipocytes before performing the analysis. Since the Celosia cristata extract was only present during the pre-culture stage, any effect can be attributed to an action during the commitment stage. We first analyse lipid accumulation in differentiated progenitor cells. Our data show that the extract decreases lipid accumulation in progenitor cells. This effect cannot be related to a toxicity effect and occurs whether adipo- cytes results from committed preadipocytes undergoing differentiation only or from uncommitted preadipocytes undergoing commitment and differentiation. Neverthe- less, quantification of lipid accumulation reveals that this decrease occurs only at the highest dose tested (0.5%) in the case of differentiation while it is dose independent and occurs already at the lowest dose tested (0.05%) when the extract is added during commitment. The differences in the dose of extract necessary to have an effect on lipid accumulation suggest possible differences in the underlying mechanisms. To gain more insight in these mechanisms we focused on master genes controlling adipocyte differentiation. Transcription fac- tors PPARγ and members of the C/EBP family play a crucial role in this tightly regulated process. In response to adipogenic signals, C/EBPβ and C/EBPδ are tran- siently expressed, leading to the activation of PPARγ. PPARγ stimulates the expression of C/EBPα that exerts a positive feedback on PPARγ to maintain the differentia- tion process [17]. They control most genes induced dur- ing adipogenesis [18]. We also focus on the expression of two specific adipocyte enzymes, the lipoprotein lipase (LPL) and the Glycerol-3-phosphate dehydrogenase (GPDH). In differentiated adipocytes resulting from committed preadipocytes and undergoing differentiation only, ex- pression analysis of C/EBPα show a reduced expression only when Celosia cristata extract is present at 0.5%. The expression of LPL and GPDH show a trend to de- creased expression. In contrast, when the Celosia cristata extract is present during commitment, expression of adi- pogenesis-related genes is distinct. Both transcription factors, C/EBPα and PPARγ2, show a dose independent reduced expression. Similar results are obtained for LPL and GDPH. Taken together results of lipid accumulation and ex- pression level of adipogenesis-related genes are in good agreements. Celosia cristata extract acts on differentia- tion at higher doses while it acts on commitment at lower doses whether for lipid accumulation or for adipogenesis- related genes expression. These results suggest that the extract impacts predominantly the commitment of human adipose tissue progenitor cells. Nevertheless, we cannot rule completely out the fact that the extract has also a direct effect on differentiation. If the underlying mecha- nism is different it should a priori act upstream of the transcription factors we studied. This assumption is sup- ported by the fact that the marker genes we studied are decreased by treatment with the Celosia cristata extract both when inducing commitment or differentiation. Due to the complexity of the Celosia cristata extract used, it is impossible to exclude that the effect observed is due to different active ingredients. Nevertheless, the fact that the Celosia cristata extract affects adipogenesis in a dose independent manner in the concentration range we tested indicates that Celosia contains one or several powerful compound(s) capable of impacting commitment of human adipose tissue progenitor cells. Many studies describing the effect of plant extracts are performed on cell lines, especially 3T3-L1 preadipocytes Open Access JCDSA
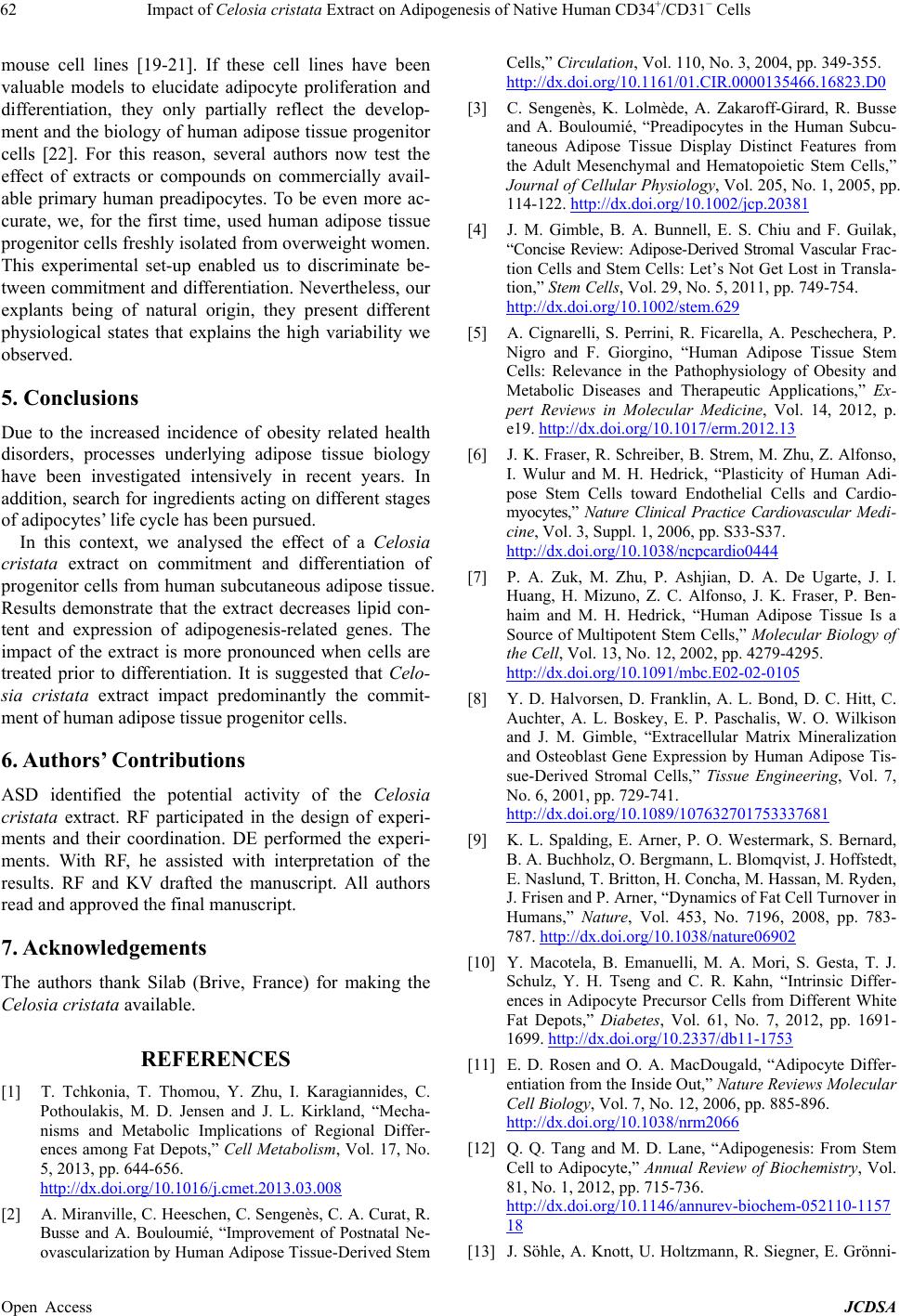 Impact of Celosia cristata Extract on Adipogenesis of Native Human CD34+/CD31− Cells 62 mouse cell lines [19-21]. If these cell lines have been valuable models to elucidate adipocyte proliferation and differentiation, they only partially reflect the develop- ment and the biology of human adipose tissue progenitor cells [22]. For this reason, several authors now test the effect of extracts or compounds on commercially avail- able primary human preadipocytes. To be even more ac- curate, we, for the first time, used human adipose tissue progenitor cells freshly isolated from overweight women. This experimental set-up enabled us to discriminate be- tween commitment and differentiation. Nevertheless, our explants being of natural origin, they present different physiological states that explains the high variability we observed. 5. Conclusions Due to the increased incidence of obesity related health disorders, processes underlying adipose tissue biology have been investigated intensively in recent years. In addition, search for ingredients acting on different stages of adipocytes’ life cycle has been pursued. In this context, we analysed the effect of a Celosia cristata extract on commitment and differentiation of progenitor cells from human subcutaneous adipose tissue. Results demonstrate that the extract decreases lipid con- tent and expression of adipogenesis-related genes. The impact of the extract is more pronounced when cells are treated prior to differentiation. It is suggested that Celo- sia cristata extract impact predominantly the commit- ment of human adipose tissue progenitor cells. 6. Authors’ Contributions ASD identified the potential activity of the Celosia cristata extract. RF participated in the design of experi- ments and their coordination. DE performed the experi- ments. With RF, he assisted with interpretation of the results. RF and KV drafted the manuscript. All authors read and approved the final manuscript. 7. Acknowledgements The authors thank Silab (Brive, France) for making the Celosia cristata available. REFERENCES [1] T. Tchkonia, T. Thomou, Y. Zhu, I. Karagiannides, C. Pothoulakis, M. D. Jensen and J. L. Kirkland, “Mecha- nisms and Metabolic Implications of Regional Differ- ences among Fat Depots,” Cell Metabolism, Vol. 17, No. 5, 2013, pp. 644-656. http://dx.doi.org/10.1016/j.cmet.2013.03.008 [2] A. Miranville, C. Heeschen, C. Sengenès, C. A. Curat, R. Busse and A. Bouloumié, “Improvement of Postnatal Ne- ovascularization by Human Adipose Tissue-Derived Stem Cells,” Circulation, Vol. 110, No. 3, 2004, pp. 349-355. http://dx.doi.org/10.1161/01.CIR.0000135466.16823.D0 [3] C. Sengenès, K. Lolmède, A. Zakaroff-Girard, R. Busse and A. Bouloumié, “Preadipocytes in the Human Subcu- taneous Adipose Tissue Display Distinct Features from the Adult Mesenchymal and Hematopoietic Stem Cells,” Journal of Cellular Physiology, Vol. 205, No. 1, 2005, pp. 114-122. http://dx.doi.org/10.1002/jcp.20381 [4] J. M. Gimble, B. A. Bunnell, E. S. Chiu and F. Guilak, “Concise Review: Adipose-Derived Stromal Vascular Frac- tion Cells and Stem Cells: Let’s Not Get Lost in Transla- tion,” Stem Cells, Vol. 29, No. 5, 2011, pp. 749-754. http://dx.doi.org/10.1002/stem.629 [5] A. Cignarelli, S. Perrini, R. Ficarella, A. Peschechera, P. Nigro and F. Giorgino, “Human Adipose Tissue Stem Cells: Relevance in the Pathophysiology of Obesity and Metabolic Diseases and Therapeutic Applications,” Ex- pert Reviews in Molecular Medicine, Vol. 14, 2012, p. e19. http://dx.doi.org/10.1017/erm.2012.13 [6] J. K. Fraser, R. Schreiber, B. Strem, M. Zhu, Z. Alfonso, I. Wulur and M. H. Hedrick, “Plasticity of Human Adi- pose Stem Cells toward Endothelial Cells and Cardio- myocytes,” Nature Clinical Practice Cardiovascular Medi- cine, Vol. 3, Suppl. 1, 2006, pp. S33-S37. http://dx.doi.org/10.1038/ncpcardio0444 [7] P. A. Zuk, M. Zhu, P. Ashjian, D. A. De Ugarte, J. I. Huang, H. Mizuno, Z. C. Alfonso, J. K. Fraser, P. Ben- haim and M. H. Hedrick, “Human Adipose Tissue Is a Source of Multipotent Stem Cells,” Molecular Biology of the Cell, Vol. 13, No. 12, 2002, pp. 4279-4295. http://dx.doi.org/10.1091/mbc.E02-02-0105 [8] Y. D. Halvorsen, D. Franklin, A. L. Bond, D. C. Hitt, C. Auchter, A. L. Boskey, E. P. Paschalis, W. O. Wilkison and J. M. Gimble, “Extracellular Matrix Mineralization and Osteoblast Gene Expression by Human Adipose Tis- sue-Derived Stromal Cells,” Tissue Engineering, Vol. 7, No. 6, 2001, pp. 729-741. http://dx.doi.org/10.1089/107632701753337681 [9] K. L. Spalding, E. Arner, P. O. Westermark, S. Bernard, B. A. Buchholz, O. Bergmann, L. Blomqvist, J. Hoffstedt, E. Naslund, T. Britton, H. Concha, M. Hassan, M. Ryden, J. Frisen and P. Arner, “Dynamics of Fat Cell Turnover in Humans,” Nature, Vol. 453, No. 7196, 2008, pp. 783- 787. http://dx.doi.org/10.1038/nature06902 [10] Y. Macotela, B. Emanuelli, M. A. Mori, S. Gesta, T. J. Schulz, Y. H. Tseng and C. R. Kahn, “Intrinsic Differ- ences in Adipocyte Precursor Cells from Different White Fat Depots,” Diabetes, Vol. 61, No. 7, 2012, pp. 1691- 1699. http://dx.doi.org/10.2337/db11-1753 [11] E. D. Rosen and O. A. MacDougald, “Adipocyte Differ- entiation from the Inside Out,” Nature Reviews Molecular Cell Biology, Vol. 7, No. 12, 2006, pp. 885-896. http://dx.doi.org/10.1038/nrm2066 [12] Q. Q. Tang and M. D. Lane, “Adipogenesis: From Stem Cell to Adipocyte,” Annual Review of Biochemistry, Vol. 81, No. 1, 2012, pp. 715-736. http://dx.doi.org/10.1146/annurev-biochem-052110-1157 18 [13] J. Söhle, A. Knott, U. Holtzmann, R. Siegner, E. Grönni- Open Access JCDSA
 Impact of Celosia cristata Extract on Adipogenesis of Native Human CD34+/CD31− Cells Open Access JCDSA 63 ger, A. Schepky, S. Gallinat, H. Wenck, F. Stäb and M. Winnefeld, “White Tea Extract Induces Lipolytic Activity and Inhibits Adipogenesis in Human Subcutaneous (Pre)- Adipocytes,” Nutrition & Metabolism, Vol. 6, No. 1, 2009, p. 20. http://dx.doi.org/10.1186/1743-7075-6-20 [14] S. Rayalam, M. A. Della-Fera and C. A. Baile, “Phyto- chemicals and Regulation of the Adipocyte Life Cycle,” Journal of Nutritional Biochemistry, Vol. 19, No. 11, 2008, pp. 717-726. http://dx.doi.org/10.1016/j.jnutbio.2007.12.007 [15] N. Gooda Sahib, N. Saari, A. Ismail, A. Khatib, F. Ma- homoodally and A. Abdul Hamid, “Plants’ Metabolites as Potential Antiobesity Agents,” The Scientific World Jour- nal, 2012, Article ID: 436039. [16] R. Ratnawati, M. R. Indra and A. Satuman, “Epigallo- catechin Gallate of Green Tea Inhibits Proliferation, Dif- ferentiation and TNF-α in the Primary Human Visceral Preadipocytes Culture,” Majalah Llmu Faal Indonesia, Vol. 6, No. 3, 2007, pp. 160-168. [17] P. Tontonoz and B. M. Spiegelman, “Fat and Beyond: The Diverse Biology of PPARgamma,” Annual Review of Biochemistry, Vol. 77, 2008, pp. 289-312. http://dx.doi.org/10.1146/annurev.biochem.77.061307.09 1829 [18] R. Siersbaek, R. Nielsen and S. Mandrup, “PPARgamma in Adipocyte Differentiation and Metabolism—Novel In- sights from Genome-Wide Studies,” FEBS Letters, Vol. 584, No. 15, 2010, pp. 3242-3249. http://dx.doi.org/10.1016/j.febslet.2010.06.010 [19] Z. Dudhia, J. Louw, C. Muller, E. Joubert, D. de Beer, C. Kinnear and C. Pheiffer, “Cyclopia maculata and Cyclo- pia subternata (Honeybush Tea) Inhibits Adipogenesis in 3T3-L1 Pre-Adipocytes,” Phytomedicine, Vol. 20, No. 5, 2013, pp. 401-408. http://dx.doi.org/10.1016/j.phymed.2012.12.002 [20] C. H. Jung, S. J. Jang, J. Ahn, S. Y. Gwon, T. I. Jeon, T. W. Kim and T. Y. Ha, “Alpinia officinarum Inhibits Adi- pocyte Differentiation and High-Fat Diet-Induced Obesity in Mice through Regulation of Adipogenesis and Lipo- genesis,” Journal of Medicinal Food, Vol. 15, No. 11, 2012, pp. 959-967. http://dx.doi.org/10.1089/jmf.2012.2286 [21] U. H. Park, J. C. Jeong, J. S. Jang, M. R. Sung, H. Youn, S. J. Lee, E. J. Kim and S. J. Um, “Negative Regulation of Adipogenesis by Kaempferol, a Component of Rhi- zoma Polygonati falcatum in 3T3-L1 Cells,” Biological & Pharmaceutical Bulletin, Vol. 35, No. 9, 2012, pp. 1525- 1533. [22] S. P. Poulos, M. V. Dodson and G. J. Hausman, “Cell Line Models for Differentiation: Preadipocytes and Adi- pocytes,” Experimental Biology and Medicine, Vol. 235, No. 10, 2010, pp. 1185-1193. http://dx.doi.org/10.1258/ebm.2010.010063
|