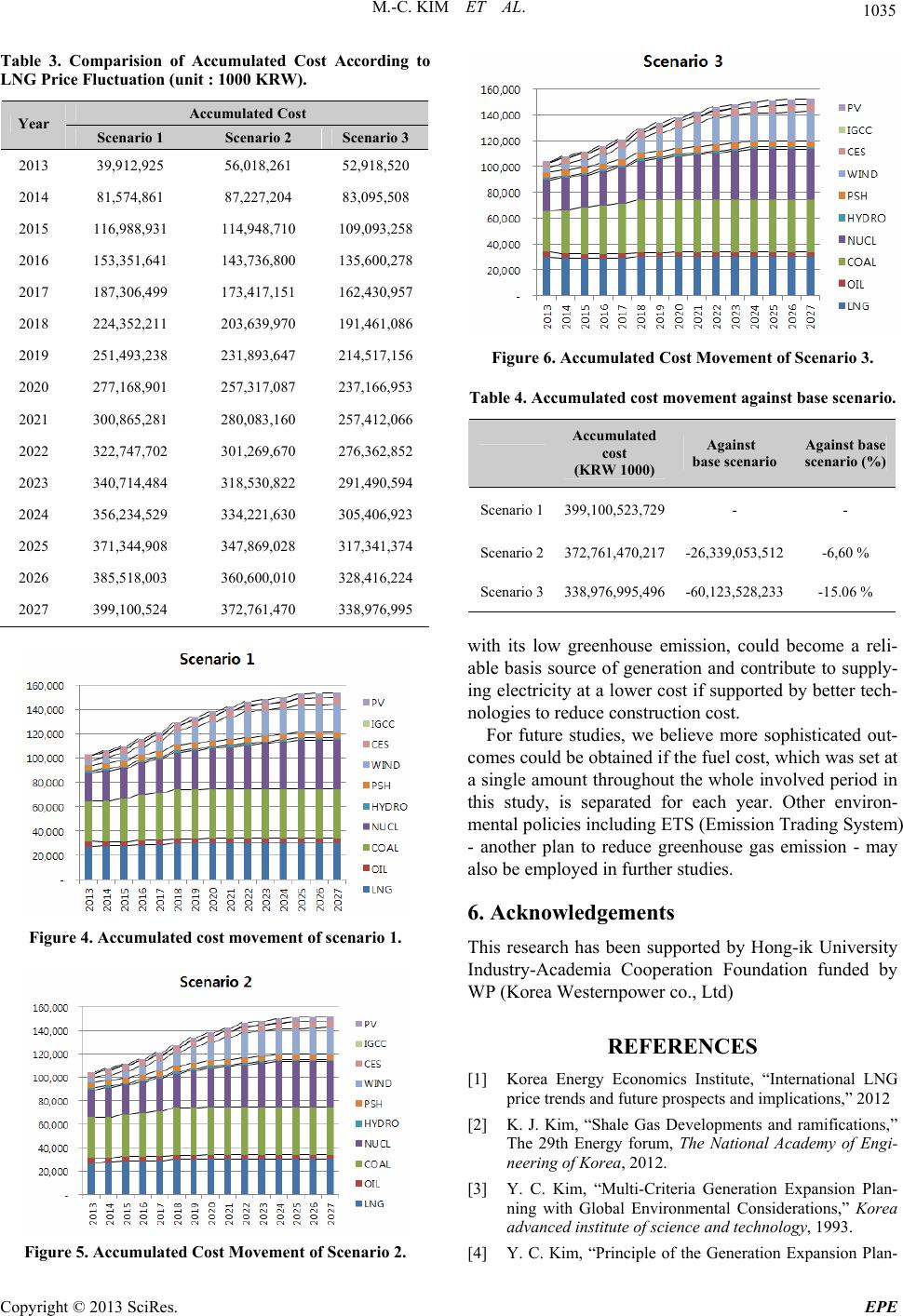
M.-C. KIM ET AL. 1035
Table. Compag to 3rision of Accumulated Cost Accordin
LNG Price Fluctuation (unit : 1000 KRW).
Accumulated Cost
Year Scenario 1 Scenario 2 Scenario 3
2013 39,912,925 56,018,261 52,918,520
2014 81,574,861 87,227,204 83,095,508
2015 116,988,931 114,948,710 109,093,258
2016 153,351,641 143,736,800 135,600,278
2017 187,306,499 173,417,151 162,430,957
2018 224,352,211 203,639,970 191,461,086
2019 251,493,238 231,893,647 214,517,156
2020 277,168,901 257,317,087 237,166,953
2021 300,865,281 280,083,160 257,412,066
2022 322,747,702 301,269,670 276,362,852
2023 340,714,484 318,530,822 291,490,594
2024 356,234,529 334,221,630 305,406,923
2025 371,344,908 347,869,028 317,341,374
2026 385,518,003 360,600,010 328,416,224
2027 399,100,524 372,761,470 338,976,995
Figure 4. Accumulated cost movement of scenario 1.
Figure 6. Accumulated Cost Movement of Scenario 3.
able 4. Accumulated cost movement against base scenario. T
Accumulated Against Against base
cost
Figure 5. Accumulated Cost Movement of Scenario 2.
(KRW 1000) base scenario scenario (%)
Scenario 1399,100,523,729- -
Scenario 2372,761,470,217-26 ,339,053,512 -6,60 %
Scenario 3338,97 6,995,496- 60,123,528,233 -15.06 %
ith its low greenhouse emission, could become a reli-
isticated out-
co
6. Acknowledgements
rted by Hong-ik University
REFERENCES
[1] Korea EnergyInternational LNG
a Generation Expansion Plan-
Plan-
w
able basis source of generation and contribute to supply-
ing electricity at a lower cost if supported by better tech-
nologies to reduce construction cost.
For future studies, we believe more soph
mes could be obtained if the fuel cost, which was set at
a single amount throughout the whole involved per iod in
this study, is separated for each year. Other environ-
mental policies including ETS (Emission Trading System)
- another plan to reduce greenhouse gas emission - may
also be employed in further studies.
This research has been suppo
Industry-Academia Cooperation Foundation funded by
WP (Korea Westernpower co., Ltd)
Economics Institute, “
price trends and future prospects and implications,” 2012
[2] K. J. Kim, “Shale Gas Developments and ramifications,”
The 29th Energy forum, The National Academy of Engi-
neering of Korea, 2012.
[3] Y. C. Kim, “Multi-Criteri
ning with Global Environmental Considerations,” Korea
advanced institute of science and technology, 1993.
[4] Y. C. Kim, “Principle of the Generation Expansion
Copyright © 2013 SciRes. EPE