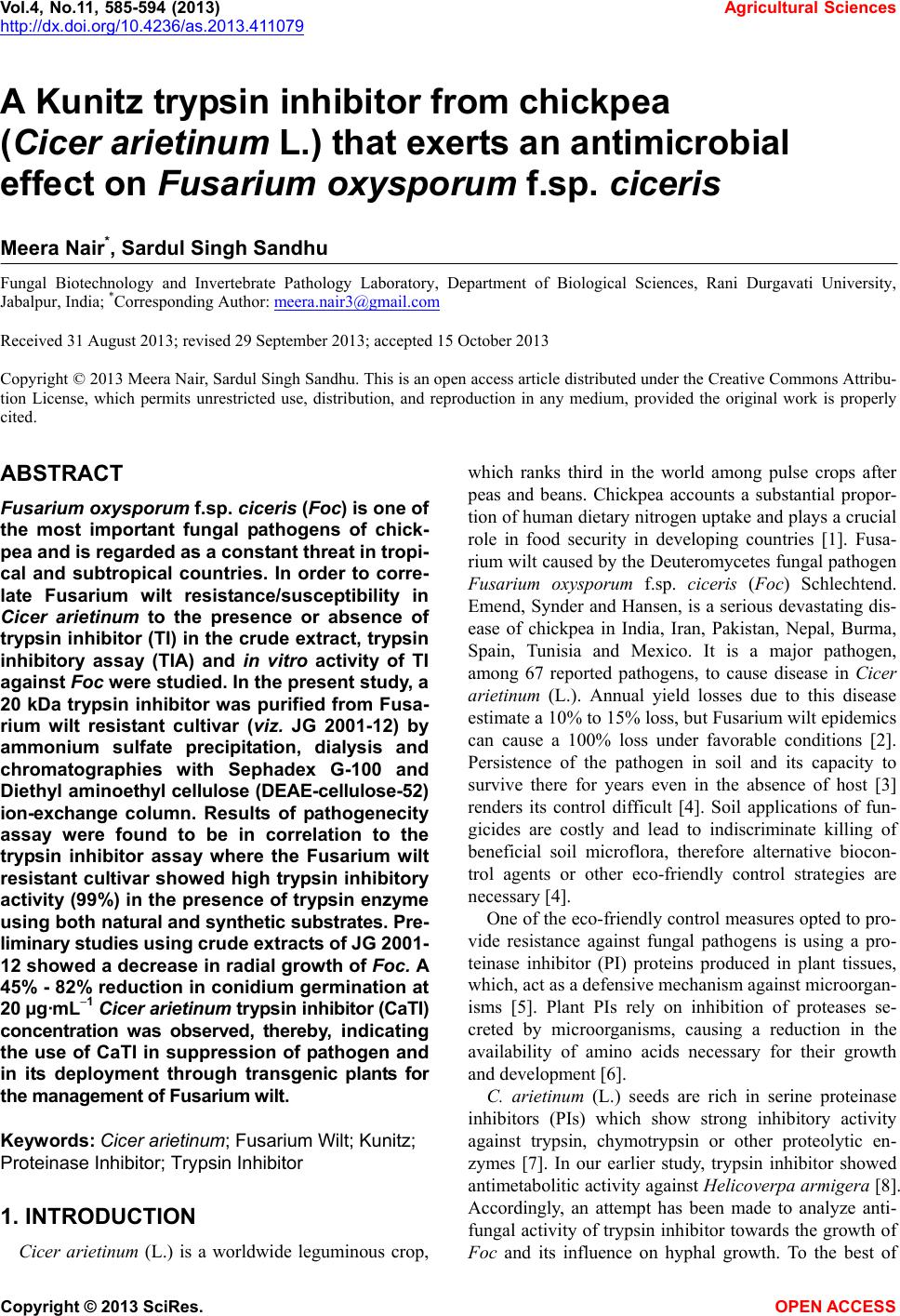 Vol.4, No.11, 585-594 (2013) Agricultural Sciences http://dx.doi.org/10.4236/as.2013.411079 A Kunitz trypsin inhibitor from chickpea (Cicer arietinum L.) that exerts an antimicrobial effect on Fusarium oxysporum f.s p. ciceris Meera Nair*, Sardul Singh Sandhu Fungal Biotechnology and Invertebrate Pathology Laboratory, Department of Biological Sciences, Rani Durgavati University, Jabalpur, India; *Corresponding Author: meera.nair3@gmail.com Received 31 August 2013; revised 29 September 2013; accepted 15 October 2013 Copyright © 2013 Meera Nair, Sardul Singh Sandhu. This is an open access article distributed under the Creative Commons Attribu- tion License, which permits unrestricted use, distribution, and reproduction in any medium, provided the original work is properly cited. ABSTRACT Fusarium oxysporum f.sp. ciceris (Foc) is one of the most important fungal pathogens of chick- pea and is re garded a s a c onstant threat in trop i- cal and subtropical countries. In order to corre- late Fusarium wilt resistance/susceptibility in Cicer arietinum to the presence or absence of trypsin inhibitor (TI) in the crude extract, trypsin inhibitory assay (TIA) and in vitro activity of TI against Foc were studied. In the present study, a 20 kDa trypsin inhibitor was purified from Fusa- rium wilt resistant cultivar (viz. JG 2001-12) by ammonium sulfate precipitation, dialysis and chromatographies with Sephadex G-100 and Die thyl ami noeth yl cellulose (DEAE-cellulose-52) ion-exchange column. Results of pathogenecity assay were found to be in correlation to the trypsin inhibitor assay where the Fusarium wilt resistant cultivar showed high trypsin inhibitory activity (99%) in the presence of trypsin enzyme using both natural and synthetic substrates. Pre- li m in a ry s tu d i es using crude extra ct s o f JG 200 1- 12 showed a decrease in radial growth of Foc. A 45% - 82% reduction in conidium germination at 20 µg·mL−1 Cicer arietinum trypsin inhibitor (CaTI) concentration was observed, thereby, indicating the use of CaTI in suppression of pathogen and in its deployment through transgenic plants for the management of Fusarium wilt. Keywords: Cicer arietinum; Fusarium Wilt; Kunitz; Proteinase Inhibitor; Trypsin Inhibitor 1. INTRODUCTION Cicer arietinum (L.) is a worldwide leguminous crop, which ranks third in the world among pulse crops after peas and beans. Chickpea accounts a substantial propor- tion of human dietary nitrogen uptake and plays a crucial role in food security in developing countries [1]. Fusa- rium wilt caused by the Deuteromycetes fungal pathogen Fusarium oxysporum f.sp. ciceris (Foc) Schlechtend. Emend, Synder and Hansen, is a serious devastating dis- ease of chickpea in India, Iran, Pakistan, Nepal, Burma, Spain, Tunisia and Mexico. It is a major pathogen, among 67 reported pathogens, to cause disease in Cicer arietinum (L.). Annual yield losses due to this disease estimate a 10% to 15% loss, but Fusarium wilt epidemics can cause a 100% loss under favorable conditions [2]. Persistence of the pathogen in soil and its capacity to survive there for years even in the absence of host [3] renders its control difficult [4]. Soil applications of fun- gicides are costly and lead to indiscriminate killing of beneficial soil microflora, therefore alternative biocon- trol agents or other eco-friendly control strategies are necessary [4]. One of the eco-friendly control measures opted to pro- vide resistance against fungal pathogens is using a pro- teinase inhibitor (PI) proteins produced in plant tissues, which, act as a defensive mechanism against microorgan- isms [5]. Plant PIs rely on inhibition of proteases se- creted by microorganisms, causing a reduction in the availability of amino acids necessary for their growth and development [6]. C. arietinum (L.) seeds are rich in serine proteinase inhibitors (PIs) which show strong inhibitory activity against trypsin, chymotrypsin or other proteolytic en- zymes [7]. In our earlier study, trypsin inhibitor showed antimetabolitic activity against Helicoverpa armigera [8]. Accordingly, an attempt has been made to analyze anti- fungal activity of trypsin inhibitor towards the growth of Foc and its influence on hyphal growth. To the best of Copyright © 2013 SciRes. OPEN ACCE SS
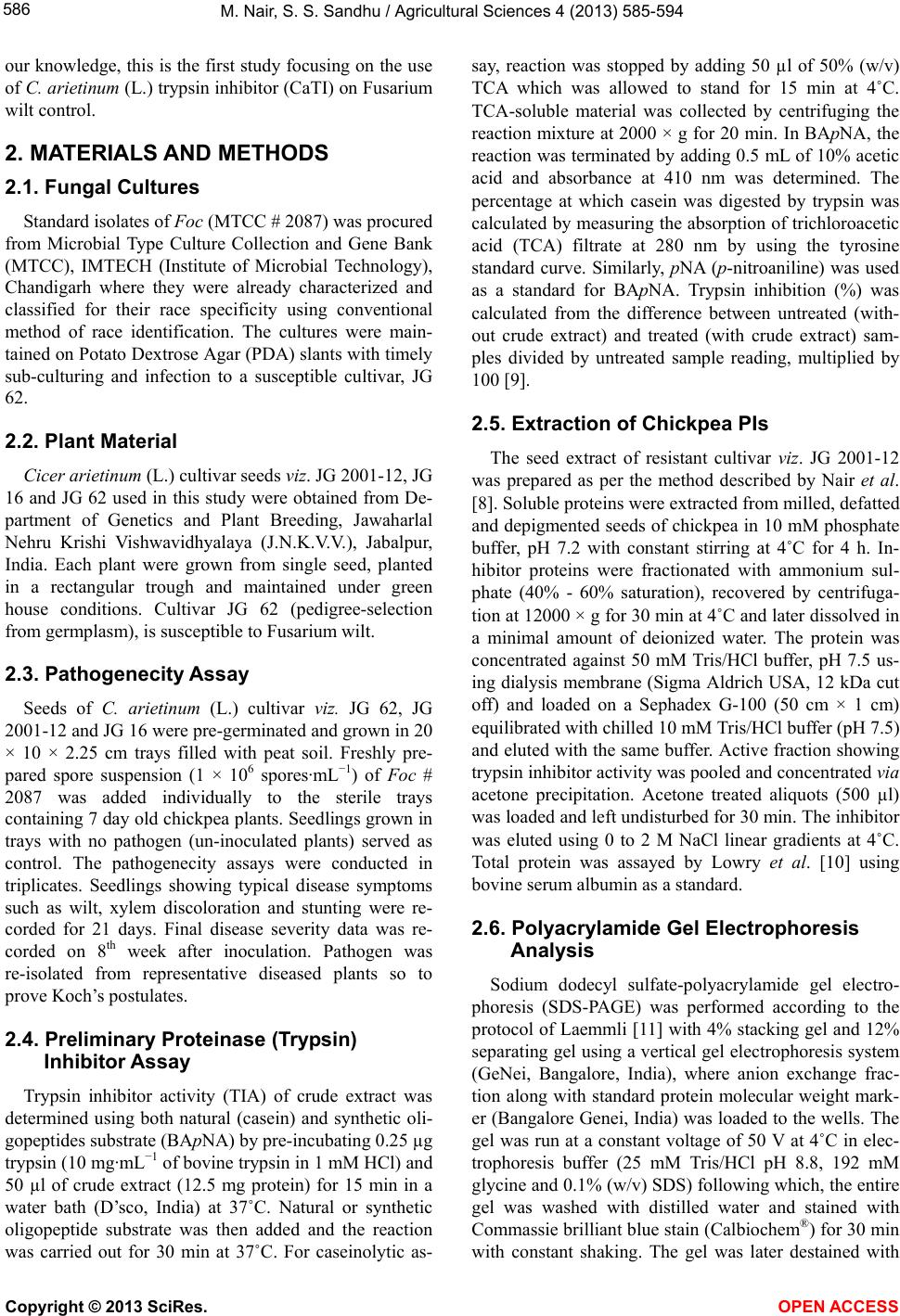 M. Nair, S. S. Sandhu / Agricultural Sciences 4 (2013) 585-594 586 our knowledge, this is the first study focusing on the use of C. arietinum (L.) trypsin inhibitor (CaTI) on Fusarium wilt control. 2. MATERIALS AND METHODS 2.1. Fungal Cultures Standard isolates of Foc (MTCC # 2087) was procured from Microbial Type Culture Collection and Gene Bank (MTCC), IMTECH (Institute of Microbial Technology), Chandigarh where they were already characterized and classified for their race specificity using conventional method of race identification. The cultures were main- tained on Potato Dextrose Agar (PDA) slants with timely sub-culturing and infection to a susceptible cultivar, JG 62. 2.2. Plant Material Cicer arietinum (L.) cultivar seeds viz. JG 2001-12, JG 16 and JG 62 used in this study were obtained from De- partment of Genetics and Plant Breeding, Jawaharlal Nehru Krishi Vishwavidhyalaya (J.N.K.V.V.), Jabalpur, India. Each plant were grown from single seed, planted in a rectangular trough and maintained under green house conditions. Cultivar JG 62 (pedigree-selection from germplasm), is susceptible to Fusarium wilt. 2.3. Pathogenecity Assay Seeds of C. arietinum (L.) cultivar viz. JG 62, JG 2001-12 and JG 16 were pre-germinated and grown in 20 × 10 × 2.25 cm trays filled with peat soil. Freshly pre- pared spore suspension (1 × 106 spores·mL−1) of Foc # 2087 was added individually to the sterile trays containing 7 day old chickpea plants. Seedlings grown in trays with no pathogen (un-inoculated plants) served as control. The pathogenecity assays were conducted in triplicates. Seedlings showing typical disease symptoms such as wilt, xylem discoloration and stunting were re- corded for 21 days. Final disease severity data was re- corded on 8th week after inoculation. Pathogen was re-isolated from representative diseased plants so to prove Koch’s postulates. The seed extract of resistant cultivar viz. JG 2001-12 was prepared as per the method described by Nair et al. [8]. Soluble proteins were extracted from milled, defatted and depigmented seeds of chickpea in 10 mM phosphate buffer, pH 7.2 with constant stirring at 4˚C for 4 h. In- hibitor proteins were fractionated with ammonium sul- phate (40% - 60% saturation), recovered by centrifuga- tion at 12000 × g for 30 min at 4˚C and later dissolved in a minimal amount of deionized water. The protein was concentrated against 50 mM Tris/HCl buffer, pH 7.5 us- ing dialysis membrane (Sigma Aldrich USA, 12 kDa cut off) and loaded on a Sephadex G-100 (50 cm × 1 cm) equilibrated with chilled 10 mM Tris/HCl buffer (pH 7.5) and eluted with the same buffer. Active fraction showing trypsin inhibitor activity was pooled and concentrated via acetone precipitation. Acetone treated aliquots (500 µl) was loaded and left undisturbed for 30 min. The inhibitor was eluted using 0 to 2 M NaCl linear gradients at 4˚C. Total protein was assayed by Lowry et al. [10] using bovine serum albumin as a standard. 2.4. Preliminary Proteinase (Trypsin) Inhibitor Assay Trypsin inhibitor activity (TIA) of crude extract was determined using both natural (casein) and synthetic oli- gopeptides substrate (BApNA) by pre-incubating 0.25 µg trypsin (10 mg·mL−1 of bovine trypsin in 1 mM HCl) and 50 µl of crude extract (12.5 mg protein) for 15 min in a water bath (D’sco, India) at 37˚C. Natural or synthetic oligopeptide substrate was then added and the reaction was carried out for 30 min at 37˚C. For caseinolytic as- say, reaction was stopped by adding 50 µl of 50% (w/v) TCA which was allowed to stand for 15 min at 4˚C. TCA-soluble material was collected by centrifuging the reaction mixture at 2000 × g for 20 min. In BApNA, the reaction was terminated by adding 0.5 mL of 10% acetic acid and absorbance at 410 nm was determined. The percentage at which casein was digested by trypsin was calculated by measuring the absorption of trichloroacetic acid (TCA) filtrate at 280 nm by using the tyrosine standard curve. Similarly, pNA (p-nitroaniline) was used as a standard for BApNA. Trypsin inhibition (%) was calculated from the difference between untreated (with- out crude extract) and treated (with crude extract) sam- ples divided by untreated sample reading, multiplied by 100 [9]. 2.5. Extraction of Chickpea PIs 2.6. Polyacrylamide Gel Electrophoresis Analysis Sodium dodecyl sulfate-polyacrylamide gel electro- phoresis (SDS-PAGE) was performed according to the protocol of Laemmli [11] with 4% stacking gel and 12% separating gel using a vertical gel electrophoresis system (GeNei, Bangalore, India), where anion exchange frac- tion along with standard protein molecular weight mark- er (Bangalore Genei, India) was loaded to the wells. The gel was run at a constant voltage of 50 V at 4˚C in elec- trophoresis buffer (25 mM Tris/HCl pH 8.8, 192 mM glycine and 0.1% (w/v) SDS) following which, the entire gel was washed with distilled water and stained with Commassie brilliant blue stain (Calbiochem®) for 30 min with constant shaking. The gel was later destained with Copyright © 2013 SciRes. OPEN ACCE SS
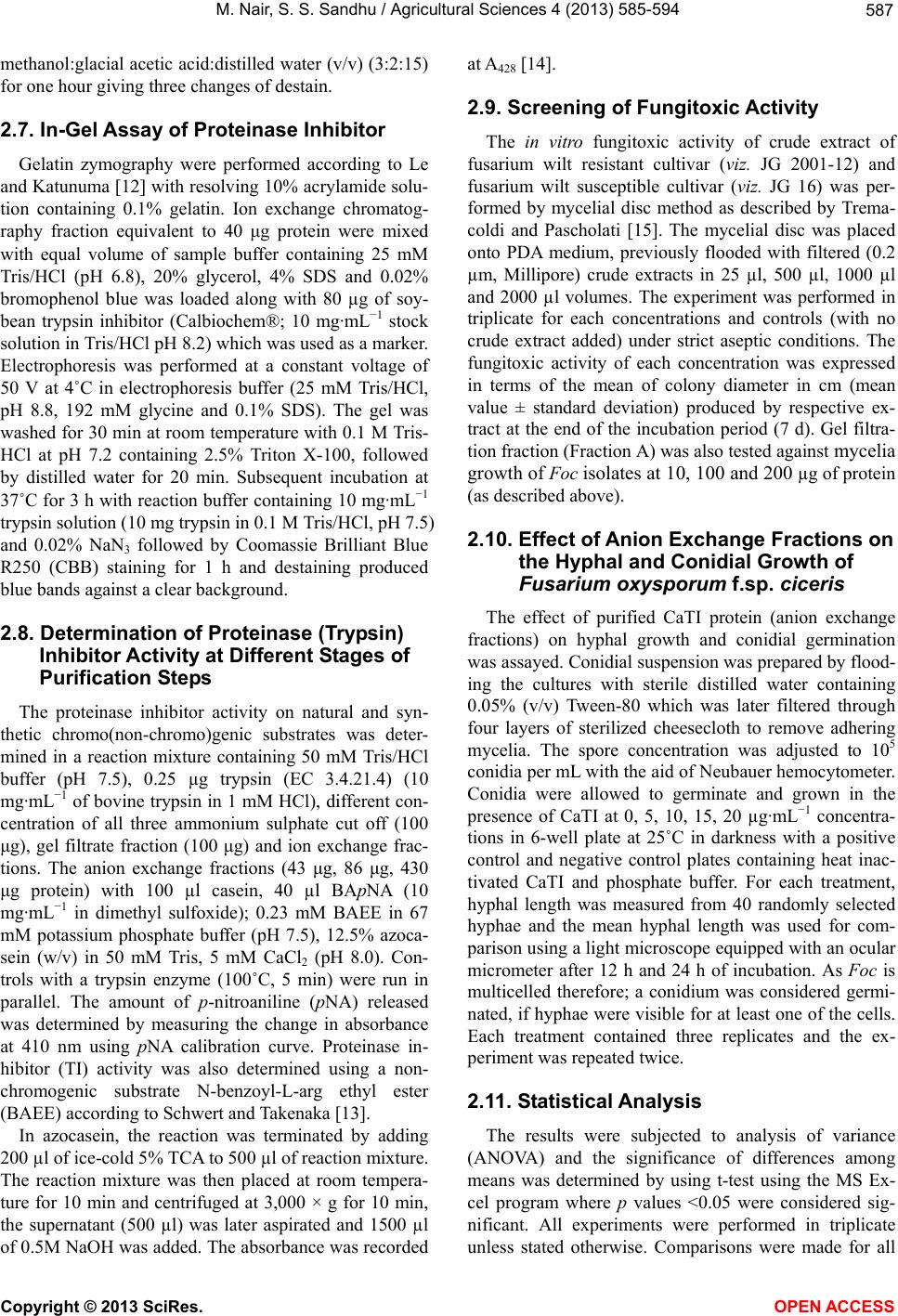 M. Nair, S. S. Sandhu / Agricultural Sciences 4 (2013) 585-594 587 methanol:glacial acetic acid:distilled water (v/v) (3:2:15) for one hour giving three changes of destain. 2.7. In-Gel Assay of Proteinase Inhibitor Gelatin zymography were performed according to Le and Katunuma [12] with resolving 10% acrylamide solu- tion containing 0.1% gelatin. Ion exchange chromatog- raphy fraction equivalent to 40 μg protein were mixed with equal volume of sample buffer containing 25 mM Tris/HCl (pH 6.8), 20% glycerol, 4% SDS and 0.02% bromophenol blue was loaded along with 80 µg of soy- bean trypsin inhibitor (Calbiochem®; 10 mg·mL−1 stock solution in Tris/HCl pH 8.2) which was used as a marker. Electrophoresis was performed at a constant voltage of 50 V at 4˚C in electrophoresis buffer (25 mM Tris/HCl, pH 8.8, 192 mM glycine and 0.1% SDS). The gel was washed for 30 min at room temperature with 0.1 M Tris- HCl at pH 7.2 containing 2.5% Triton X-100, followed by distilled water for 20 min. Subsequent incubation at 37˚C for 3 h with reaction buffer containing 10 mg·mL−1 trypsin solution (10 mg trypsin in 0.1 M Tris/HCl, pH 7.5) and 0.02% NaN3 followed by Coomassie Brilliant Blue R250 (CBB) staining for 1 h and destaining produced blue bands against a clear background. 2.8. Determination of Proteinase (Trypsin) Inhibitor Activity at Different Stages of Purification Steps The proteinase inhibitor activity on natural and syn- thetic chromo(non-chromo)genic substrates was deter- mined in a reaction mixture containing 50 mM Tris/HCl buffer (pH 7.5), 0.25 µg trypsin (EC 3.4.21.4) (10 mg· mL −1 of bovine trypsin in 1 mM HCl), different con- centration of all three ammonium sulphate cut off (100 μg), gel filtrate fraction (100 μg) and ion exchange frac- tions. The anion exchange fractions (43 μg, 86 μg, 430 μg protein) with 100 µl casein, 40 µl BApNA (10 mg· mL −1 in dimethyl sulfoxide); 0.23 mM BAEE in 67 mM potassium phosphate buffer (pH 7.5), 12.5% azoca- sein (w/v) in 50 mM Tris, 5 mM CaCl2 (pH 8.0). Con- trols with a trypsin enzyme (100˚C, 5 min) were run in parallel. The amount of p-nitroaniline (pNA) released was determined by measuring the change in absorbance at 410 nm using pNA calibration curve. Proteinase in- hibitor (TI) activity was also determined using a non- chromogenic substrate N-benzoyl-L-arg ethyl ester (BAEE) according to Schwert and Takenaka [13]. In azocasein, the reaction was terminated by adding 200 µl of ice-cold 5% TCA to 500 µl of reaction mixture. The reaction mixture was then placed at room tempera- ture for 10 min and centrifuged at 3,000 × g for 10 min, the supernatant (500 µl) was later aspirated and 1500 µl of 0.5M NaOH was added. The absorbance was recorded at A428 [14]. 2.9. Screening of Fungitoxic Activity The in vitro fungitoxic activity of crude extract of fusarium wilt resistant cultivar (viz. JG 2001-12) and fusarium wilt susceptible cultivar (viz. JG 16) was per- formed by mycelial disc method as described by Trema- coldi and Pascholati [15]. The mycelial disc was placed onto PDA medium, previously flooded with filtered (0.2 µm, Millipore) crude extracts in 25 µl, 500 µl, 1000 µl and 2000 µl volumes. The experiment was performed in triplicate for each concentrations and controls (with no crude extract added) under strict aseptic conditions. The fungitoxic activity of each concentration was expressed in terms of the mean of colony diameter in cm (mean value ± standard deviation) produced by respective ex- tract at the end of the incubation period (7 d). Gel filtra- tion fraction (Fraction A) was also tested against mycelia growth of Foc isolates at 10, 100 and 200 µg of protein (as described above). 2.10. Effect of Anion Exchange Fractions on the Hyphal and Conidial Growth of Fusarium oxysporum f.sp. ciceris The effect of purified CaTI protein (anion exchange fractions) on hyphal growth and conidial germination was assayed. Conidial suspension was prepared by flood- ing the cultures with sterile distilled water containing 0.05% (v/v) Tween-80 which was later filtered through four layers of sterilized cheesecloth to remove adhering mycelia. The spore concentration was adjusted to 105 conidia per mL with the aid of Neubauer hemocytometer. Conidia were allowed to germinate and grown in the presence of CaTI at 0, 5, 10, 15, 20 µg·mL−1 concentra- tions in 6-well plate at 25˚C in darkness with a positive control and negative control plates containing heat inac- tivated CaTI and phosphate buffer. For each treatment, hyphal length was measured from 40 randomly selected hyphae and the mean hyphal length was used for com- parison using a light microscope equipped with an ocular micrometer after 12 h and 24 h of incubation. As Foc is multicelled therefore; a conidium was considered germi- nated, if hyphae were visible for at least one of the cells. Each treatment contained three replicates and the ex- periment was repeated twice. 2.11. Statistical Analysis The results were subjected to analysis of variance (ANOVA) and the significance of differences among means was determined by using t-test using the MS Ex- cel program where p values <0.05 were considered sig- nificant. All experiments were performed in triplicate unless stated otherwise. Comparisons were made for all Copyright © 2013 SciRes. OPEN ACCE SS
 M. Nair, S. S. Sandhu / Agricultural Sciences 4 (2013) 585-594 588 parameters within the amount of inhibitor used as well as the hours of incubation during antimicrobial activity on Foc. 3. RESULTS AND DISCUSSION 3.1. Pathogenecity Assays Chickpea plants viz. JG 62, JG 2001-12 and JG 16 were inoculated with fungal spores of Foc individually where un-inoculated plants served as control. Upon in- fection, JG 62 and JG 16 showed wilting symptoms by 10 days after inoculation (dai), while no wilting was ob- served in JG 2001-12 cultivar even by the 8th week. Figure 1 shows the chickpea cultivars, where JG 16 ex- hibit wilting symptoms whereas JG 2001-12, display no wilting symptoms. 3.2. Preliminary Proteinase (Trypsin) Inhibitor Activity The inhibitory activity of the crude extract (viz., JG 2001-12 and JG 16) against bovine trypsin was deter- mined using natural and synthetic substrate viz. casein, BApNA respectively. Caseinolytic activity: Crude extract of JG 2001-12 and JG 16 was used to determine the proteinase inhibi- tory activity where the specific activity of trypsin was found to be 227 ± 0.5 nmol·tyr·min−1·mg−1 protein in absence of crude extract (trypsin inhibitor). The crude extract of Fusarium wilt resistant cultivar (viz. JG 2001- 12) displayed a 99% trypsin inhibition with 0.7 ± 0.4 nmol·tyr·min−1·mg−1 protein. The caseinolytic activity of Fusarium wilt susceptible cultivar (viz. JG 16) showed a 12% inhibition with 200 ± 0.5 nmol·tyr·min−1·mg−1 pro- tein TIA (Table 1). BApNA: Trypsin activity was rapidly inhibited (99.4% inhibition) by the crude extract of C. arietinum fusarium wilt resistant cultivar (viz. JG 2001-12), indicating the presence of trypsin inhibitor with 1.0 ± 0.3 µmol pNA min−1mg−1 protein TIA against bovine trypsin (Table 1). 3.3. Purification of Cicer Arietinum Trypsin Inhibitor (CaTI) The trypsin inhibitor from JG 2001-12 seed crude ex- tract was identified by trypsin inhibitory activity (TIA) assays using trypsin as the enzyme at preliminary identi- fication and purification steps. The purification results are presented in Figure 2 and Table 2. The proteinase inhibitor activity was revealed in 40% - 60% ammonium sulphate cut off (Figure 3). Chickpea proteinase (trypsin) inhibitor was isolated by dialysis, desalting in Sephadex G-100 and purified using DEAE-cellulose column eluted with 0.1 M NaCl. Upon size-exclusion chromatography Table 1. Trypsin Inhibitory activity (TIA) of crude extracts of fusarium wilt resistant (JG 2001-12) and susceptible (JG 16) cultivars. Casein BApNA TIA* TI# TIA* TI# Control 227.6 ± 0.50 172.01 ± 0.50 Crude extract of cultivar 1)JG 16 200b ± 0.5 12 145.2a ± 0.315.5 2)JG 2001-120.7a ± 0.4 99 1.0b ± 0.3 99.4 a,bMeans with different superscripts in a column differs significantly (p < 0.05). *TIA = nmol tyr min−1mg−1 protein; **TIA = µmol pNA min−1mg−1 protein; TI# = Trypsin Inhibition (%). Table 2. Purification performance of Trypsin Inhibitor from JG 2001-12 cultivar. Sample Total protein (mg) Specific Activity (µM pNA min−1mg −1) TIA* Yield (%) Purification Fold Crude Extract 258 2.32 44.5 100 1 Sephadex G-1009 72.54 86.7 25 57.1 DEAE-cellulose-52 column 0.1 140 94.4 10 65.4 *TIA = Trypsin Inhibitory activity. JG 2001-12 JG 16 Figure 1. Fusarium wilt susceptible cultivar (JG 16) and resistant (JG 2001-12) showing wilting and chlorosis. on Sephadex G-100 column, two protein peaks were ob- tained where proteinase inhibitor was detected in the first peak (Figure 2(a)) which consisted of three fractions (fraction 9, 10, 11 called as Fraction A). Fraction A showed maximum trypsin inhibitory activity against trypsin enzyme (Figures 4(a), (b)). Further, the trypsin inhibitor was retained by anion exchange column- DEAE-cellulose column and eluted in fractions of 0.1 M NaCl (Figure 2(b)). Taken together, approximately 10% recovery and 65.4 fold increase in specific activity re- sulted (Table 2). Fractions of size exclusion chromatog- raphy, anion exchange chromatography were employed on SDS-PAGE and reverse zymography (using gelatin as ubstrate) to verify the purity of trypsin inhibitor and s Copyright © 2013 SciRes. OPEN ACCE SS
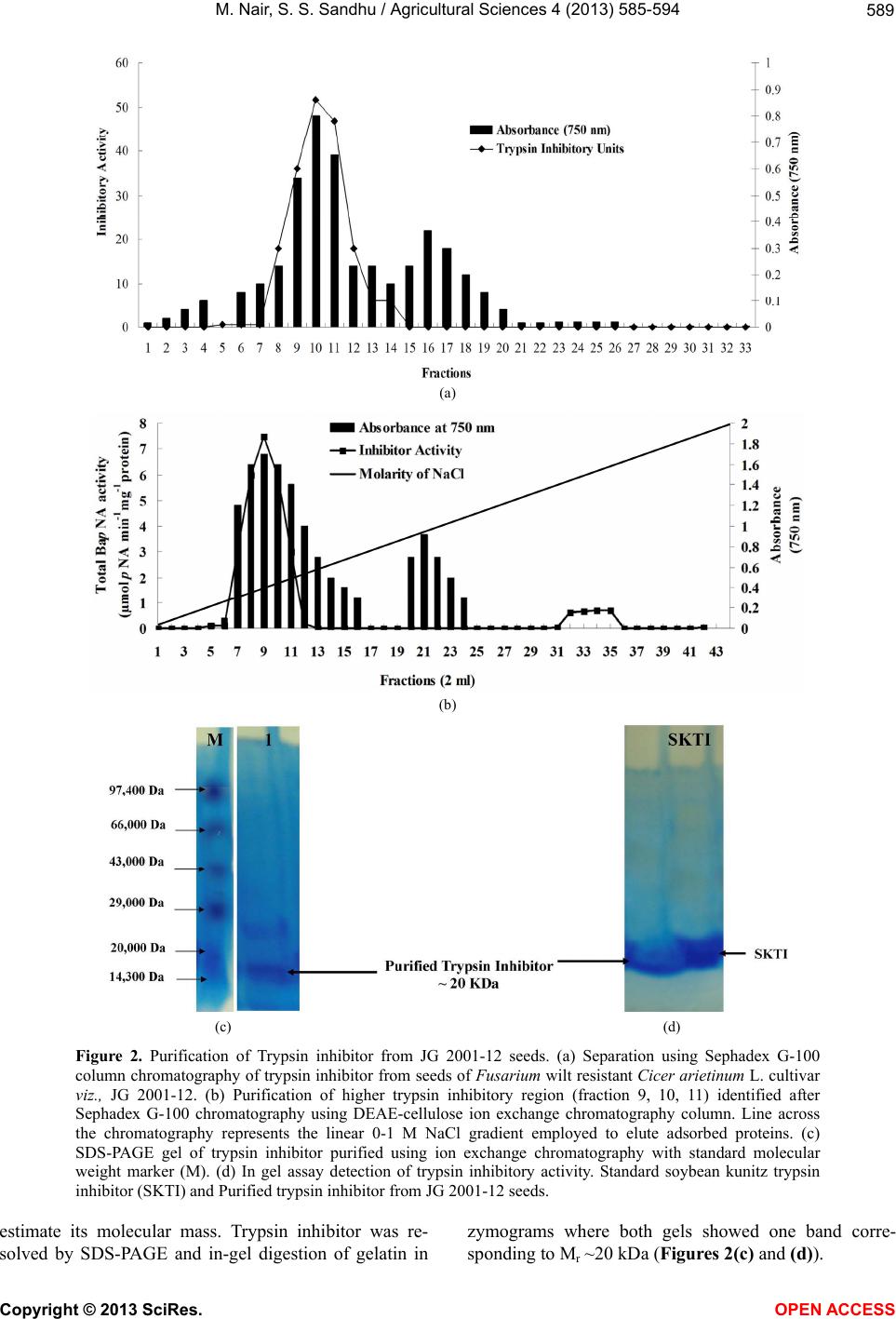 M. Nair, S. S. Sandhu / Agricultural Sciences 4 (2013) 585-594 Copyright © 2013 SciRes. 589 (a) (b) (c) (d) Figure 2. Purification of Trypsin inhibitor from JG 2001-12 seeds. (a) Separation using Sephadex G-100 column chromatography of trypsin inhibitor from seeds of Fusarium wilt resistant Cicer arietinum L. cultivar viz., JG 2001-12. (b) Purification of higher trypsin inhibitory region (fraction 9, 10, 11) identified after Sephadex G-100 chromatography using DEAE-cellulose ion exchange chromatography column. Line across the chromatography represents the linear 0-1 M NaCl gradient employed to elute adsorbed proteins. (c) SDS-PAGE gel of trypsin inhibitor purified using ion exchange chromatography with standard molecular weight marker (M). (d) In gel assay detection of trypsin inhibitory activity. Standard soybean kunitz trypsin inhibitor (SKTI) and Purified trypsin inhibitor from JG 2001-12 seeds. estimate its molecular mass. Trypsin inhibitor was re- solved by SDS-PAGE and in-gel digestion of gelatin in zymograms where both gels showed one band corre- sponding to Mr ~20 kDa (Figures 2(c) and (d)). OPEN A CCESS
 M. Nair, S. S. Sandhu / Agricultural Sciences 4 (2013) 585-594 590 Figure 3. Trypsin Inhibitory Activity (TIA) in different ammo- nium sulphate cut offs of protein homogenate of JG 2001-12 cultivar in comparison to control (with no crude extract) using BApNA substrate. * * ** * *** * 0 50 100 150 200 TIA ControlFraction 9Fraction 10Fraction 11 Treatments Trypsin Inhibition (%)TIA * ** ** 0 20 40 60 80 100 120 140 160 180 200 ControlJG 2001-12Gel Filtration Fraction (Fraction A) Anion Exchange Fraction Treatments TIA (µmol pNA min -1 mg -1 protein) Figure 4. Trypsin Inhibitory Activity (TIA) Assay. 3.4. Trypsin Inhibitor Activity Assay Trypsin inhibitor activity assay (TIA) of purified tryp- sin inhibitor at different purification steps using synthetic substrates (BApNA, azocasein, BAEE) were carried where the reliability of the reaction conditions was de- termined in triplicate sets. The TIA using BApNA sub- strate, at different concentration of CaTI protein [(5 μL) (43 μg), 10 (86 μg), 15 μL (430 μg)] showed a progres- sive reduction in trypsin activity, with increase in con- centration of CaTI protein. The inhibitory activity was increased from 86.2%, 97.2% to 98.61% with protein concentration (Figure 5). Similarly, in azocasein and BAEE substrate, absorbance at 428 nm and 253 nm was found to be high in control (with no CaTI) with respect to the proteinase activity in the presence of trypsin in- hibitor in fusarium wilt resistant cultivar crude extract viz. JG 2001-12. The trypsin activity was calculated in units where, one unit of trypsin was defined as the amount that increased A428 by 0.01/min under the assay conditions. Figure 5. Effect of different concentration of trypsin inhibitor on activity of trypsin using synthetic substrates (BApNA, Azocasein, BAEE) as described in Materials and Methods. Error bars represent standard deviations. Inset, showing the protein concentration at different volume of trypsin inhibitor. Trypsin inhibitory units were calculated from the number of trypsin units under similar conditions in the presence of trypsin inhibitor. 50 µl of inhibitor (320 µg equivalent protein) was used as the optimum concentration so to inhibit trypsin activity. It was found that after 30 min of incubation, there was no increase in the absorbance at 428 nm and 253 nm, suggesting an inhibition in trypsin activity (Figure 5). Our results demonstrated no varia- tion in proteolytic activity of trypsin on natural and syn- thetic substrates in presence and absence of trypsin in- hibitor. The results indicated that the crude extract of fusarium wilt resistant cultivar (viz. JG 2001-12) inhibit proteolysis of natural and synthetic substrates in the presence of bovine trypsin. The method we adopted here Copyright © 2013 SciRes. OPEN ACCE SS
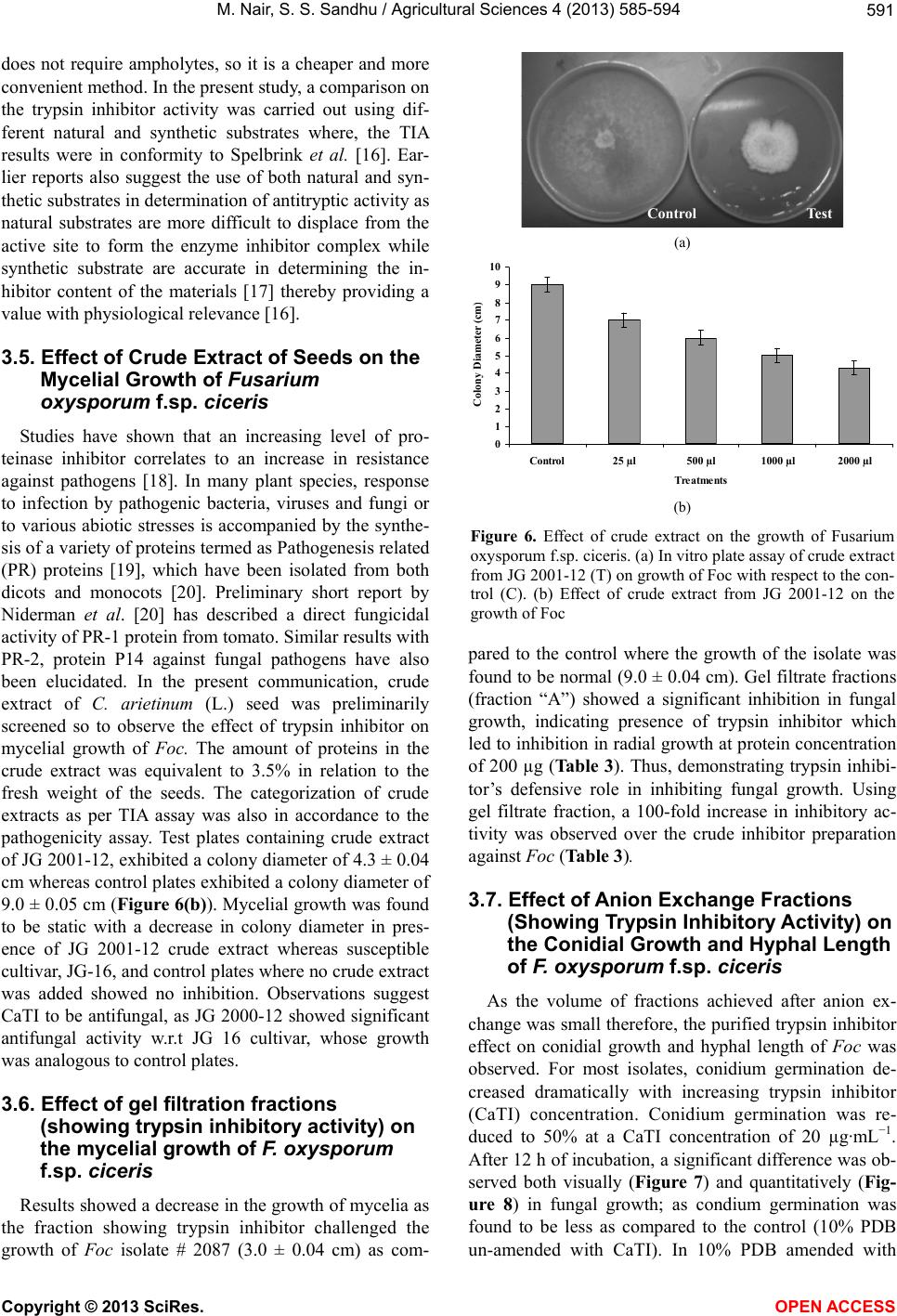 M. Nair, S. S. Sandhu / Agricultural Sciences 4 (2013) 585-594 591 does not require ampholytes, so it is a cheaper and more convenient method. In the present study, a comparison on the trypsin inhibitor activity was carried out using dif- ferent natural and synthetic substrates where, the TIA results were in conformity to Spelbrink et al. [16]. Ear- lier reports also suggest the use of both natural and syn- thetic substrates in determination of antitryptic activity as natural substrates are more difficult to displace from the active site to form the enzyme inhibitor complex while synthetic substrate are accurate in determining the in- hibitor content of the materials [17] thereby providing a value with physiological relevance [16]. 3.5. Effect of Crude Extract of Seeds on the Mycelial Growth of Fusarium oxysporum f.sp. ciceris Studies have shown that an increasing level of pro- teinase inhibitor correlates to an increase in resistance against pathogens [18]. In many plant species, response to infection by pathogenic bacteria, viruses and fungi or to various abiotic stresses is accompanied by the synthe- sis of a variety of proteins termed as Pathogenesis related (PR) proteins [19], which have been isolated from both dicots and monocots [20]. Preliminary short report by Niderman et al. [20] has described a direct fungicidal activity of PR-1 protein from tomato. Similar results with PR-2, protein P14 against fungal pathogens have also been elucidated. In the present communication, crude extract of C. arietinum (L.) seed was preliminarily screened so to observe the effect of trypsin inhibitor on mycelial growth of Foc . The amount of proteins in the crude extract was equivalent to 3.5% in relation to the fresh weight of the seeds. The categorization of crude extracts as per TIA assay was also in accordance to the pathogenicity assay. Test plates containing crude extract of JG 2001-12, exhibited a colony diameter of 4.3 ± 0.04 cm whereas control plates exhibited a colony diameter of 9.0 ± 0.05 cm (Figure 6(b)). Mycelial growth was found to be static with a decrease in colony diameter in pres- ence of JG 2001-12 crude extract whereas susceptible cultivar, JG-16, and control plates where no crude extract was added showed no inhibition. Observations suggest CaTI to be antifungal, as JG 2000-12 showed significant antifungal activity w.r.t JG 16 cultivar, whose growth was analogous to control plates. 3.6. Effect of gel filtration fractions (showing trypsin inhibitory activity) on the mycelial growth of F. oxysporum f.sp. ciceris Results showed a decrease in the growth of mycelia as the fraction showing trypsin inhibitor challenged the growth of Foc isolate # 2087 (3.0 ± 0.04 cm) as com- Control Test (a) 0 1 2 3 4 5 6 7 8 9 10 Control25 µl500 µl1000 µl2000 µl Treatments Colony Diameter (cm (b) Figure 6. Effect of crude extract on the growth of Fusarium oxysporum f.sp. ciceris. (a) In vitro plate assay of crude extract from JG 2001-12 (T) on growth of Foc with respect to the con- trol (C). (b) Effect of crude extract from JG 2001-12 on the growth of Foc pared to the control where the growth of the isolate was found to be normal (9.0 ± 0.04 cm). Gel filtrate fractions (fraction “A”) showed a significant inhibition in fungal growth, indicating presence of trypsin inhibitor which led to inhibition in radial growth at protein concentration of 200 µg (Table 3). Thus, demonstrating trypsin inhibi- tor’s defensive role in inhibiting fungal growth. Using gel filtrate fraction, a 100-fold increase in inhibitory ac- tivity was observed over the crude inhibitor preparation against Foc (Table 3). 3.7. Effect of Anion Exchange Fractions (Showing Trypsin Inhibitory Activity) on the Conidial Growth and Hyphal Length of F. oxysporum f.sp. ciceris As the volume of fractions achieved after anion ex- change was small therefore, the purified trypsin inhibitor effect on conidial growth and hyphal length of Foc was observed. For most isolates, conidium germination de- creased dramatically with increasing trypsin inhibitor (CaTI) concentration. Conidium germination was re- duced to 50% at a CaTI concentration of 20 µg·mL−1. After 12 h of incubation, a significant difference was ob- served both visually (Figure 7) and quantitatively (Fig- ure 8) in fungal growth; as condium germination was found to be less as compared to the control (10% PDB un-amended with CaTI). In 10% PDB amended with Copyright © 2013 SciRes. OPEN ACCE SS
 M. Nair, S. S. Sandhu / Agricultural Sciences 4 (2013) 585-594 592 Table 3. Effect of gel filtrate fraction “A” on growth of Fusarium oxysporum f. sp. ciceris at different concentration of protein (µg). Treatment Colony diameter (cm) 1) Control 9.0 ± 0.04 2) 10 µg 5.0 ± 0.2 3) 100 µg 4.0 ± 0.25 4) 200 µg 3.0 ± 0.04 a) (b) (c) (d) Figure 7. Inhibition of Fusarium oxysporum f.sp. ciceris co- nidia germination. Ion exchange chromatography fractions are mixed with PDB and incubated at 37˚C. Bar = 10 μm. (a) 10% potato dextrose broth (PDB) alone at 0 h; (b) PDB amended with trypsin inhibitor (20 μg·mL–1) at 12 h; (c) PDB amended with trypsin inhibitor (20 μg·mL–1) after 24 h; (d) Control, with no trypsin inhibitor. CaTI (15 µg·mL−1), hyphal growth was observed to be 25 µm as compared with 200 µm in 10% PDB (control). At the end of 24 h of incubation, PDB amended with CaTI (15 µg·mL−1) showed an increase in hyphal growth from 25 µm to 52 µm, whereas there was a drastic in- crease in germination and hyphal growth of the mycelia (340 µm) in 10% PDB (control). At the end of 24 h of incubation, the hyphal length in the PDB amended with CaTI at 20 μg·mL−1 remained *** ** ** *** 0 50 100 150 200 250 300 350 400 Control5 µg/mL10 µg/mL15 µg/mL 20 µg/mL Treatments Hyphal growth µ 12 h24 h ( Figure 8. Fungal hyphal growth (μm) with different treat- ments of trypsin inhibitor fractions of anion exchange column chromatography as described in Materials and Method. nearly the same as that at 12 h with little variation (i.e. at 12 h the hyphal growth was found to be 40 μm and after 24 h the hyphal growth was 42 μm). The results obtained using conidial germination and hyphal growth was in correlation to each other as the results showed inhibition in the presence of trypsin inhibitor were consistent (Figures 7 and 8). These studies were similar to Chen et al. [21] reports, which showed that the resistance of cer- tain corn genotypes to fungal infection is related to the action of trypsin inhibitor, which is due to the lowering in the production and activity of fungal alpha-amylase, which in turn reduce the availability of simple sugars for fungal growth. Similar studies on the inhibition of spore germination and mycelium growth of Alternaria alter- nata by buckwheat trypsin/chymotrypsin was observed [22]. Likewise, cysteine proteinase inhibitors from pearl millet also inhibited the growth of many pathogenic fungi including Trichoderma reesei [23]. In addition, the antifungal effect of trypsin inhibitor was observed in wheat kernel [24], corn [21], barley [25] and cabbage [26]. Our results were in corroborance with Chen et al. [21], Huang et al. [27], Revina et al. [28] who described the use of resistant TI in inhibiting germination and hyphal growth of plant pathogenic fungi. Collating the present and previously reported data, it appears that Cicer arietinum trypsin inhibitor (CaTI) act as an antifungal agent since in vitro activity of CaTI showed a 45% - 82% suppression of conidial germination and hyphal growth, thereby showing its effectiveness against Foc. As Foc survives in soil, therefore use of TI containing Cicer arietinum L. (CaTI) would be an easy approach in order to reduce the use of chemicals/fungicides to com- bat Fusarium wilt. In the present study, a sustainable ap- proach in controlling Fusarium wilt was utilized via the use of trypsin inhibitor as implementation of cultural, physical, biological and chemical measures minimize economic risks to consumers and the environment. To sum up, CaTI provide a defensive property to host plant Copyright © 2013 SciRes. OPEN ACCE SS
 M. Nair, S. S. Sandhu / Agricultural Sciences 4 (2013) 585-594 593 as a cultivar containing trypsin inhibitor showed resis- tance against Fusarium wilt. In this context, future re- search to identify eco-friendly tools for the biological control of pathogens and further studies are warranted to confirm its biotechnological potential against other pathogens inflicting Cicer arietinum (L.). 3.8. Abbreviations and Acronyms BAEE, N-benzoyl-L-Arg ethyl ester; BApNA, N-α- Benzoyl-D, L-arginine p-nitroanilide hydrochloride; CLA, carnation leaf agar; CaTI, Cicer arietinum Trypsin In- hibitor; Foc, Fusarium oxysporum f.sp. ciceris; PDA, Potato Dextrose Agar; PDB, Potato Dextrose Broth; p-NA, para-nitroaniline; TI, Trypsin Inhibitor; TIA, Trypsin Inhibitor Activity; TCA, Trichloroacetic acid. 4. ACKNOWLEDGEMENTS The authors would like to thank the Head of the Department of Bio- logical Sciences, Rani Durgavati University, Jabalpur (India) for labo- ratory facilities and University Grant Commission Project (Govt. of India), New Delhi for financial assistance vide project no. UGC (32-370/2006) (SR) dated. 24/04/07. Authors also acknowledge JNKVV, Jabalpur, India for providing the seed material. REFERENCES [1] Varshney, R.K., Song, C., Saxena, R.K., Azam, S., Yu, S., Sharpe, A.G. Cannon, S., Baek, J., Rosen, B.D., Tar'an, B., Millan, T., Zhang, X., Ramsay, L.D., Iwata, A., Wang, Y., Nelson, W., Farmer, A.D., Gaur, P.M., Soderlund, C., Penmetsa, R.V., Xu, C., Bharti, A.K., He, W., Winter, P., Zhao, S., Hane, J.K.,Carrasquilla-Garcia, N., Condie, J.A., Upadhyaya, H.D., Luo, M.C., Thudi, M., Gowda, C.L., Singh, N.P., Lichtenzveig, J., Gali, K.K., Rubio, J., Na- darajan, N., Dolezel, J., Bansal, K.C., Xu, X., Edwards, D., Zhang, G., Kahl, G., Gil, J., Singh, K.B., Datta, S.K., Jackson, S.A., Wang, J. and Cook, D.R. (2013) Draft ge- nome sequence of chickpea (Cicer arietinum) provides a resource for trait improvement. Nature Biotechnology, 31, 240-246. http://dx.doi.org/10.1038/nbt.2491 [2] Landa, B.B., Navas-Cortés, J.A., Jiménez-Gasco, M.M., Katan, J., Retig, B. and Jiménez-Díaz, R.M. (2006) Tem- perature response of chickpea cultivars to races of Fusa- rium oxysporum f. sp. ciceris, causal agent of Fusarium wilt. Plant Disease, 90, 365-374. http://dx.doi.org/10.1094/PD-90-0365 [3] Haware, M.P., Nene, Y.L. and Natarajan, M. (1996) The survival of Fusarium oxysporum f. sp. ciceri in the soil in the absence of chickpea. Phytopathologia Mediterranea, 35, 9-12. [4] Sharma, K.D. and Muehlbauer, F.J. (2007) Fusarium wilt of chickpea: Physiological specialization, genetics of re- sistance and resistance gene tagging. Euphytica, 157, 1-14. http://dx.doi.org/10.1007/s10681-007-9401-y [5] Habib, H. and Fazili, K.M. (2007) Plant protease inhibi- tors: A defense strategy in plants. Biotechnology and Mo- lecular Biology Review, 2, 68-85. [6] Zavala, J.A., Patankar, A.G., Gase, K., Hui, D. and Bald- win, I.T. (2004) Manipulation of endogenous trypsin pro- teinase inhibitor production in Nicotiana attenuata dem- onstrates their function as antiherbivore defenses. Plant Physiology, 134, 1181-1190. http://dx.doi.org/10.1104/pp.103.035634 [7] Srinivasan, A., Chougule, N.P., Giri, A.P., Gatehouse, J.A. and Gupta, V.S. (2005) Podborer (Helicoverpa armigera Hübn.) does not show specific adaptations in gut pro- teinases to dietary Cicer arietinum Kunitz proteinase in- hibitor. Journal of Insect Physiology, 51, 1268-1276. http://dx.doi.org/10.1016/j.jinsphys.2005.07.005 [8] Nair, M., Singh, S.S. and Babbar, A. (2013) Purification of trypsin inhibitor (TI) from seeds of Cicer arietinum (L.) and its insecticidal potentiality against Helicoverpa ar- migera (Hübner). Theoretical and Experimental Plant Physiology, Vol. 25, No. 2, pp. 141-152. [9] Brock, F.M., Forsberg, C.W. and Buchanan-Smith, J.G. (1982) Proteolytic activity of rumen microorganisms and effects of proteinase inhibitors. Applied and Environ- mental Microbiology, 44, 561-569. [10] Lowry, O., Rosbrough, N., Farr, A. and Randall, R. (1951) Protein measurement with the folin phenol reagent. Jour- nal of Biological Chemistry, 193, 265-275. [11] Laemmli, U.K. (1970) Cleavage of structural proteins during the assembly of head of bacteriophage T4. Nature, 227, 680-685. http://dx.doi.org/10.1038/227680a0 [12] Le, Q.T. and Katunuma, N. (2004) Determination of pro- tease inhibitors by a reverse zymography method per- formed in a Tris-Tricine buffer system. Analytical Bio- chemistry, 324, 237-240. http://dx.doi.org/10.1016/j.ab.2003.09.033 [13] Schwert, W.H. and Takenaka, Y. (1955) A spectropho- tometric determination of trypsin and chymotrypsin. Bio- chimica Biophysica Acta, 16, 570-575. http://dx.doi.org/10.1016/0006-3002(55)90280-8 [14] Gatehouse, A.M.R., Gatehouse, J.A. and Boulter, D. (1980) Isolation and characterization of trypsin inhibitors from cowpea (Vigna unguiculata). Phytochemistry, 19, 751-756. http://dx.doi.org/10.1016/0031-9422(80)85104-1 [15] Tremacoldi, C.R. and Pascholati, S.F. (2002) Detection of trypsin inhibitor in seeds of Eucalyptus urophylla and its influence on the in vitro growth of the fungi Pisolithus tinctorius and Rhizoctonia solani. Brazilian Journal of Microbiology, 33, 281-286. http://dx.doi.org/10.1590/S1517-83822002000400001 [16] Spelbrink, R.E.J., Gerrits, P.J., Mooij, C. and Giuseppin, M.L.F. (2011) Quantitative Determination of Trypsin In- hibitory Activity in Complex Matrices. Open Food Sci- ence Journal, 5, 42-46. http://dx.doi.org/10.2174/1874256401105010042 [17] Kakade, M.L., Simons, N. and Liener, I.E. (1969) An evaluation of natural vs. synthetic substrates for measure- ing the antitryptic activity of soybean samples. Cereal Chemistry, 46, 518-526. [18] Woloshuk, C.P., Meulenhoff, J.S., Sela-Buurlage, M., van den Elzen, P.J.M. and Cornelissen, B.J.C. (1991) Patho- Copyright © 2013 SciRes. OPEN ACCE SS
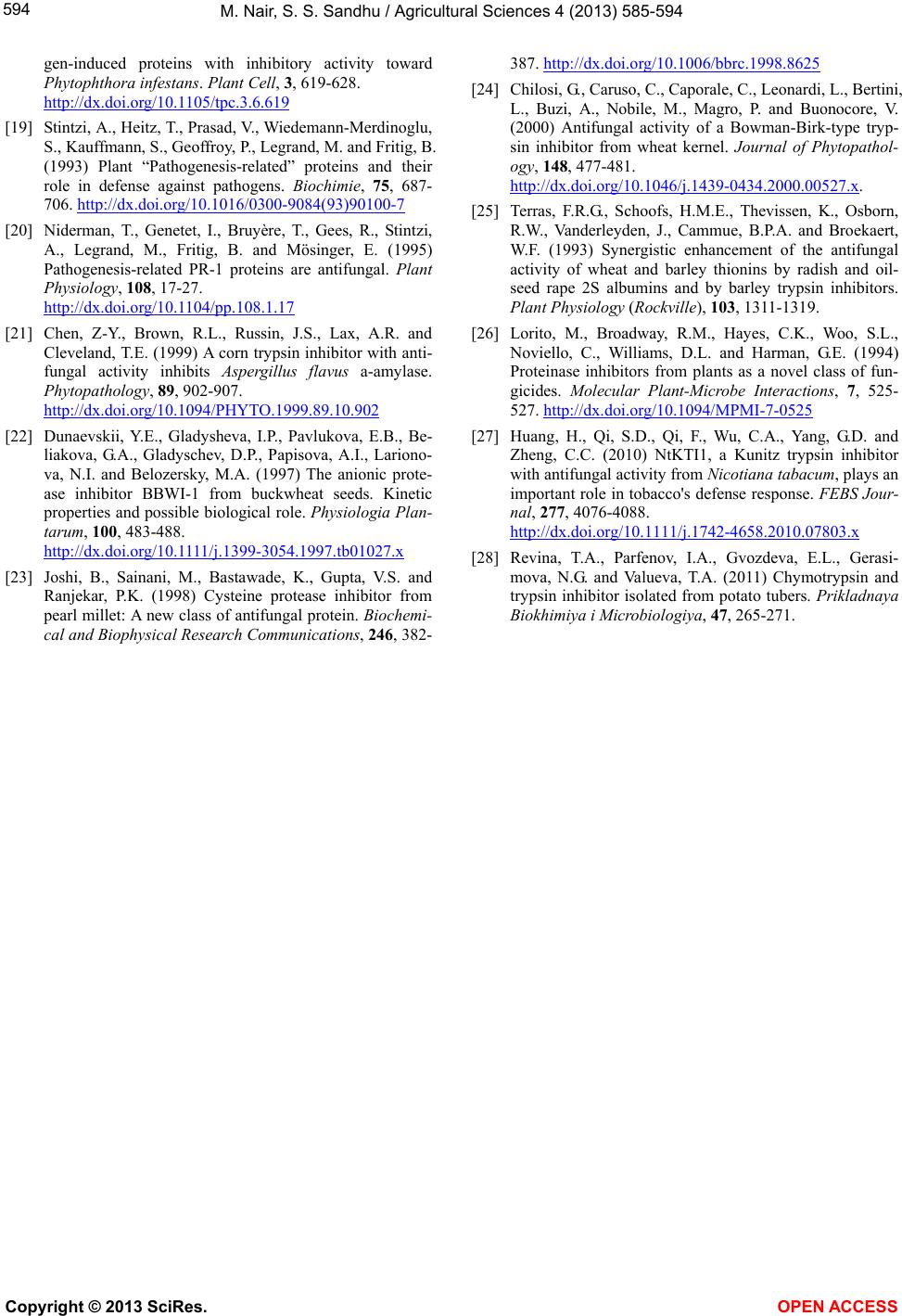 M. Nair, S. S. Sandhu / Agricultural Sciences 4 (2013) 585-594 Copyright © 2013 SciRes. OPEN ACCE SS 594 gen-induced proteins with inhibitory activity toward Phytophthora infestans. Plant Cell, 3, 619-628. http://dx.doi.org/10.1105/tpc.3.6.619 [19] Stintzi, A., Heitz, T., Prasad, V., Wiedemann-Merdinoglu, S., Kauffmann, S., Geoffroy, P., Legrand, M. and Fritig, B. (1993) Plant “Pathogenesis-related” proteins and their role in defense against pathogens. Biochimie, 75, 687- 706. http://dx.doi.org/10.1016/0300-9084(93)90100-7 [20] Niderman, T., Genetet, I., Bruyère, T., Gees, R., Stintzi, A., Legrand, M., Fritig, B. and Mösinger, E. (1995) Pathogenesis-related PR-1 proteins are antifungal. Plant Physiology, 108, 17-27. http://dx.doi.org/10.1104/pp.108.1.17 [21] Chen, Z-Y., Brown, R.L., Russin, J.S., Lax, A.R. and Cleveland, T.E. (1999) A corn trypsin inhibitor with anti- fungal activity inhibits Aspergillus flavus a-amylase. Phytopathology, 89, 902-907. http://dx.doi.org/10.1094/PHYTO.1999.89.10.902 [22] Dunaevskii, Y.E., Gladysheva, I.P., Pavlukova, E.B., Be- liakova, G.A., Gladyschev, D.P., Papisova, A.I., Lariono- va, N.I. and Belozersky, M.A. (1997) The anionic prote- ase inhibitor BBWI-1 from buckwheat seeds. Kinetic properties and possible biological role. Physiologia Plan- tarum, 100, 483-488. http://dx.doi.org/10.1111/j.1399-3054.1997.tb01027.x [23] Joshi, B., Sainani, M., Bastawade, K., Gupta, V.S. and Ranjekar, P.K. (1998) Cysteine protease inhibitor from pearl millet: A new class of antifungal protein. Biochemi- cal and Biophysical Research Communications, 246, 382- 387. http://dx.doi.org/10.1006/bbrc.1998.8625 [24] Chilosi, G., Caruso, C., Caporale, C., Leonardi, L., Bertini, L., Buzi, A., Nobile, M., Magro, P. and Buonocore, V. (2000) Antifungal activity of a Bowman-Birk-type tryp- sin inhibitor from wheat kernel. Journal of Phytopathol- ogy, 148, 477-481. http://dx.doi.org/10.1046/j.1439-0434.2000.00527.x. [25] Terras, F.R.G., Schoofs, H.M.E., Thevissen, K., Osborn, R.W., Vanderleyden, J., Cammue, B.P.A. and Broekaert, W.F. (1993) Synergistic enhancement of the antifungal activity of wheat and barley thionins by radish and oil- seed rape 2S albumins and by barley trypsin inhibitors. Plant Physiology (Rockville), 103, 1311-1319. [26] Lorito, M., Broadway, R.M., Hayes, C.K., Woo, S.L., Noviello, C., Williams, D.L. and Harman, G.E. (1994) Proteinase inhibitors from plants as a novel class of fun- gicides. Molecular Plant-Microbe Interactions, 7, 525- 527. http://dx.doi.org/10.1094/MPMI-7-0525 [27] Huang, H., Qi, S.D., Qi, F., Wu, C.A., Yang, G.D. and Zheng, C.C. (2010) NtKTI1, a Kunitz trypsin inhibitor with antifungal activity from Nicotiana tabacum, plays an important role in tobacco's defense response. FEBS Jour- nal, 277, 4076-4088. http://dx.doi.org/10.1111/j.1742-4658.2010.07803.x [28] Revina, T.A., Parfenov, I.A., Gvozdeva, E.L., Gerasi- mova, N.G. and Valueva, T.A. (2011) Chymotrypsin and trypsin inhibitor isolated from potato tubers. Prikladnaya Biokhimiya i Microbiologiya, 47, 265-271.
|