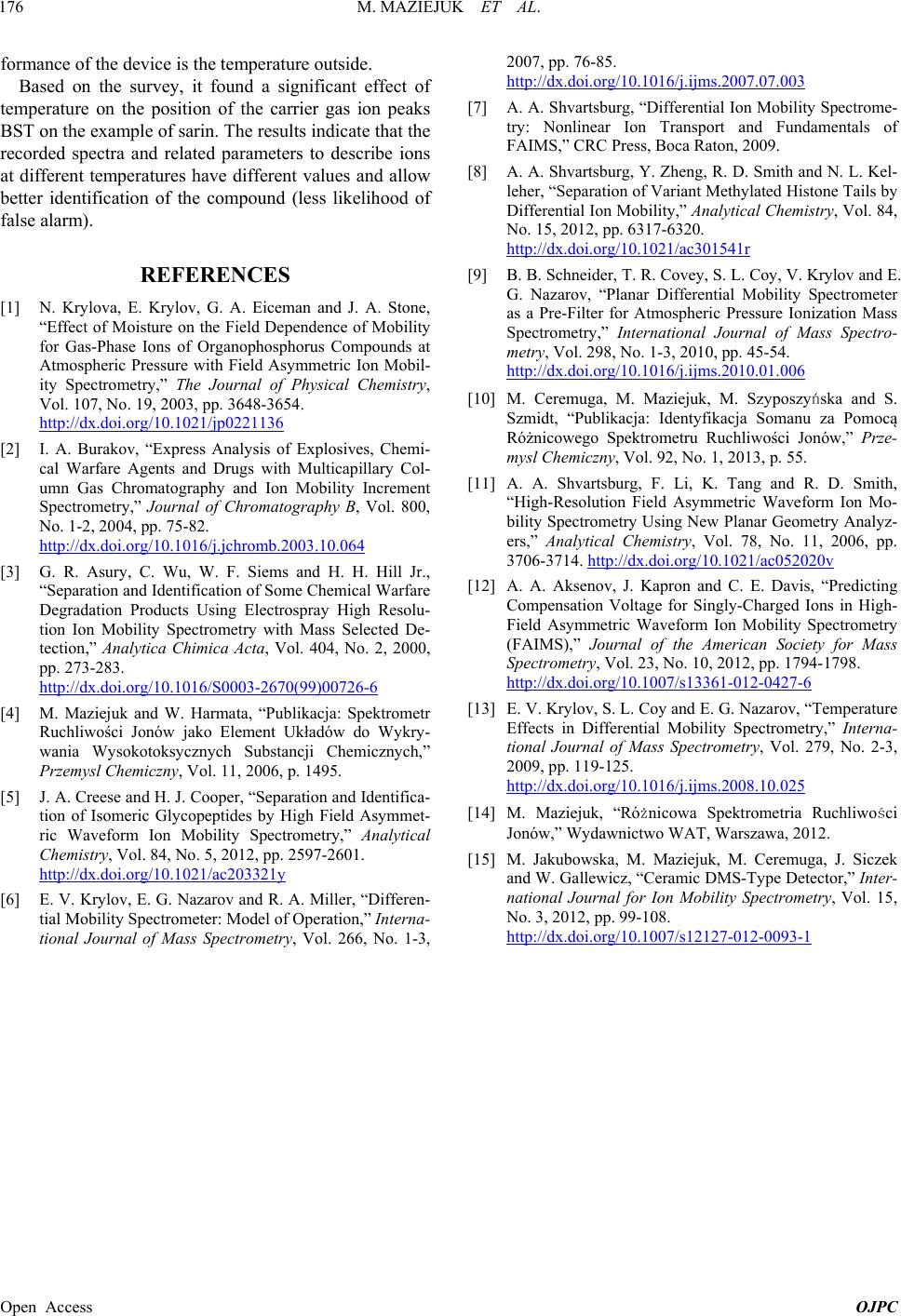
M. MAZIEJUK ET AL.
Open Access OJPC
176
formance of the device is the temperature outside.
Based on the survey, it found a significant effect of
temperature on the position of the carrier gas ion peaks
BST on the example of sarin. The results indicate that the
recorded spectra and related parameters to describe ions
at different temperatures have different values and allow
better identification of the compound (less likelihood of
false alarm).
REFERENCES
[1] N. Krylova, E. Krylov, G. A. Eiceman and J. A. Stone,
“Effect of Moisture on the Field Dependence of Mobility
for Gas-Phase Ions of Organophosphorus Compounds at
Atmospheric Pressure with Field Asymmetric Ion Mobil-
ity Spectrometry,” The Journal of Physical Chemistry,
Vol. 107, No. 19, 2003, pp. 3648-3654.
http://dx.doi.org/10.1021/jp0221136
[2] I. A. Burakov, “Express Analysis of Explosives, Chemi-
cal Warfare Agents and Drugs with Multicapillary Col-
umn Gas Chromatography and Ion Mobility Increment
Spectrometry,” Journal of Chromatography B, Vol. 800,
No. 1-2, 2004, pp. 75-82.
http://dx.doi.org/10.1016/j.jchromb.2003.10.064
[3] G. R. Asury, C. Wu, W. F. Siems and H. H. Hill Jr.,
“Separation and Identification of Some Chemical Warfare
Degradation Products Using Electrospray High Resolu-
tion Ion Mobility Spectrometry with Mass Selected De-
tection,” Analytica Chimica Acta, Vol. 404, No. 2, 2000,
pp. 273-283.
http://dx.doi.org/10.1016/S0003-2670(99)00726-6
] M. Maziejuk and W. H
ykry-
[4 armata, “Publikacja: Spektrometr
Ruchliwości Jonów jako Element Układów do W
wania Wysokotoksycznych Substancji Chemicznych,”
Przemysl Chemiczny, Vol. 11, 2006, p. 1495.
[5] J. A. Creese and H. J. Cooper, “Separation and Identifica-
tion of Isomeric Glycopeptides by High Field Asymmet-
ric Waveform Ion Mobility Spectrometry,” Analytical
Chemistry, Vol. 84, No. 5, 2012, pp. 2597-2601.
http://dx.doi.org/10.1021/ac203321y
[6] E. V. Krylov, E. G. Nazarov and R. A. Miller, “Differen-
tial Mobility Spectrometer: Model of Operation,” Interna-
tional JournalVol. 266, No. 1-3
2007, pp. 76-85.
http://dx.doi.org/10.1016/j.ijms.2007.07.003
of Mass Spectrometry, ,
[7] A. A. Shvartsburg, “Differential Ion Mobility Spectrome-
try: Nonlinear Ion Transport and Fundamentals of
FAIMS,” CRC Press, Boca Raton, 2009.
[8] A. A. Shvartsburg, Y. Zheng, R. D. Smith and N. L. Kel-
leher, “Separation of Variant Methylated Histone Tails by
Differential Ion Mobility,” Analytical Chemistry, Vol. 84,
No. 15, 2012, pp. 6317-6320.
http://dx.doi.org/10.1021/ac301541r
[9] B. B. Schneider, T. R. Covey, S. L. Coy, V. Krylov and E.
G. Nazarov, “Planar Differential Mobility Spectrometer
as a Pre-Filter for Atmospheric Pressure Ionization Mass
Spectrometry,” International Journal of Mass Spectro-
metry, Vol. 298, No. 1-3, 2010, pp. 45-54.
http://dx.doi.org/10.1016/j.ijms.2010.01.006
[10] M. Ceremuga, M. Maziejuk, M. Szyposzyńska and S.
Szmidt, “Publikacja: Identyfikacja Somanu za Pomocą
Różnicowego Spektrometru Ruchliwości Jonów,” Prze-
mysl Chemiczny, Vol. 92, No. 1, 2013, p. 55.
[11] A. A. Shvartsburg, F. Li, K. Tang and R. D. Smith,
“High-Resolution Field Asymmetric Waveform Ion Mo-
bility Spectrometry Using New Planar Geometry Analyz-
ers,” Analytical Chemistry, Vol. 78, No. 11, 2006, pp.
3706-3714. http://dx.doi.org/10.1021/ac052020v
[12] A. A. Aksenov, J. Kapron and C. E. Davis, “Predicting
Compensation Voltage for Singly-Charged Ions in High-
Field Asymmetric Waveform Ion Mobility Spectrometry
(FAIMS),” Journal of the American Society for Mass
Spectrometry, Vol. 23, No. 10, 2012, pp. 1794-1798.
http://dx.doi.org/10.1007/s13361-012-0427-6
E. G. Nazarov, “Temperature [13] E. V. Krylov, S. L. Coy and
Effects in Differential Mobility Spectrometry,” Interna-
tional Journal of Mass Spectrometry, Vol. 279, No. 2-3,
2009, pp. 119-125.
http://dx.doi.org/10.1016/j.ijms.2008.10.025
[14] M. Maziejuk,
Jonów,” Wydawnictwo WAT, Warszawa, 2012.
“Różnicowa Spektrometria Ruchliwości
[15] M. Jakubowska, M. Maziejuk, M. Ceremuga, J. Siczek
and W. Gallewicz, “Ceramic DMS-Type Detector,” Inter-
national Journal for Ion Mobility Spectrometry, Vol. 15,
No. 3, 2012, pp. 99-108.
http://dx.doi.org/10.1007/s12127-012-0093-1