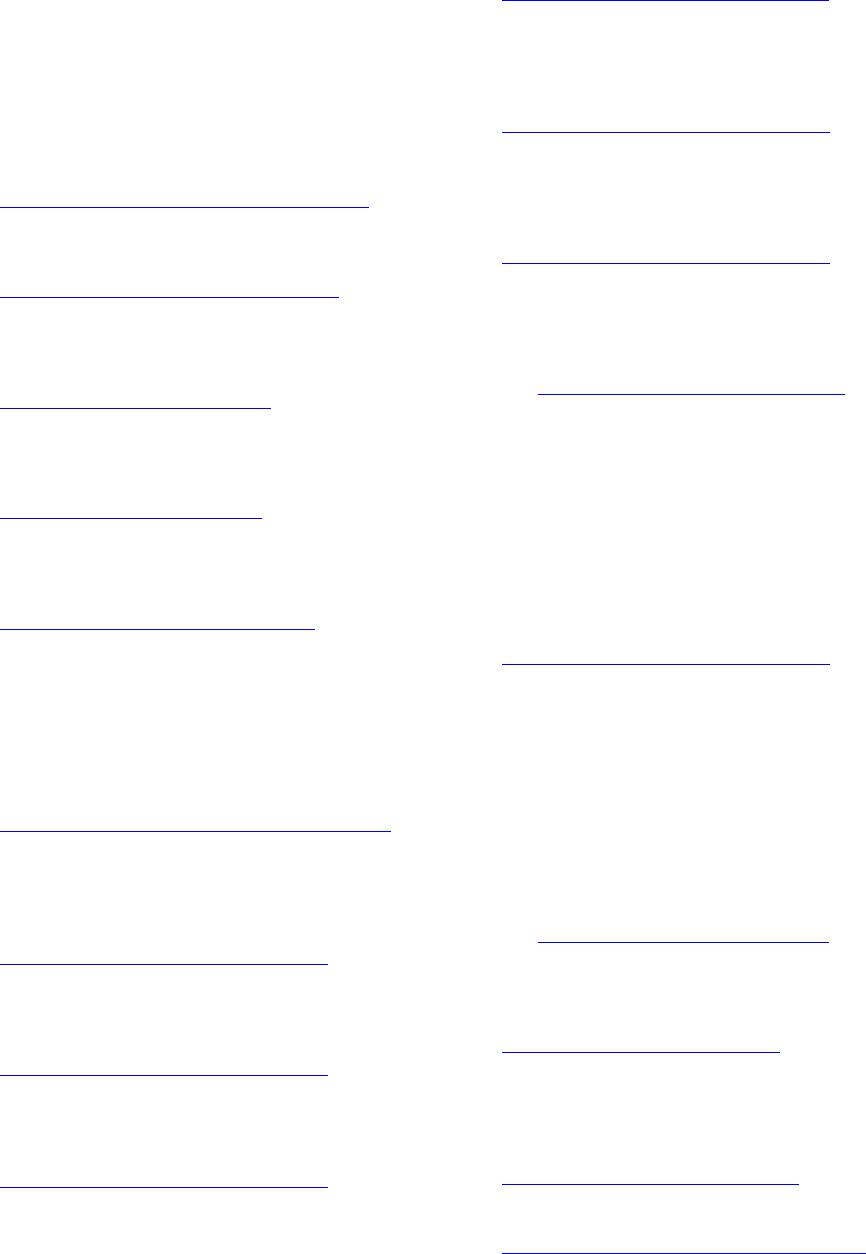
H. NORITOMI ET AL.
370
application such as the extraction.
REFERENCES
[1] P. Kumar and K. L. Mittal, “Handbook of Microemulsion
Science and Technology,” CRC Press, New York, 1999.
[2] K. Tonova and Z. Lazarova, “Reversed Micelle Solvents
as Tools of Enzyme Purification and Enzyme-Catalyzed
Conversion,” Biotechnology Advances, Vol. 26, No. 6,
2008, pp. 516-532.
http://dx.doi.org/10.1016/j.biotechadv.2008.06.002
[3] P. Barnickel and A. Wokaun, “Synthesis of Metal Col-
loids in Inverse Microemulsions,” Molecular Physics,
Vol. 69, No. 1, 1990, pp. 1-9.
http://dx.doi.org/10.1080/00268979000100011
[4] A. Taleb, C. Petit and M. P. Pileni, “Synthesis of Highly
Monodisperse Silver Nanoparticles from AOT Reverse
Micelles: A Way to 2D and 3D Self-Organization,”
Chemistry of Materials, Vol. 9, No. 4, 1997, pp. 950-959.
http://dx.doi.org/10.1021/cm960513y
[5] R. P. Bagwe and K. C. Khilar, “Effects of Intermicellar
Exchange Rate on the Formation of Silver Nanoparticles
in Reverse Microemulsions of AOT,” Langmuir, Vol. 16,
No. 3, 2000, pp. 905-910.
http://dx.doi.org/10.1021/la980248q
[6] W. Zhang, X. Qiao, J. Chen and H. Wang, “Preparation
of Silver Nanoparticles in Water-in-Oil AOT Reverse
Micelles,” Journal of Colloid and Interface Science, Vol.
302, No. 1, 2006, pp. 370-373.
http://dx.doi.org/10.1016/j.jcis.2006.06.035
[7] Y. Okumura, “Organic Solvents and Surfactants for Tox-
icity Test Using Aquatic Organisms and Their Acceptable
Concentrations,” Bulletin of the National Research Insti-
tute of Fisheries Science, Vol. 11, No. 11, 1998, pp. 113-
134.
[8] P. D. Abel, “Toxicity of Synthetic Detergents to Fish and
Aquatic Invertebrates,” Journal of Fish Biology, Vol. 6,
No. 3, 1974, pp. 279-298.
http://dx.doi.org/10.1111/j.1095-8649.1974.tb04545.x
[9] H. Noritomi, S. Ito, N. Kojima, S. Kato and K. Nagahama,
“Forward and Backward Extractions of Cytochrome c
Using Reverse Micellar System of Sucrose Fatty Acid
Ester,” Colloid and Polymer Science, Vol. 284, No. 6,
2006, pp. 604-610.
http://dx.doi.org/10.1007/s00396-005-1398-y
[10] H. Noritomi, H. Kowata, N. Kojima, S. Kato and K. Na-
gahama, “Application of Sucrose Fatty Acid Ester to Re-
verse Micellar Extraction of Lysozyme,” Colloid and
Polymer Science, Vol. 284, No. 6, 2006, pp. 677-682.
http://dx.doi.org/10.1007/s00396-005-1419-x
[11] H. Noritomi, N. Kojima, S. Kato and K. Nagahama,
“How Can Temperature Affect Reverse Micellar Extrac-
tion Using Sucrose Fatty Acid Ester?” Colloid and Poly-
mer Science, Vol. 284, No. 6, 2006, pp. 683-687.
http://dx.doi.org/10.1007/s00396-005-1452-9
[12] H. Noritomi, T. Takasugi and S. Kato, “Refolding of
Denatured Lysozyme by Water-in-Oil Microemulsions of
Sucrose Fatty Acid Esters,” Biotechnology Letters, Vol.
30, No. 4, 2008, pp. 689-693.
http://dx.doi.org/10.1007/s10529-007-9587-z
[13] H. Noritomi, K. Kagitani, Y. Muratsubaki and S. Kato,
“Effect of Composition of Sucrose Fatty Acid Esters on
Formation of Palladium Nanoparticles in Reverse Mi-
celles,” Colloid and Polymer Science, Vol. 287, No. 7,
2009, pp. 795-799.
http://dx.doi.org/10.1007/s00396-009-2031-2
[14] H. Noritomi, N. Igari, K. Kagitani, Y. Umezawa, Y. Mu-
ratsubaki and S. Kato, “Synthesis and Size Control of
Silver Nanoparticles Using Reverse Micelles of Sucrose
Fatty Acid Esters,” Colloid and Polymer Science, Vol.
288, No. 8, 2010, pp. 887-891.
http://dx.doi.org/10.1007/s00396-010-2214-x
[15] H. Noritomi, Y. Umezawa, S. Miyagawa and S. Kato,
“Preparation of Highly Concentrated Silver Nanoparticles
in Reverse Micelles of Sucrose Fatty Acid Esters through
Solid-Liquid Extraction Method,” Advances in Chemical
Engineering and Science, Vol. 1, No. 4, 2011, pp. 299-
304. http://dx.doi.org/10.4236/aces.2011.14041
[16] H. Noritomi, “Preparation of Highly Concentrated Silver
Nanoparticles: Method Using Solid-Liquid Extraction by
Reverse Micelles,” LAP LAMBERT Academic Publish-
ing, Saarbrücken, 2012.
[17] N. Otomo, “Self-Organized Structures of Food Emulsifi-
ers and Their Applications,” Japan Journal of Food En-
gineering, Vol. 4, No. 1, 2003, pp. 1-9.
[18] H. Noritomi, S. Tamai, H. Saito and S. Kato, “Extraction
of Water Miscible Organic Dyes by Reverse Micelles of
Alkyl Glucosides,” Colloid and Polymer Science, Vol.
287, No. 4, 2009, pp. 455-459.
http://dx.doi.org/10.1007/s00396-008-1988-6
[19] H. Noritomi, S. Miyagawa, N. Igari, H. Saito and S. Kato,
“Application of Reverse Micelles of Alkyl Glucosides to
Synthesis of Silver Nanoparticles,” Advances in Nano-
particles, accepted.
[20] D. J. Shaw, “Introduction to Colloid and Surface Chemis-
try,” Butterworth-Heinemann, Oxford, 1992.
[21] J. N. Israelachvili, “Intermolecular and Surface Forces,”
Academic Press Ltd., London, 1985.
[22] D. J. Mitchell and B. W. Ninham, “Micelles, Vesicles,
and Microemulsions,” Journal of the Chemical Society,
Faraday Transactions 2, Vol. 77, No. 4, 1981, pp. 601-
629. http://dx.doi.org/10.1039/f29817700601
[23] T. Kawai, K. Hamada, N. Shindo and K. Kon-no, “For-
mation of AOT Reversed Micelles and W/O Microemul-
sions,” Bulletin of the Chemical Society of Japan, Vol. 65,
No. 10, 1992, pp. 2715-2719.
http://dx.doi.org/10.1246/bcsj.65.2715
[24] K. Kon-no, K. Morita, H. Nagasawa and T. Kawai,
“Solubilized States of Water and Formation of Reverse
Micelles of Homogeneous Dodecyl Polyethylene Glycol
Ethers in Cyclohexane,” Journal of Japan Oil Chemist’s
Society, Vol. 43, No. 6, 1994, pp. 490-494.
http://dx.doi.org/10.5650/jos1956.43.490
[25] W. F. Waugh, “Protein-Protein Interactions,” Advances in
Protein Chemistry, Vol. 9, 1954, pp. 325-437.
http://dx.doi.org/10.1016/S0065-3233(08)60210-7
Open Access ANP