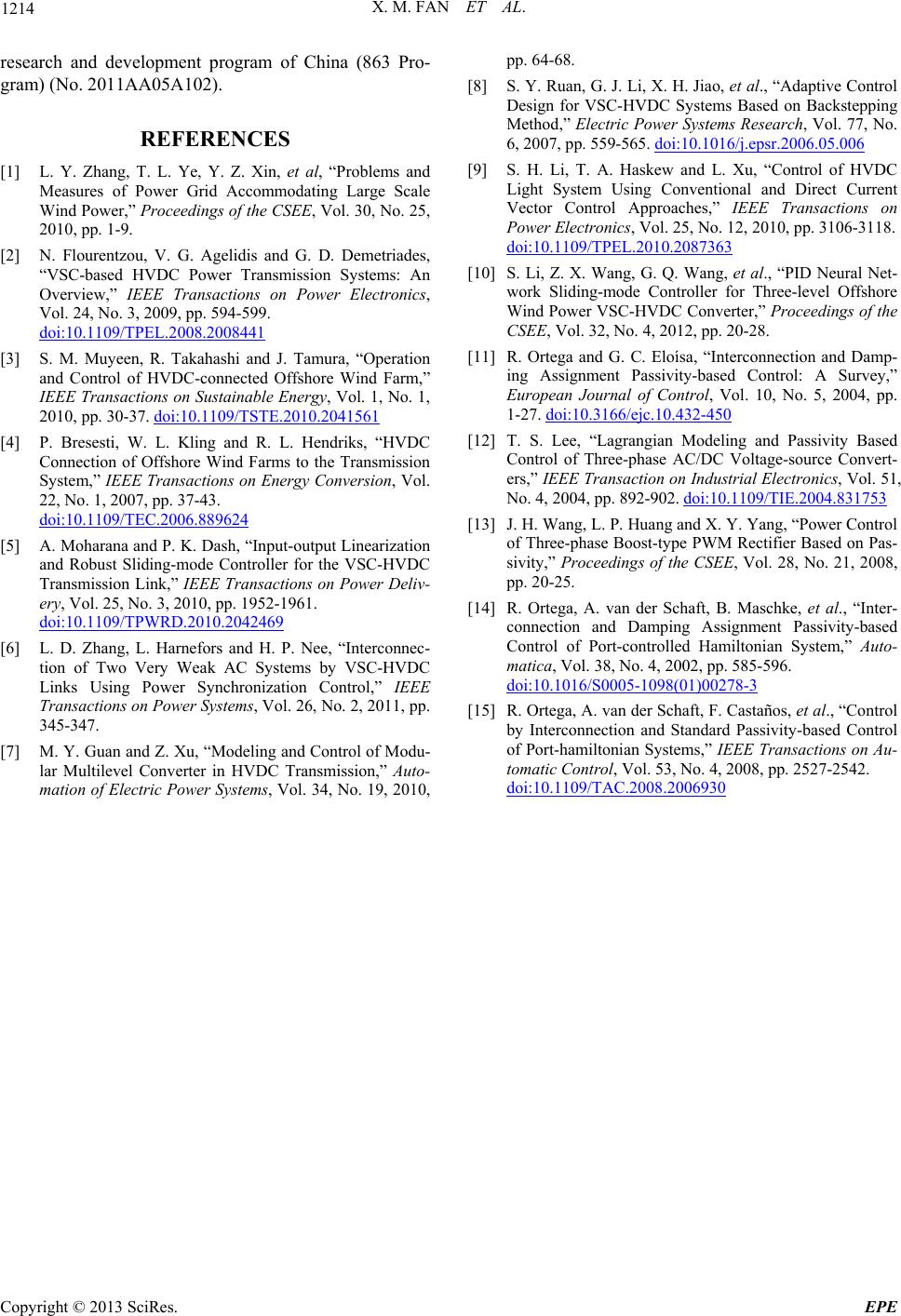
X. M. FAN ET AL.
Copyright © 2013 SciRes. EPE
1214
REFERENCES
[1] L. Y. Zhang, al, “Problem
, V. G. Agelidis and G. D. Demetriades,
research and development program of China (863 Pro-
gram) (No. 2011AA05A102).
T. L. Ye, Y. Z. Xin, et s and
Measures of Power Grid Accommodating Large Scale
Wind Power,” Proceedings of the CSEE, Vol. 30, No. 25,
2010, pp. 1-9.
[2] N. Flourentzou
“VSC-based HVDC Power Transmission Systems: An
Overview,” IEEE Transactions on Power Electronics,
Vol. 24, No. 3, 2009, pp. 594-599.
doi:10.1109/TPEL.2008.2008441
[3] S. M. Muyeen, R. Takahashi and J. Tamura, “Operation
and Control of HVDC-connected Offshore Wind Farm,”
IEEE Transactions on Sustainable Energy, Vol. 1, No. 1,
2010, pp. 30-37. doi:10.1109/TSTE.2010.2041561
[4] P. Bresesti, W. L. Kling and R. L. Hendriks, “HVDC
Connection of Offshore Wind Farms to the Transmission
System,” IEEE Transactions on Energy Conversion, Vol.
22, No. 1, 2007, pp. 37-43.
doi:10.1109/TEC.2006.889624
[5] A. Moharana and P. K. Dash, “Input-output Linearization
and Robust Sliding-mode Controller for the VSC-HVDC
Transmission Link,” IEEE Transactions on Power Deliv-
ery, Vol. 25, No. 3, 2010, pp. 1952-1961.
doi:10.1109/TPWRD.2010.2042469
[6] L. D. Zhang, L. Harnefors and H. P. Nee, “Interconnec-
and Z. Xu, “Modeling and Control of Modu-
tion of Two Very Weak AC Systems by VSC-HVDC
Links Using Power Synchronization Control,” IEEE
Transactions on Power Systems, Vol. 26, No. 2, 2011, pp.
345-347.
[7] M. Y. Guan
lar Multilevel Converter in HVDC Transmission,” Auto-
mation of Electric Power Systems, Vol. 34, No. 19, 2010,
pp. 64-68.
[8] S. Y. Ruan, G. J. Li, X. H. Jiao, et al., “Adaptive Control
Design for VSC-HVDC Systems Based on Backstepping
Method,” Electric Power Systems Research, Vol. 77, No.
6, 2007, pp. 559-565. doi:10.1016/j.epsr.2006.05.006
[9] S. H. Li, T. A. Haskew and L. Xu, “Control of HVDC
Light System Using Conventional and Direct Current
Vector Control Approaches,” IEEE Transactions on
Power Electronics, Vol. 25, No. 12, 2010, pp. 3106-3118.
doi:10.1109/TPEL.2010.2087363
[10] S. Li, Z. X. Wang, G. Q. Wang, et al., “PID Neural Net-
work Sliding-mode Controller for Three-level Offshore
Wind Power VSC-HVDC Converter,” Proceedings of the
CSEE, Vol. 32, No. 4, 2012, pp. 20-28.
[11] R. Ortega and G. C. Eloísa, “Interconnection and Damp-
ing Assignment Passivity-based Control: A Survey,”
European Journal of Control, Vol. 10, No. 5, 2004, pp.
1-27. doi:10.3166/ejc.10.432-450
[12] T. S. Lee, “Lagrangian Modeling and Passivity Based
Control of Three-phase AC/DC Voltage-source Convert-
ers,” IEEE Transaction on Industrial Electronics, Vol. 51,
No. 4, 2004, pp. 892-902. doi:10.1109/TIE.2004.831753
[13] J. H. Wang, L. P. Huang and X. Y. Yang, “Power Control
of Three-phase Boost-type PWM Rectifier Based on Pas-
sivity,” Proceedings of the CSEE, Vol. 28, No. 21, 2008,
pp. 20-25.
[14] R. Ortega, A. van der Schaft, B. Maschke, et al., “Inter-
connection and Damping Assignment Passivity-based
Control of Port-controlled Hamiltonian System,” Auto-
matica, Vol. 38, No. 4, 2002, pp. 585-596.
doi:10.1016/S0005-1098(01)00278-3
[15] R. Ortega, A. van der Schaft, F. Castaños, et al., “Control
by Interconnection and Standard Passivity-based Control
of Port-hamiltonian Systems,” IEEE Transactions on Au-
tomatic Control, Vol. 53, No. 4, 2008, pp. 2527-2542.
doi:10.1109/TAC.2008.2006930