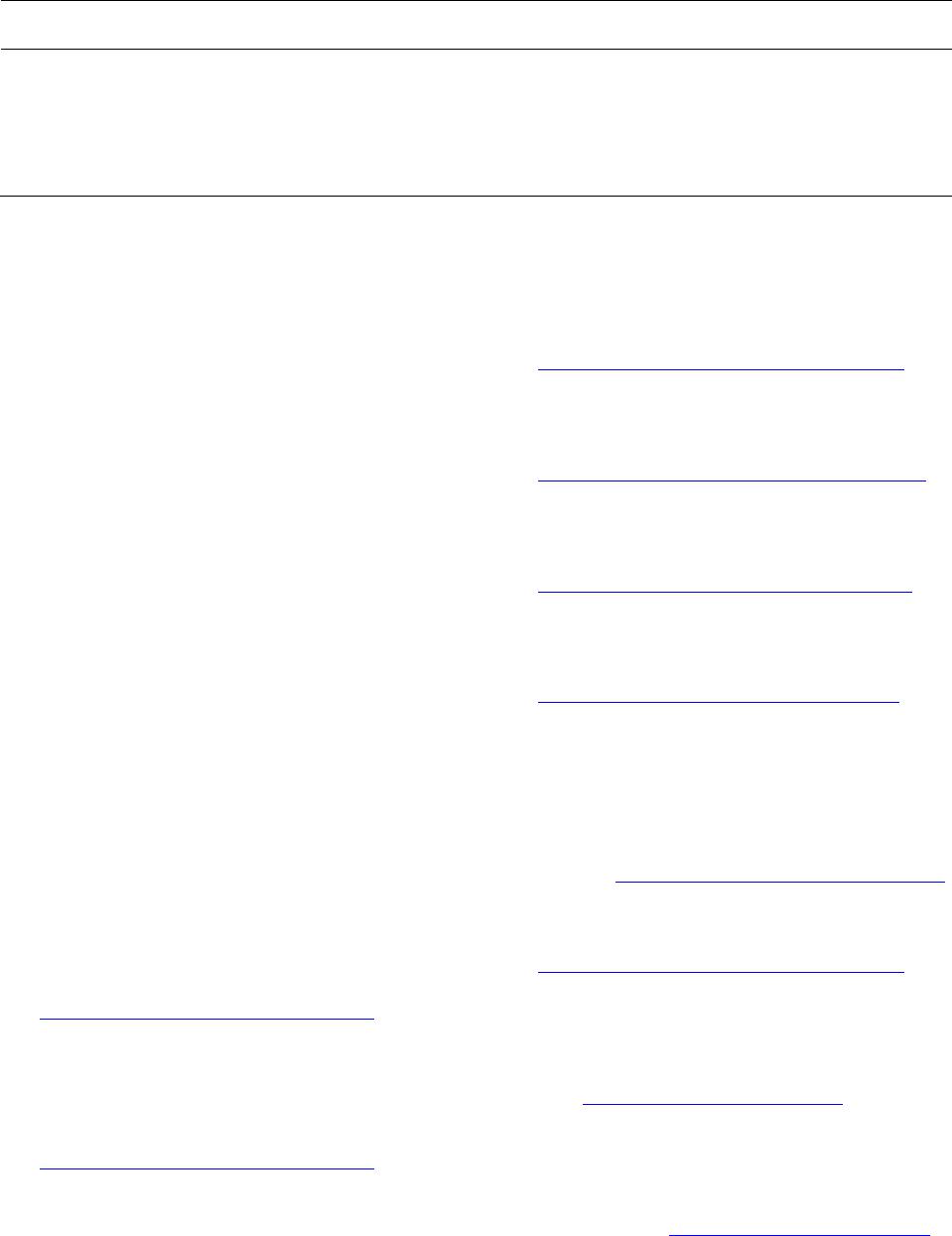
P. L. LENG ET AL.
Open Access ANP
382
na magnetic
Ni0.25Zn0.75Fe2O4 Calcinations Average particle Average particle Saturation magnetization,Coercivity Peak to peak line factorResonance
Table 1. The average particle sizes (nm) of Ni0.25Zn0.75Fe2O4oparticles determined from XRD and TEM andn
properties observed from VSM and EPR at room temperature.
nanoparticles temperature (K) size XRD (nm) size TEM (nm)Ms (emu/g) field Hc (G)width ΔHpp (G) g- field, Hr (G)
Ni-2.0670Zn ferrite 1 723 9 7 11.414 7 758 3170
Ni-Zn ferrite 2 773 11 9 15.785 10 755 2.08853140
Ni-Zn ferrite 3 823 14 13 20.320 26 753 2.10503118
Ni-Zn ferrite 4 873 23 20 26.447 29 750 2.12203090
nthesis of Ni0.25Zn0.75Fe2O4 nanocrystals with grain
by the Ministry of Higher
[1] A. Goldman, “logy,” 2nd Edition,
6/j.jmmm.2006.02.031
sy
size ranging from 7 to 25 nm at the calcination tempera-
tures from 723 to 873 K as measured by XRD and TEM.
The PVP stabilized the particles and prevented them
from agglomerating. The FTIR measurement confirmed
the removal of all organic matters and leaving pure metal
oxides at 873 K. The VSM results showed that the satu-
ration magnetization increased from 11 emu/g to 26 emu/
g and the coercivity value increased from 7 to 29 G at
calcination temperatures from 723 K to 873 K due to the
increasing of the volume of single domain grains. The
values of g-factor increases with increase of calcination
temperature and particle size were increased. This simple
method, which is cost-effective and environmentally
friendly, produces no toxic byproduct effluents and can
be used to fabricate pure, crystalline spinel ferrite nano-
crystals.
5. Acknowledgements
This work was supported
Education of Malaysia under the FRGS grant. The au-
thors would also like to thank staff of the Faculty of Sci-
ence and the Bioscience Institute of University Putra
Malaysia, who had contributed to this work.
REFERENCES
Modern Ferrite Techno
Springer Science Business Media, Inc., New York, 2006.
[2] S. W. Lee and C. S. Kim, “Superparamagnetic Properties
Ni-Zn Ferrite for Nano-Bio Fusion Applications,” Jour-
nal of Magnetism and Magnetic Materials, Vol. 304, No.
1, 2006, pp. 418-420.
http://dx.doi.org/10.101
assa[3] G. R. Amiri, M. H. Yousefi, M. R. Abolhni, S.
Manouchehri, M. H. Keshavarz and S. Fatahian, “Mag-
netic Properties and Microwave Absorption in Ni-Zn and
Mn-Zn Ferrite Nanoparticles Synthesized by Low-Tem-
perature Solid-State Reaction,” Journal of Magnetism and
Magnetic Materials, Vol. 323, No. 6, 2011, pp. 730-734.
http://dx.doi.org/10.1016/j.jmmm.2010.10.034
[4] M. Mohapatra and S. Anand, “Synthesis and Applications
nology, Vol. 2, No. 8, 2010, pp. 127-146.
anodimensional
of Nano-Structured Iron Oxides/Hydroxides—A Review,”
International Journal of Engineering, Science and Tech-
[5] E. Manova, D. Paneva, B. Kunev, E. Rivière, C. Estour-
nès and I. Mitov, “Characterization of N
Ni-Zn Ferrite Prepared by Mechanochemical and Thermal
Methods,” Journal of Physics: Conference Series, Vol.
217, No. 1, 2010, Article ID: 012102.
http://dx.doi.org/10.1088/1742-6596/217/1/012102
[6] K. Velmurugan, V. S. K. Venkatachalapathy and S.
hilnathan, “Synthesis of Nickel Zinc Iron Nanopar
Send-
ticles
by Coprecipitation Technique,” Materials Research, Vol.
13, No. 3, 2010, pp. 299-303.
http://dx.doi.org/10.1590/S1516-14392010000300005
[7] B. T. Naughton, P. Majewski and D. R. Clarke, “Mag-
netic Properties of Nickel-Zinc Ferrite Toroids Prepar
ed
from Nanoparticles,” Journal of the American Ceramic
Society, Vol. 90, No. 11, 2007, pp. 3547-3553.
http://dx.doi.org/10.1111/j.1551-2916.2007.01981.x
[8] E. E. Sileo, R. Rotelo and S. E. Jacobo, “Nickel
Ferrites Prepared by the Citrate Precursor Method,”
Zinc
Phy-
sica B: Condensed Matter, Vol. 320, No. 1-4, 2002, pp.
257-260.
http://dx.doi.org/10.1016/S0921-4526(02)00705-6
[9] R. K. Singh, C. Upadhyay, S. Layek and A. Yadav
“Cation Distribution of NiZnFe O Nanopart
,
icles,”
ed Nickel Zinc
0.50.524
International Journal of Engineering, Science and Tech-
nology, Vol. 2, No. 8, 2010, pp. 104-109.
[10] A. Kumar, Annveer, M. Arora, M. S. Yadav and R. P.
Panta, “Induced Size Effect on Ni Dop
Ferrite Nanoparticles,” Physics Procedia, Vol. 9, 2010,
pp. 20-23. http://dx.doi.org/10.1016/j.phpro.2010.11.006
[11] M. Shigeta and A. B. Murphy, “Thermal Plasmas for
Nanofabrication,” Journal of Physics D: Applied Physics,
Vol. 44, No. 17, 2011, Article ID: 174025.
http://dx.doi.org/10.1088/0022-3727/44/17/174025
[12] S. A. Morrison, C. L. Cahill, E. E. Carpenter, S. Calvin
Swaminathan, M. E. McHenry and V. G. Harris, “
, R.
Mag-
netic and Structural Properties of Nickel Zinc Ferrite
Nanoparticles Synthesized at Room Temperature,” Jour-
nal of Applied Physics, Vol. 95, No. 11, 2004, Article ID:
6392. http://dx.doi.org/10.1063/1.1715132
[13] S. Thakur, S. C. Katyal, A. Gupta, V. R. Reddy, S. K.
Sharma, M. Knobel and M. Singh, “Nickel-Zinc Ferrite
from Reverse Micelle Process: Structural and Magnetic
Properties, Mössbauer Spectroscopy Characterization,”
Journal of Physical Chemistry C, Vol. 113, No. 49, 2009,
pp. 20785-20794. http://dx.doi.org/10.1021/jp9050287